Phonation is primarily the result of aerodynamic forces acting on the inherently elastic tissue of the vocal folds, setting them into vibration and creating acoustic energy which we call “voice.” The characteristics of this vibration (e.g., the frequency of vibration) may be modified by muscular forces which influence the effective mass and tension of the vibrating folds. We are constantly modifying the aerodynamic and muscular forces underlying voice production to result in the wide variations of pitch and loudness produced in typical speech or in other aspects of voice function such as singing.
When laryngeal structure or physiology is impaired, the result may be a negative impact on laryngeal function, the efficiency of phonation, communication effectiveness, and subsequent perceptual characteristics of voice quality. The negative impacts on phonation and voice quality are perceptually labeled as dysphonia, which many speech–language pathologists will encounter during clinical practice. The purpose of this chapter is to provide an overview of the anatomy and physiology underlying normal, healthy phonation as a foundation to facilitate advanced understanding of impairments that result in dysphonia and their assessment, diagnosis, and treatment, which subsequent chapters will cover.
1.3 Evolution and Biological Roles of the Larynx
Voice production is considered an “overlaid” or nonbiological function of the larynx, taking a backseat to respiration, airway protection, and the generation of lung pressure to fixate the thorax during physical activity. According to Hirose, the larynx evolved as a simple muscular sphincter atop the primitive lung that would protect against entry of water or food. 1 This sphincter evolved into a more complicated valve capable of abduction (separation) or adduction (combination) at various levels within the larynx. Eventually, in mammals this protective structure also became used as a type of flutter valve that would vibrate during the controlled expiration.
During passive and active respiration, the glottis (the space between the two vocal folds) acts as an air valve. At rest, the two vocal folds lie in an abducted (away from midline) position creating an open glottis and a continuous passageway from the lungs to the oral cavity. During inspiratory cycles, the glottis will widen and then return to a resting open position during expiration. Deep, forceful inhalations will be accompanied by an even wider glottis. The vocal folds can be adjusted to an adducted (toward midline) position, so that the glottis will close forcefully to allow for the buildup of subglottal air pressure, which is required for coughing and clearing material that inadvertently falls into or irritates the larynx, trachea, or lungs. By closing the glottis in this manner, an individual can also fixate the thorax to direct muscular effort to the limbs for lifting and exercise. In a similar manner, glottal closure allows for the generation of abdominal pressures for bodily functions such as defecation.
Phonation for speech is an intentional (voluntary) behavior, but a number of involuntary reflexes mediated by the nervous system can override phonation, interrupting ongoing voice production (e.g., causing a voice break) or preventing a speaker from initiating phonation (e.g., think about food/liquid “going down the wrong pipe” when swallowing—you will experience strong laryngeal closure and/or cough, but not be able to produce voice until the stimulus is cleared). Peripheral sensory receptors located throughout the laryngeal surfaces can elicit adductor responses and cough subsequent to noxious stimuli. Central nervous system (CNS) pattern generators also mediate tonic and phasic activity in the 10th cranial nerve, called the vagus nerve, during respiration and swallowing.
The laryngeal adductor reflex is a response triggered by activation of the sensory division of the vagus nerve, which elicits activity within a brainstem nucleus called the solitary tract nucleus (STN—a.k.a., nucleus tractus solitaries; ▶ Fig. 1.1) in the medulla. The STN forms a reflex loop with the motor nucleus of the vagus nerve, the nucleus ambiguus (NA). Upon activation, the STN relays excitatory signals to the NA which then stimulates activity in the laryngeal adductor muscles via firing of alpha motor neurons. This reflex serves to forcefully close the glottis to protect the lower airway. At its most extreme, the laryngeal adductor reflex can present as laryngospasm, which is a prolonged tonic contraction of the laryngeal adductor muscles.
Fig. 1.1 Locations of sensory and motor nuclei of the vagus nerve in the brainstem.
(From LaPointe L. Atlas of Neuroanatomy for Communication Science and Disorders, 1st ed. New York: Thieme Publishers; 2011.)
