Purpose
Postoperative endophthalmitis is a potentially sight-threatening complication of cataract surgery. However, the pathophysiological mechanisms are not completely understood. We sought to study and evaluate the intraocular environment (aqueous and vitreous humors), the capsular tissue, and the intraocular lens (IOL) surfaces of normal eyes after long-term uncomplicated cataract surgery.
Design
Experimental laboratory investigation.
Methods
We studied 69 eyes donated for transplantation that had previously undergone cataract surgery with posterior chamber IOL implantation and that had no recorded clinical history of postoperative inflammation. We assessed the intraocular environment (DNA traces and biofilm formation) by microbiological evaluation of intraocular fluids using conventional microbiology and molecular techniques, including assessment for the presence of microbes (biofilm formation) on the IOL surface by scanning electron microscopy and ultrastructural capsular remnants by transmission electron microscopy.
Results
Isolated or aggregated cocci were probable in 18.8% of IOL optic surfaces (n = 13) studied by scanning electron microscopy, suggesting the presence of bacterial biofilm. In 3 intraocular fluid samples for IOLs with biofilm, we identified 16S rDNA by polymerase chain reaction and sequencing. No microbial contamination was found in intraocular fluids by conventional microbiological methods.
Conclusions
Our data suggest the possibility of bacterial biofilm formation on the optic surface of IOLs in normal eyes after long-term uncomplicated cataract surgery even in the absence of clinical or subclinical symptoms.
Cataract removal and lens replacement with an artificial intraocular lens (IOL) is the most frequent surgery performed by ophthalmologists. The most fearsome complication of cataract surgery is postoperative endophthalmitis, which is defined as a severe inflammation of both the anterior and posterior segments of the eye secondary to an infectious agent. With incidence rates of 0.05%–0.34%, postoperative endophthalmitis is clinically classified into acute or chronic, depending on the onset after surgery. Acute endophthalmitis presents with pain; photophobia; floaters; reduced vision; an inflamed anterior segment, including a variable hypopyon; and vitritis. The predominant clinical feature of chronic or delayed-onset endophthalmitis is white plaques on the lens capsule or the IOL surface, representing collections of organisms. Endophthalmitis is associated with recurring episodes of low-grade inflammation that are initially responsive to topical steroid treatment, but that become refractive at later stages.
Coagulase-negative staphylococci, especially Staphylococcus epidermidis , and others, such as S aureus and Streptococcus spp. are currently the most common etiologic agents of acute postoperative endophthalmitis. In contrast, indolent organisms are usually responsible for chronic endophthalmitis, including Propionibacterium acnes and Corynebacterium spp. However, it is well known that microorganisms can be introduced into the eye from the patient’s external microbiota during surgery. In fact, molecular typing techniques have been used to confirm that 68%–82% of cases of postoperative endophthalmitis are caused by ocular and periocular microbiota. Reducing the number of organisms present on the ocular surface with the preoperative application of topical povidone-iodine in the conjunctival sac and periocular skin is the only measure with proven prophylactic value against endophthalmitis.
Endophthalmitis is a potentially sight-threatening infection. Detection of endophthalmitis and identification of the causative agent are therefore essential to ensure that rational treatment is given, yet routine microbiologic diagnosis relies on time-consuming classical culture techniques that offer low sensitivity; indeed, the positivity rates range from 22% to 30% in the aqueous humor and from 40% to 69% in the vitreous humor. Modern molecular techniques—such as polymerase chain reaction (PCR) combined with different post-PCR analyses—now allow for greater sensitivity and more rapid results and are particularly useful when only a small amount of material is available (eg, aqueous humor) or if the patient has previously received antibiotic treatment. In addition, molecular techniques are promising for the detection of organisms associated with chronic postoperative endophthalmitis because the samples are often small, difficult to culture, fastidious, or slow growing.
The mechanism through which postoperative endophthalmitis develops has not been completely elucidated to date. Nevertheless, it is recognized that bacterial adhesion to IOLs and intraocular tissues during implantation is probably the primary step in the pathogenesis. Then, cell-to-cell adhesion and the production of extracellular polysaccharide glycocalyx (slime) on the lens surface, followed by the formation of a confluent biofilm structure, appear to be relevant. Indeed, S epidermidis produces few virulence factors, but its success as an opportunistic pathogen is related to its ability to adhere to, and then form a mature biofilm on, the surface of different medical devices. Finally, although numerous in vitro studies have demonstrated that bacterial adhesion may be influenced by IOL biomaterials, differences in experimental conditions and protocols have meant that results differ.
Despite advances in our knowledge, little is known about the intraocular environment and behavior of the IOL surface in the long term after uncomplicated cataract surgery. Thus, we aimed to analyze the intraocular fluids (aqueous and vitreous humors), capsular tissues, and IOL surfaces of normal eyes donated for corneal transplantation after long-term uncomplicated cataract surgery. To our knowledge, this is the first time that biofilm formation has been studied in this setting on a large scale.
Methods
Eye Bank Specimens
In this study, we analyzed 69 donated eyes that had previously undergone cataract surgery and posterior chamber IOL implantation. Globes were obtained from the Eye Bank for the Treatment of Blindness (Centro de Oftalmología Barraquer, Barcelona, Spain) after obtaining consent from the family and in accordance with the Standardized Rules for Development and Applications of Tissues and Organ Transplants, as defined in Spanish law. Eyes were donated for corneal transplantation, but were discarded because they did not meet the criteria for penetrating or lamellar keratoplasty. According to the recorded family interview documentation for donation, all eyes underwent cataract surgery without postoperative complications. Globes with suspected or confirmed histories of postoperative complications were discarded. Donated eyes were evaluated and processed between 12 and 36 hours postmortem. Eyes were maintained in a 0.1 M phosphate-buffered solution (pH 7.4) containing antibiotics and antifungals (penicillin 100 IU/mL, streptomycin 10 mg/mL, and amphotericin B 2.5 μg/mL) at 4 C for 8–12 hours and then decontaminated by immersion in 40 mL of 5% povidone-iodine solution for 3 minutes under a laminar-flow hood.
