2 Retinal and Retinal Pigment Epithelial Physiology
2.1 Introduction
Many retinal disorders have a selective effect on particular cells or metabolic systems within the retina and retinal pigment epithelium (RPE). Effective management of these disorders, which include conditions as diverse as retinitis pigmentosa (RP), central serous chorioretinopathy (CSC), and congenital color blindness, requires an understanding of retinal physiology and of the interaction between the retina and RPE. The physiology of the retina cannot be separated from that of the RPE, especially in a clinical context, because the RPE actively supports and facilitates retinal function and is also a frequent site of damage in disorders of the ocular fundus. 1
2.2 Retinal Pigment Epithelium
Although the RPE is derived embryologically from the same neural tube tissue as the neurosensory retina, the RPE cells differentiate into a monolayer epithelium that behaves functionally more like the lining of the gallbladder than like central nervous system tissue. The cells are linked by tight junctions and show anatomic and physiologic membrane specializations on the apical and basal surfaces (Fig. 2-1). These enable the RPE to control water and nutrient access to the subretinal space, which is critical to the viability of the photoreceptors. Major functions of the RPE are outlined in the following text box.

Selected Functions of the Retinal Pigment Epithelium
Pigment functions
Light adaptation and screening
Detoxification and binding
Lipofuscin accumulation
Antigenic properties
Environment and metabolic control
Blood–retinal barrier
Transport of nutrients and ions
Dehydration of subretinal space
Synthesis of enzymes, growth factors, and pigments
Interaction with endocrine, vascular, and proliferative factors
Visual pigment cycle
Capture and storage of vitamin A
Isomerization of all-trans to 11-cis vitamin A
Retinal adhesion and interphotoreceptor matrix (IPM)
Matrix domains surrounding rods and cones
Metabolic control of adhesiveness
Photoreceptor phagocytosis and aging
Phagocytosis of outer segment tips
Digestion and recycling of membrane material
Aging effects: lipofuscin, drusen
Deposits and alterations in Bruch’s membrane
Electrical activity
Response to light-induced ionic changes: c-wave, fast oscillation
Response to light-induced chemical signals: electro-oculogram (EOG)
Nonphotic responses to chemical agents
Repair and reactivity
Repair and regeneration
Immunologic interactions
Scarring and pigment migration
Role in modulating fibrovascular proliferation
2.2.1 Pigments and Optical Functions of the Retinal Pigment Epithelium
The RPE is so named because of its content of melanin, a large polymeric molecule synthesized in cytoplasmic granules called melanosomes. The RPE is the first tissue in the body to become pigmented, and some degree of melanogenesis continues throughout life. However, in older eyes, many melanin granules fuse with lysosomes and break down, and the elderly fundus typically appears rather depigmented. It has long been assumed that a major function of melanin was the absorption of stray light, to minimize scatter within the eye. This is understandably true in animals such as the frog, in which long pigment-containing processes extend up between the photoreceptors. However, the RPE processes around rods are rather short in humans, and in general the RPE is less pigmented than the choroid (most racial differences in fundus appearance reflect pigmentation of the choroid rather than of the RPE). There is no difference in visual acuity between blond and heavily pigmented fundi. The loss of acuity in albinism is a result of poor foveal cone organization rather than of light scatter.
Melanin may also serve a protective function in the eye, as a free radical scavenger and as an agent that can bind toxins. This latter role is controversial, however, because it is not clear whether the binding of certain retinotoxic drugs, such as chloroquine and thioridazine, protects the retina by removing the toxic agents from the cytoplasm or enhances their toxicity by concentrating the agents near the retina.