The production of cough can be under voluntary or involuntary control by the nervous system. Involuntary cough is triggered by stimulation of the laryngeal sensory receptors and typically serves to eliminate foreign particles or adverse sensations throughout the larynx or in the subglottic spaces. 2 The physiology of cough can be grouped into three phases: an inspiratory phase involving recruitment of the inspiratory muscles (diaphragm and intercostals), a compressive phase involving recruitment of the expiratory muscles along with strong medial compression at the glottis via recruitment of the laryngeal adductors (and subsequent buildup of subglottal pressure), and an expulsive phase via recruitment of additional activity in the abdominal muscles resulting in high pressure air pulses being sent through the glottis. 2 The pressure pulses flowing through the glottis help to clear the airway but also result in phonation, producing the familiar perception of a cough sound. Conscious suppression of cough is possible through cortical mechanisms; however, the neural circuitry which allows for this voluntary override of the cough reflex is not well understood. 3 Voice therapy focusing on conscious control and patterning of the respiratory muscles along with semiocclusion (narrowing) in the oral cavity during exhalation are used as a behavioral voice therapy modality to treat conditions of chronic cough. 4
It is clear that the basic biological functions of the larynx generally have precedence over the overlaid development of behavioral voice function. In fact, the body’s need to breathe or protect the trachea and lungs can interrupt phonation, which many readers of this book will have experienced when trying to speak while swallowing or after an exhausting physical exercise.
1.4 Respiratory Function
Phonation is built upon a foundation of respiration. Respiratory drive provides the power source for phonation. Speakers breathe during speech using a combination of diaphragmatic and thoracic muscular activity. Primary use of the diaphragm (▶ Fig. 1.2) for speech breathing is considered the most efficient method as its contraction is not resisted by bone, whereas the thoracic muscles must expand the rib cage to influence lung volume. During passive rest breathing, brainstem pattern generators control respiratory cycles at an unconscious level. However, for speech, pyramidal (voluntary) pathways in the CNS engage the lower motor neurons (LMN) of specific spinal nerves that form the phrenic nerve (nerves C3, C4, and C5) which innervates the diaphragm. Spinal intercostal nerves also leave the spinal cord at the thoracic level to innervate the internal and external intercostal muscles (▶ Fig. 1.3). Diaphragmatic contraction moves the lungs inferiorly, while contraction of the external intercostal muscles moves the lungs horizontally and superiorly. This has the effect of increasing lung volume and lowering air pressure within the lungs, causing air to flow in for inspiration.
Fig. 1.2 The diaphragm.
(From Baker E. Anatomy for Dental Medicine, 2nd ed. New York: Thieme Medical Publishers; 2016.)
Fig. 1.3 The intercostal muscles.
(From Baker E. Anatomy for Dental Medicine, 2nd ed. New York: Thieme Medical Publishers; 2016.)
Exhalation during passive breathing is accomplished by rapid relaxation of the diaphragm and/or intercostal muscles. For speech, the air must be efficiently controlled. This is accomplished through two muscular checking actions: (1) relaxation of the diaphragm and external intercostals in concert with (2) increasing activation in the abdominal and internal intercostal muscles. The abdominal muscles are antagonistic to the diaphragm, while the internal intercostals are antagonistic to the external intercostals. These checking actions help control the outflow of air from the lungs to support speech production. Exhalation is also controlled at the level of the vocal folds, which act to valve the upward flowing air. Fully adducted vocal folds will completely seal the glottis, which will cause an increase in subglottal air pressure as exhalation from the lower lungs continues.
1.4.1 Nervous System Regulation of Respiration
The primary purpose of respiration is life sustenance, with respiratory support for speech being a secondary overlaid function. Nervous system pathways and regions that modulate the activity in respiratory muscles will vary depending on whether respiration is being controlled volitionally (e.g., during speech, holding breath, and blowing) or at an unconscious involuntary level (e.g., quiet breathing while reading, watching television, and sleeping). Control from involuntary pathways will override conscious voluntary control whenever the body detects an urgent need for different blood chemical levels (e.g., oxygen).
Conscious control of the respiratory cycle (inhalation/exhalation) involves cortical motor regions which include the bilateral pyramidal pathways. Pyramidal neurons, the majority of which originate in the primary motor cortex (precentral gyrus) of the frontal lobe, are responsible for executing motor programs for volitional, planned movement including respiratory activity during speech production. These neurons will travel a direct route to synapse on LMNs in the brainstem and spinal cord. Target LMNs in the spinal cord include those of the intercostal and phrenic nerve, which travel into the periphery to innervate the diaphragm, intercostal, and abdominal muscles.
The brainstem reticular formation contains neurons which control the involuntary patterns of respiration (▶ Fig. 1.4). Located throughout the pons and medulla, the regions of reticular formation related to involuntary respiratory patterns can be organized into four areas, two in the pons (pneumotaxic and apneustic centers) and two in the medulla (ventral respiratory group [VRG] and dorsal respiratory group [DRG]). The medullary DRG acts as the pacemaker for involuntary/passive respiration. These neurons also drive activity in the VRG. The DRG needs stimulation to modulate respiratory activity—this stimulation arrives in the form of sensory information provided by peripheral sensory nerves via input from the lungs and cardiovascular system (mechanoreceptors and chemoreceptors). This peripheral stimulation also influences the apneustic center. When neurons of the DRG depolarize and fire, they send excitatory signals to the phrenic and intercostal nerves which innervate the diaphragm and external intercostal muscles, respectively. When ventilation demands are high, such as during physical exercise, the DRG will facilitate activity in neurons of the VRG, which then recruit additional motor neuron pools to further activate the muscles of inspiration and expiration.
Fig. 1.4 Brainstem respiratory centers responsible for involuntary respiratory patterns.
(From LaPointe L. Atlas of Neuroanatomy for Communication Science and Disorders, 1st ed. New York: Thieme Publishers; 2011.)
The pontine pneumotaxic and apneustic centers can be thought of as “fine-tuning” the activity of the DRG. The apneustic center provides stimulation to the DRG to facilitate and prolong inspiration (e.g., when out of breath and needing to take deep, prolonged inhalations). The pneumotaxic center acts as an “off-switch” for inspiration. Neuronal activity in the pneumotaxic center causes termination of inspiration by inhibiting activity of the DRG. This in turn causes higher respiratory frequency (e.g., breathing faster) and reduced tidal volumes.
Activity of the brainstem respiratory pattern generator results in a resting respiratory rate of 12 to 16 breaths per minute. Inspiration usually lasts approximately 2 seconds, and expiration lasts about 3 seconds. The normal inspiratory rate and rhythm is called eupnea, and difficult respiration is termed dyspnea. Respiratory rate and patterns can be modified when respiration is brought to a conscious level by voluntary acts (e.g., speech) and during complex involuntary reflexes such as sneezing, coughing, and vomiting. In addition, respiratory activity can be affected by conditions such as emotional state via input from the limbic system, or temperature via the hypothalamus.
The vagus nerve is also influenced by brainstem respiratory pattern generators and voluntary cortical pathways, and is active during voluntary and involuntary cycles of respiration. Laryngeal muscles controlled by the vagus are activated by brainstem respiratory centers during inspiration. The abduction–relaxation phasic action of the glottis resulting from this muscular activity is visible on laryngeal endoscopy when a patient is breathing at rest. During expiration, laryngeal muscles are also activated. Voluntary cortical control of laryngeal muscles during inspiration is present for such tasks as respiratory support for speech and deep inhalations, both of which require increased activity in laryngeal muscles via the vagus nerve to widen the glottis.
1.5 Laryngeal Framework
The larynx is located in the midline of the anterior neck (▶ Fig. 1.5), suspended from the hyoid bone above and attached to the trachea below via connective tissue (membranes, ligaments, and muscles). Removal of the connective tissue within the larynx would reveal a framework of six named cartilages (some are paired and some are unpaired). Five of these cartilages articulate with at least one other laryngeal cartilage, while the remaining one is suspended in connective tissue. Although technically not part of the laryngeal framework, the influence of the hyoid bone on laryngeal position must be emphasized. It is generally accepted that vertical hyoid position, which is determined by the degree of activation in muscles which attach to it, can have significant influence on the physiology of phonation.