As the eye ages, there is accumulation of another RPE pigment, lipofuscin. This is an aging pigment, present throughout the nervous system, but it may have special significance within the eye. Lipofuscin in the RPE is thought to be derived from lipids of the photoreceptor outer segments and metabolic by-products of the visual pigment regeneration cycle that have been ingested and digested by the RPE through the process of outer segment renewal (see later), including membrane fragments that may have been damaged by light absorption or oxidation. A major component is N-retinylidene-N-retinyl-ethanolamine, or A2E, a toxic by-product of all-trans-retinal binding that is controlled in part by the ABCA4 (Stargardt’s disease) gene. 2 , 3 Lipofuscin can be identified even in the RPE of children, but it does not become prominent until adulthood. With aging, the RPE cells are often clogged massively with the golden autofluorescent pigment.
Controversial Points
Melanin in the RPE may have a protective function in scavenging free radicals and binding toxins. However, this latter role is controversial, as it is not clear whether binding of certain drugs, such as chloroquine and thioridazine, protects the retina or enhances toxicity by concentrating the agents near the retina.
A2E is a component of the lipofuscin-like material that accumulates in the RPE of eyes with Stargardt’s disease, fundus flavimaculatus, and Best’s disease (vitelliform dystrophy). 4 The autofluorescent pigment in these disorders clogs cells throughout the retina, even at younger ages, and not just in the macular region, where fundus pathology of these disorders is most prominent clinically. However, we still have much to learn about these disorders. For example, although a Stargardt’s disease phenotype can by caused by ABCA4 mutations, defects in the same gene can also cause cone dystrophy or even retinitis pigmentosa. Furthermore, there can be phenotypic variations within a family, and the relationship to A2E is not always clear.
2.2.2 Control of the Subretinal Space
The neurosensory retina is embryologic brain tissue, and as such it requires the same environmental protection as tissue in the brain. A blood–brain or blood–retinal barrier is provided in part by the intrinsic retinal blood vessels, which have tight junctions like cerebral vessels (inner blood–retinal barrier). However, the choroidal vessels, which provide nutrition to the outer retina, are leaky, and the other part of the blood–retinal barrier is provided by the tight junctions of the RPE (outer blood–retinal barrier). Secreted factors, such as vascular endothelial growth factor (VEGF), play an important role in fluid homeostasis in the subretinal space and maintenance of this outer blood–retinal barrier (see later). The apical and basal RPE membranes contain a variety of selective ion channels and a variety of active and facilitative transport systems that control the movement of ions and water and the transport of metabolites, such as glucose and amino acids. 5 The amino acid taurine, for example, is essential for photoreceptor function and is concentrated by the RPE; D-glucose but not L-glucose is transported into the subretinal space. There are different channels and transporters on the apical and basal surfaces, such as an electrogenic sodium–potassium pump on the apical membrane and a chloride–bicarbonate exchange transporter on the basal membrane. The asymmetry between the apical and basal membranes accounts for the development of a voltage (called the standing potential) across the RPE, and it also results in a net movement of ions away from the subretinal space that drives the transport of water.
Special Considerations
The blood–retinal barrier is provided by the tight junctions of the retinal blood vessels (inner barrier) and the tight junctions of the RPE (outer barrier). For proper protection and function of the neurosensory retina, the barrier needs to be intact at both levels.
The RPE active transport mechanisms for water are very powerful. If a large retinal detachment is buckled without drainage, fluid can clear within 24 hours. The RPE can remove water from the subretinal space against a substantial gradient of hydrostatic or osmotic pressure, even when the subretinal fluid contains protein. 6 It is important to recognize that it is the presence of tight junctions between the RPE cells that necessitates this active transport. Passive transport mechanisms, such as intraocular pressure against the retina and osmotic pressure from the choroid, also tend to drive fluid out of the subretinal space—but water movement is normally restricted by the RPE barrier. When the RPE barrier is damaged, fluid actually leaves the subretinal space faster than under normal conditions. In other words, a normal eye will not accumulate fluid in the subretinal space just because the RPE barrier is disrupted.