Fig. 1.5 Anterior view. The larynx is located in the midline of the anterior neck. Laryngeal structures at rest are positioned at characteristic vertebral levels in adult males: hyoid bone: C3; thyroid cartilage (superior border): C4; cricotracheal membrane: C6–C7. These structures are a half vertebra higher in females and children.
(From Baker E. Anatomy for Dental Medicine, 2nd ed. New York: Thieme Medical Publishers; 2016.)
1.5.1 Hyoid Bone
The hyoid is a horseshoe-shaped bone in the anterior midline of the neck positioned at the level of the third cervical vertebra. The broad central portion of the hyoid is referred to as the body, to which are fused two lateral (left and right) and superior bony projections called the “greater horns” (or cornu, which is Latin for “horn”) and “lesser horns” of the hyoid, respectively (▶ Fig. 1.6). The body, greater horns, and lesser horns serve as points of attachment for muscles and other connective tissue. The hyoid serves as an origin or insertion point for muscles that move the jaw, tongue, and larynx. Laryngeal muscles that connect to the hyoid can have the effect of elevating the hyoid bone in a superior and anterior direction, bringing the hyoid bone in closer approximation to the laryngeal framework, or depressing the larynx in an inferior direction.
Fig. 1.6 Laryngeal framework. Oblique left anterolateral view.
(From Baker E. Anatomy for Dental Medicine, 2nd ed. New York: Thieme Medical Publishers; 2016.)
1.5.2 Thyroid Cartilage
The singular (unpaired) thyroid cartilage is located in the anterior midline of the neck and forms a large portion of the anterior laryngeal border. The thyroid cartilage is shaped like a shield (▶ Fig. 1.6), formed by two broad plates (laminae) of hyaline cartilage which fuse at the midline. Numerous muscles attach to the thyroid lamina, influencing its vertical position. Superior and inferior thyroid horns (cornua) project from the posterior borders of the thyroid lamina and serve as point of attachment for connective tissue. At the apex of the thyroid midline, the fusion is incomplete creating the thyroid notch, which can be located by digital palpation in most adults. Immediately inferior to the notch is a raised portion of cartilage known as the thyroid prominence (a.k.a. “Adam’s Apple”), which can be very prominent in males. The vocal fold tissue is attached to the thyroid cartilage on the internal surface of the thyroid lamina just below the thyroid notch at a point called the anterior commissure. The superior borders of the thyroid are connected to the hyoid bone via a sheet of fibrous tissue called the thyrohyoid membrane. The middle and lateral margins of this membrane are thickened and referred to as the median and lateral thyrohyoid ligaments, respectively.
1.5.3 Cricoid Cartilage
The singular cricoid cartilage (▶ Fig. 1.6; ▶ Fig. 1.7) forms the inferior rim of the larynx. It is attached to the trachea inferiorly via the cricotracheal ligament. The cricoid is shaped like a ring with a thin anterior rim of hyaline cartilage which arches posterolateral (backward and to the side) to form a broad posterior lamina. A raised smooth surface is located on each lateral arch (left and right) forming articular facets where the cricoid communicates with the inferior horns of the thyroid, forming the cricothyroid (CT) joint. Additional articular facets are located on the superior surface of the posterior lamina where the cricoid communicates with the arytenoid cartilages, forming the cricoarytenoid joint.
Fig. 1.7 Cricoid cartilage. (a) Posterior view. (b) Anterior view. (c) Left lateral view.
(From Baker E. Anatomy for Dental Medicine, 2nd ed. New York: Thieme Medical Publishers; 2016.)
1.5.4 Arytenoid Cartilages
The paired arytenoids (▶ Fig. 1.8a) are pyramid-shaped hyaline cartilages situated atop the cricoid at the cricoarytenoid joint. At the arytenoid base are two projections, one posterolateral and one anterior, called the muscular process and vocal process, respectively. The muscular process serves as a point of attachment for muscles which will move the arytenoid to adjust the position of the vocal folds. The vocal process serves as the posterior attachment for the vocal fold tissues. The body and apex of the arytenoids serve as attachment points for laryngeal muscles and connective tissue. Muscular contraction can move the arytenoids such that during adduction, they rotate medially to approximate each other with a slightly inferior tilt, while also sliding horizontally along the cricoarytenoid joint.
Fig. 1.8 Arytenoid and corniculate cartilages. (a) Right lateral view. (b) Left lateral (medial) view. (c) Posterior view. (d) Superior view. (e) Corniculate and cuneiform cartilages. Endoscopic view of the larynx showing the pyramid-shaped arytenoids capped by the pointed corniculate cartilages, with the cuneiform tubercles (the two rounded eminences) visible on the superior surface of the aryepiglottic folds. Corniculate (left arrow) and cuneiform (right arrow) cartilages.
(a–d from Baker E. Anatomy for Dental Medicine, 2nd ed. New York: Thieme Medical Publishers; 2016.)
1.5.5 Epiglottis
The epiglottis is a leaf-shaped elastic cartilage attached to the inner medial surface of the thyroid just below the thyroid notch via the thyroepiglottic ligament. At its midpoint, the epiglottis is also attached to the hyoid bone via the hyoepiglottic ligament. The epiglottis (▶ Fig. 1.6; ▶ Fig. 1.9) may have a role in phonation by generating pressures above the vocal folds and also influencing resonance of sound generated during phonation. It plays a critical role in swallowing, during which inversion of the epiglottis occurs to cover the larynx to protect the lower airway.
Fig. 1.9 Posterior view of laryngeal cartilages and ligaments. Arrows indicate movement in the various joints.
(From Baker E. Anatomy for Dental Medicine, 2nd ed. New York: Thieme Medical Publishers; 2016.)
1.5.6 Corniculate and Cuneiform Cartilages
The corniculate and cuneiform cartilages provide structural integrity for the larynx but have debated influence on phonation. The paired corniculate cartilages (▶ Fig. 1.8a; ▶ Fig. 1.8b) are small horn-shaped structures situated atop the apex of the arytenoids. The paired cuneiform cartilages are embedded in a thickened rim of connective tissue called the aryepiglottic folds. Both cartilages are considered “vestigial,” meaning they have no known function other than possible structural support (▶ Table 1.1).
Cartilage | Paired/Unpaired | Shape | Functions |
Thyroid | Unpaired | Plow/Shield |
|
Cricoid | Unpaired | Ring |
|
Arytenoid | Paired | Pyramid |
|
Epiglottis | Unpaired | Leaf |
|
Corniculate | Paired | Cone |
|
Cuneiform | Paired | Wedge |
|
1.6 Connective Tissues
Additional membranes and ligaments line and connect the framework of the larynx and, with exception of the vocal folds, they are lined by a cellular layer of pseudostratified, ciliated, columnar epithelium (“respiratory epithelium”). Respiratory epithelium contains microscopic cilia which move to create a superior flow of secretions that help lubricate the larynx and remove debris (e.g., dust, and dead cells). Below the level of the vocal folds, a dense band of collagenous tissue called the conus elasticus connects the cricoid, thyroid, and arytenoid cartilages with each other (▶ Fig. 1.10; ▶ Fig. 1.11). The medial and lateral portions of this membrane are thickened and known as the median and lateral cricothyroid ligaments, respectively (▶ Fig. 1.10). Above the level of the vocal folds, another dense band of connective tissue called the quadrangular membrane (▶ Fig. 1.10; ▶ Fig. 1.11) connects the arytenoids, epiglottis, and thyroid cartilages. The superior rim of this membrane is thickened and called the aryepiglottic folds, forming the rim of the entry into the larynx known as the laryngeal vestibule. The most inferior portion of the quadrangular membrane is also thickened on each side (left and right), coursing horizontally where it connects the posterior arytenoids to the anterior thyroid cartilage, forming the ventricular ligaments. These ligaments along with the surface epithelium and underlying musculature are known as the ventricular folds, vestibular folds, or false vocal folds (all three names are synonymous; ▶ Fig. 1.10; ▶ Fig. 1.11). A deep recess exists in the space between the ventricular folds and (true) vocal folds, called the laryngeal ventricle (▶ Fig. 1.11), which contains mucous glands that secrete fluids and lubricate the vocal fold tissue.