These observations have relevance for clinical disorders such as serous detachments. They show that the presence of a defect in the RPE is not by itself sufficient to cause serous detachment, even though it may be necessary. 7 There must be a pressure head within the choroid, such as a neovascular membrane or an area of ischemic or inflammatory injury, to drive fluid in the retinal direction. This explains the fluid that accumulates over areas of inflammation and RPE damage in Harada’s disease or over regions of choroidal ischemia in accelerated hypertension or preeclampsia, or the local fluid that accumulates over choroidal neovascularization in age-related macular degeneration. However, this does not explain why fluid spreads far beyond a small focal leak in disorders such as idiopathic CSC (Fig. 2-2 ), as one would expect the RPE to remove such fluid rapidly. These conditions probably involve a diffuse impairment of fluid transport across larger regions of RPE, which prevents rapid absorption. This could result from RPE disease, but may more often be a result of choroidal disease. For example, indocyanine green angiography of eyes with CSC typically shows broad areas of choroidal hyperperfusion and hyperpermeability, and high-resolution optical coherence tomography shows choroidal thickening in the central macula. The leak itself may be something of an epiphenomenon—but when a leak develops in a susceptible eye, fluid can accumulate and the disease becomes symptomatic (see ¦Chapter 16¦).

2.2.3 Visual Pigment Regeneration
Another critical metabolic interaction between the retina and RPE is the visual pigment cycle. The RPE serves to capture vitamin A from the bloodstream, and it stores esterified vitamin A within its cytoplasm. Specific binding proteins (e.g., interphotoreceptor retinoid-binding protein, IRBP) serve to transport vitamin A across the subretinal space to be incorporated into rhodopsin in the photoreceptors. After rhodopsin is activated by light, the desensitized (all-trans) vitamin A is transported back to the RPE to be stored and isomerized to the 11-cis conformation (Fig. 2-3). Many of the enzymes involved in this process have been found to cause human disease when missing or abnormal. 8

This cycle of rhodopsin regeneration can take upward of a half-hour in a normal eye and is a basis of the clinical test of dark adaptometry. After an extensive exposure to bright light, the cones recover to maximum sensitivity in 6 to 8 minutes in the dark, whereas the rods require close to 30 minutes. The significance of this regenerative process is apparent to all of us as the time it takes to adjust to the darkness inside a movie theater or on a moonlight walk.
The RDH5 gene in RPE causes a disorder called fundus albipunctatus, 9 , 10 which shows white dots throughout the posterior fundus associated with a curious form of night blindness. Affected individuals cannot find their seats going into a movie theater but may see well by the end of a double feature. The problem is not a lack of rod function but a striking delay in dark adaptation. After 4 hours in the dark, patients with fundus albipunctatus can have normal sensitivity and a normal rod electroretinography (ERG). Loss of the RPE genes LRAT or RPE65 will disrupt rod function severely and cause a form of Leber’s amaurosis (essentially an early-onset severe RP).
2.2.4 Photoreceptor Regeneration and Phagocytosis of Outer Segments
The photoreceptor outer segments are exposed to radiant energy on a regular basis, and they exist in a high-oxygen environment by proximity to the choriocapillaris. Both light absorption and oxygen facilitate the production of free radicals, which damage lipid membranes. Cells in the skin would not last a lifetime without renewal, and the same is true for the photoreceptor outer segments. Thus, every day more than 100 discs at the distal end of each photoreceptor outer segment are phagocytosed by the RPE (Fig. 2-4), and new discs are synthesized continually to replace them. 11 The cones tend to shed more vigorously at the onset of darkness and the rods at the onset of morning light, but some phagocytosis takes place continually. The phagocytosed disc material becomes encapsulated in bodies called phagosomes, which merge with lysosomes to facilitate digestion. Residual material is eventually egested across the basal RPE membrane. Some necessary fatty acids are retained and recycled back into the synthetic process. The metabolic demands of this process are impressive; each RPE cell ingests and digests upward of 4,000 discs daily. The ABCA4 protein is believed to be a transporter within the photoreceptor disc membrane involved in the recycling process of all-trans-retinal. Defects lead to accumulation of A2E (discussed earlier) within the discs and subsequently within the RPE cells that phagocytose them.