Fig. 1.10 Laryngeal connective tissue and spaces. (a) Posterior view with pharynx and esophagus cut along the midline and spread open. (b) Left lateral view of the midsagittal section. (c) Posterior view with the laryngeal levels. Regions shaded in pink are connected by the conus elasticus; regions covered in purple are covered by the quadrangular membrane.
(From Baker E. Anatomy for Dental Medicine, 2nd ed. New York: Thieme Medical Publishers; 2016.)
Fig. 1.11 Vocal folds, glottis, and ventricular (vestibular or false) folds.
(From Baker E. Anatomy for Dental Medicine, 2nd ed. New York: Thieme Medical Publishers; 2016.)
The paired vocal folds act as a dividing line, such that the laryngeal framework below the vocal folds is lined by the conus elasticus, while the framework above the vocal folds is lined by the quadrangular membrane. The space between the two vocal folds is known as the glottis (▶ Fig. 1.11), which can be open (abducted) or closed (adducted) depending on vocal fold position. As stated earlier, the vocal folds are attached posteriorly to the vocal process of the arytenoids and attach anteriorly at the anterior commissure of the thyroid. The vocal folds are another form of connective tissue consisting of a layered structure stratified by varying degrees of proteomic concentrations.
1.7 Vocal Fold Histology
The outermost (superficial) layer of the vocal folds is composed of nonkeratinized stratified squamous epithelium (▶ Fig. 1.12). The vocal fold surface is hydrated by transepithelial water fluxes moving to the surface from within, and mucus secretions from glands within the vocal folds and laryngeal ventricles. 5 Immediately below the epithelium is a basement membrane zone containing numerous collagen fibers which connect the epithelium to the layer immediately below the superficial layer of the lamina propria (SLLP). The SLLP, also known as Reinke’s space, is composed mainly of amorphous interstitial (between the cells) proteins along with small concentrations of elastin and collagen. The SLLP is highly viscous and characterized by a consistency likened to soft gelatin. Together the vocal fold epithelium and the SLLP move as a functional unit called the vocal fold cover.
Fig. 1.12 Vocal fold histologic section showing structural layers.
(From Kendall K, Leonard R. Laryngeal Evaluation, 1st ed. New York: Thieme Publishers; 2010.)
Deep to the SLLP, the concentration of elastin protein becomes greater, forming the intermediate layer of the lamina propria (ILLP). Deep to the ILLP, the ratio of collagen protein concentration becomes greatest, forming the deep layer of the lamina propria (DLLP). Together the ILLP and the DLLP form the vocal ligament, which connects the arytenoid cartilages to the thyroid and supports vibrational stresses during phonation. Deep to the DLLP is the vocalis muscle (see section “Laryngeal Muscles,” below), which is considered the body of the vocal fold. The transition from the most superficial layer to the deepest layer of the vocal folds is characterized by an increasing stiffness gradient, with the lax and mobile cover being able to oscillate over a stiff body, with the cover connected to the body by the vocal ligament. The health of the epithelial cells of the vocal fold cover, the proteomic concentrations in the different layers of the lamina propria, and the contractile properties of the vocalis muscle influence the physiology of vocal fold vibration. An abnormality in any of these layers can impair phonation and disturb the resulting acoustic sound energy, resulting in the perception of dysphonia (▶ Table 1.2).
Name | Attachments/Functions |
Cricotracheal ligament | Inferior rim of cricoid to first tracheal ring; connects inferior larynx to trachea |
Cricothyroid ligament (a.k.a. conus elasticus) | Connects laryngeal structures below vocal folds |
Median cricothyroid ligament | Thickened medial division connecting cricoid arch to middle thyroid lamina at inferior border |
Lateral cricothyroid ligament | Thinner lateral division connecting cricoid to lateral thyroid lamina at inferior border |
Vocal ligament | From anterior commissure of thyroid to vocal process of arytenoid. Links vocal fold cover to vocal fold body |
Cricoarytenoid ligament | Midline of posterior cricoid lamina to base of arytenoid. Stabilizes the cricoarytenoid joint |
Quadrangular membrane | Broad sheet of membrane coursing upward from arytenoids to epiglottis. Covers and connects laryngeal structures above the level of vocal folds |
Ventricular ligament (a.k.a. vestibular ligament) | From thyroid cartilage above the anterior commissure to anterior surface of arytenoid cartilage above the vocal process. Adducts via muscle contraction to prevent food/liquid from entering lower airway during swallowing |
Aryepiglottic folds | Thickened superior borders of the quadrangular membrane. Forms the laryngeal vestibule and houses the cuneiform cartilages |
Thyrohyoid membrane | From superior borders of thyroid to inferior border of hyoid bone. Connects larynx to the hyoid bone—the larynx is considered to be suspended from the hyoid via this membrane |
Median thyrohyoid ligament | Thickened medial division connecting thyroid lamina to body and cornu of hyoid bone |
Lateral thyrohyoid ligament | Thickened lateral division connecting superior thyroid cornu to greater cornu of hyoid bone |
Thyroepiglottic ligament | From thyroid just below the notch to the petiolus of epiglottis. Connects epiglottis to thyroid cartilage. |
Hyoepiglottic Ligament | From hyoid bone body to middle portion of epiglottis. Connects epiglottis to hyoid bone—when hyoid bone elevates during swallowing, the connection via this ligament results in epiglottic inversion, which covers the laryngeal vestibule |
1.8 Laryngeal Muscles
1.8.1 Extrinsic Laryngeal Muscles
Extrinsic laryngeal muscles are characterized by an origin external to the larynx with an insertion on a laryngeal cartilage or on the hyoid bone. Some of these muscles originate inferior to the larynx and some superior, and their contraction will either depress the larynx or elevate the larynx, respectively. Collectively, the extrinsic muscles are referred to as the laryngeal strap muscles, as they act to “strap” the larynx in place within the vertical plane. As such, the extrinsic laryngeal muscles position the larynx in the vertical plane along the midline of the neck. Greater activation in the laryngeal depressors will lower the larynx, while greater activation in the laryngeal elevators will raise the larynx (▶ Fig. 1.13). While these muscles do not have a primary effect on the vibratory characteristics of the vocal folds, inefficient/ineffective use of the extrinsic musculature may “leak over” to result in inefficient use of the intrinsic musculature.
Fig. 1.13 Laryngeal elevators and depressors. (a) Left lateral view. (b) Anterior view. (c) Posterosuperior view. Due to their point of origin, laryngeal elevators are also referred to as the suprahyoid muscles, while the laryngeal depressors are also referred to as the infrahyoid muscles.
(From Baker E. Anatomy for Dental Medicine, 2nd ed. New York: Thieme Medical Publishers; 2016.)