A form of RP has been identified which depends on this process. Defects in the MERTK gene leads to a failure of RPE phagocytosis, followed by degeneration of the overlying rods and cones. 12 Pathology of the phagocytic process could, in theory, be relevant to excessive lipofuscin formation or to other aspects of aging and age-related macular degeneration.
2.2.5 Retinal Adhesion and the Interphotoreceptor Matrix
The RPE contributes to the formation of the IPM and to its effectiveness as a bond between the retina and RPE. It is important to recognize that the IPM is not just a viscous “goo” but has an elaborate structure based on segregated chemical domains that contain different glycosaminoglycans and related molecules. By binding fluorescent lectins to these molecules, one can identify discrete matrix sheaths that surround the cones and rods independently and bond to both RPE and outer segment membranes.
Adhesion of the retina to the RPE is a complex physiologic function; it appears to involve several complementary systems that keep the retina firmly in place unless a positive force (such as vitreous traction) pulls it off. 13 Retinal apposition is facilitated by intraocular pressure on the retina, by the draw of choroidal osmotic pressure, and by the presence of a formed vitreous gel. The RPE microvilli also wrap tightly around the tips of the outer segments for the purpose of phagocytosis, but there are no anatomic connections between the RPE microvilli and the outer segments. The IPM forms a viscous bond between the two layers, but this fluid connection is probably of lesser importance than the structural and chemical bond that is formed by the IPM by means of specific receptors where the matrix is in contact with the outer segment and RPE membranes. For example, these matrix domains can be seen to stretch dramatically when the retina is peeled away from the RPE (Fig. 2-5), which demonstrates the firm attachment of the IPM to both the retinal and RPE surfaces. 14

The structural and bonding characteristics of the IPM are affected by its degree of hydration and its ionic content, both of which are controlled by the transport characteristics of the RPE. Thus, the strength of retinal adhesion is highly dependent on metabolic activity, which governs RPE ion and water transport. 13 For example, retinal adhesive strength drops within minutes after death or after occlusion of the ocular circulation. This metabolic dependence of retinal adhesion may explain, in part, the greater susceptibility to detachment in older eyes (or perhaps highly myopic eyes), in which the vascular supply may be less functional as a result of age (or ocular expansion).
Retinal adhesive strength can be modulated osmotically and metabolically in experimental models. Systemic osmotic agents dehydrate the subretinal space and IPM and increase retinal adhesion to a modest degree, and a similar increase in adhesive strength can be produced by acetazolamide and other carbonic anhydrase inhibitors because they increase the rate at which fluid is transported out of the subretinal space. These agents could, in theory, prove helpful in the management of detachment disorders, but to date no such applications have been demonstrated.
Pearls
The IPM plays a significant role in the normal adhesion of the neurosensory retina to the RPE. The structural and bonding characteristics of the IPM are affected by its degree of hydration and its ionic content, both of which are controlled by the RPE. Therefore, conditions that affect the metabolic activity of the RPE can, in turn, affect the strength of retinal adhesion.