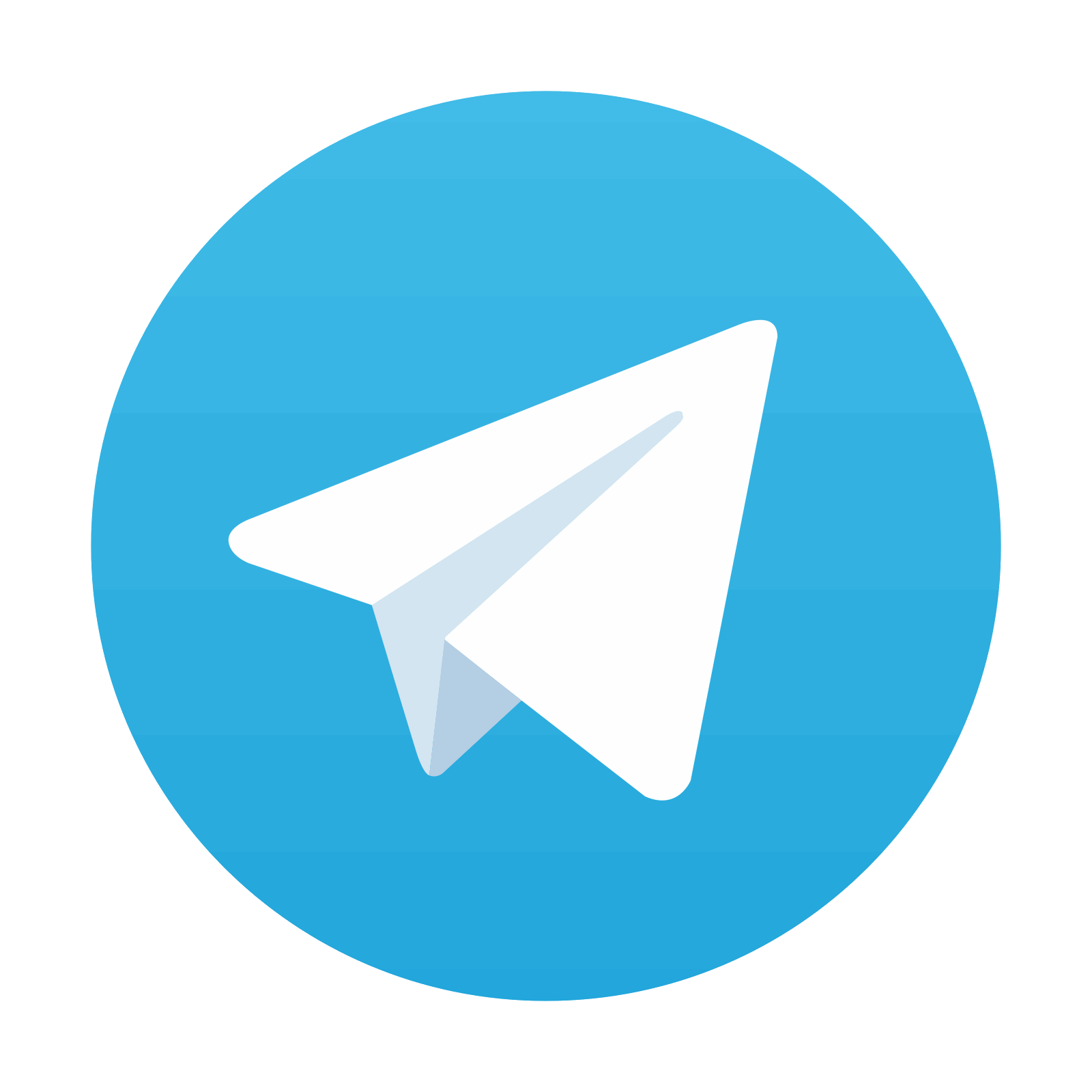
Stay updated, free articles. Join our Telegram channel

Full access? Get Clinical Tree
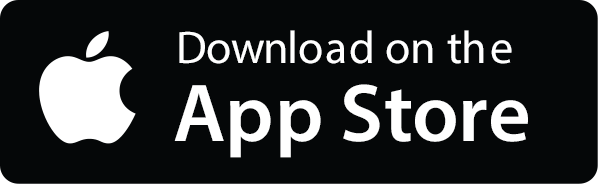
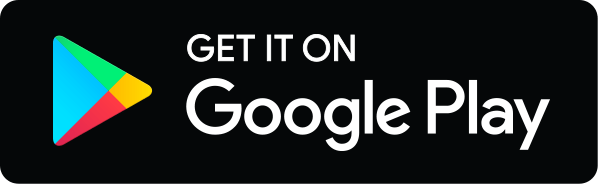