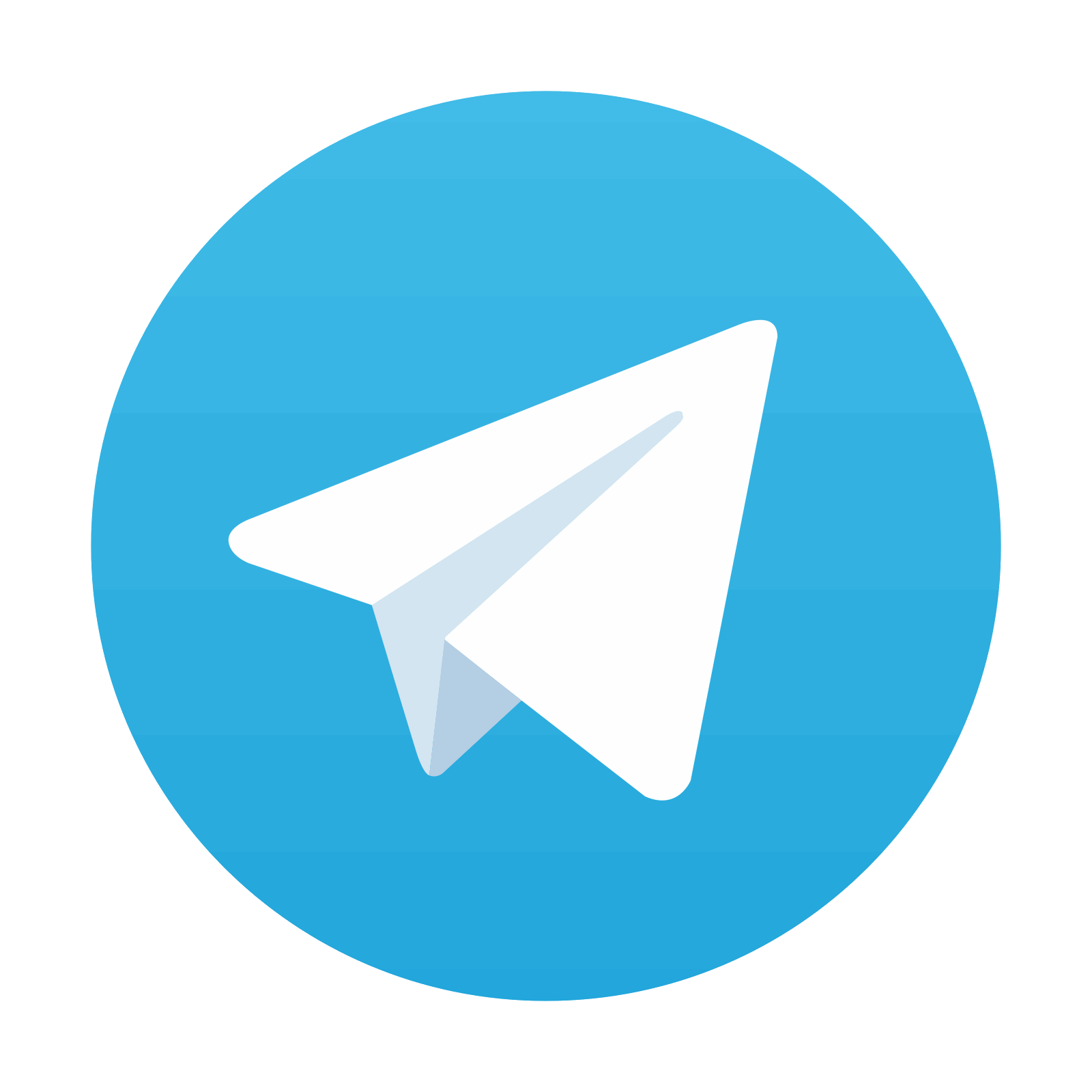
Stay updated, free articles. Join our Telegram channel

Full access? Get Clinical Tree
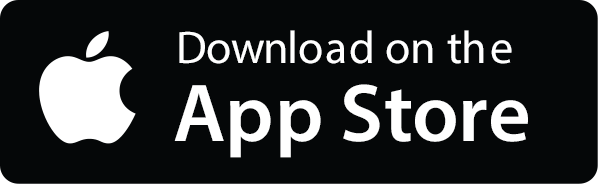
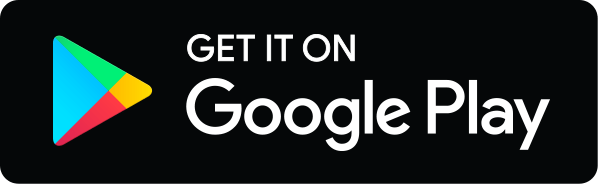