Decontamination Efficacy of 5% Povidone-Iodine
The procedure was performed under a laminar-flow hood. Initially, the eye globe was removed from the antimicrobial maintenance solution and rinsed with 500 mL of sterile saline solution. A mark was made to divide the globe into 2 equal halves and a sterile swab was taken from half of the limbus and cornea. Next, the globe was fully immersed in 40 mL of 5% povidone-iodine for 3 minutes, removed, and rinsed with 500 mL of sterile saline to remove excess decontaminating agent that could inhibit microorganism growth. A postdecontamination sterile swab was then taken from the second half of the limbus and cornea, taking care not to swab the same area as in the pretreatment swab to avoid false-negative results. Pre- and postdecontamination swabs were rotated and soaked onto different culture media. The incubation conditions are summarized in Supplemental Table 1 (Supplemental Material available at AJO.com ). Isolates were identified by standard microbiological methods. The effectiveness of decontamination was determined by calculating the following: (1) the percentage reduction of positive ocular surfaces after decontamination and (2) the percentage reduction in the number of each bacterial species after decontamination.
Microbiological Analysis of Intraocular Fluids by Classical Culture Techniques
Samples of aqueous and vitreous humor were collected aseptically under a laminar-flow hood. The aqueous humor samples (100–150 μL) were collected by anterior chamber limbic paracentesis with a 30 G needle, whereas the vitreous samples (500 μL) were obtained by pars plana puncture 4 mm from the limbic region with a 19 G needle. Samples were divided into 2 aliquots: 1 aliquot per sample was kept at −80 C for further analysis and the other was inoculated onto different culture media. Microbiological analysis and procedures were carried out as described ( Supplemental Table 1 ).
Light and Electron Microscopy Studies
Posterior chamber IOLs and their corresponding capsule remnants were explanted from ocular globes under aseptic conditions. A portion of lens capsule and IOLs were immediately fixed in 2% paraformaldehyde + 2.5% glutaraldehyde solution in a 0.1 M phosphate buffer. Ocular globes with IOL implanted into the sulcus or anterior chamber were excluded. After chemical fixation over 12 hours, IOLs were examined with a binocular magnifying loupe, photographed, and classified into polymethylmethacrylate (PMMA), silicone, and hydrophobic or hydrophilic acrylic lenses.
For scanning electron microscopy (SEM), fixed IOLs were dehydrated in ethanol–water mixtures with increasing concentrations of ethanol (50%, 70%, 90%, 96%, and 100% ethanol by volume). After critical point drying, samples were stuck on metal holders with double-sided adhesive tape and coated with gold. The IOL surface observations were performed at 15 kV with an SEM (JEOL JSM-840; JEOL Ltd, Tokyo, Japan). The entire IOL surface was examined for the presence of adherent microorganisms or biofilm.
For transmission electron microscopy (TEM), capsule remnants were abundantly rinsed in phosphate-buffered saline, postfixed in 1% osmium tetroxide in 0.1 M phosphate-buffered solution (pH 7.4) for 1 hour, dehydrated in increasing graded concentrations of acetone, and then progressively embedded in resin (Spurr technique) for polymerization at 60 C. We obtained semi-thin (0.5–1.0 μm) and ultrathin (50–75 nm) sections by conventional ultramicrotomy (OmU2; Reichert-Jung, Wein, Austria). The semi-thin sections were placed on coverslips and stained with toluidine blue solution, then observed by light microscopy (BX61; Olympus R-FTL-T; Olympus America Inc, Center Valley, Pennsylvania, USA) coupled with the Olympus DP Controller Program for digital image acquisition. Ultrathin sections were placed on copper grids (200 mesh) and stained with uranyl acetate and lead citrate for conventional TEM (Hitachi 800 MT; Hitachi, Tokyo, Japan).
Microbiological Analysis by Molecular Techniques
To minimize the possibility of cross-DNA contamination, we performed DNA extraction, PCR mixture preparation, and post-PCR analyses in separate rooms, using designated equipment. Total DNA was extracted from intraocular fluids with a DNA purification kit (QIAamp DNA mini kit; Qiagen, Hilden, Germany) according to the tissue protocol, with a few modifications. The oligonucleotide primers designed for panbacterial and panfungal detection have been described previously, and they produced 590- and 654-base-pair fragments of 16S and 18S ribosomal DNA, respectively ( Supplemental Table 2 ; Supplemental Material available at AJO.com ). Positive control consisted of 10 ng of purified Escherichia coli or Candida albicans DNA for the 16S and 18S reactions, respectively. Sterile purified water was used as a negative control. PCR amplification was carried out in a GeneAmp PCR System 2400 thermocycler (Perkin-Elmer, Emeryville, California, USA) as follows: 35 cycles of 30 seconds at 94 C; 30 seconds at 52 C or 50 C, for bacterial and fungal DNA, respectively; and 40 or 45 seconds at 68 C for bacterial and fungal DNA, respectively; followed by 7 minutes of final elongation step at 68 C. Amplified products were purified using the Wizard SV Gel and PCR Clean-Up System (Promega Corporation, Madison, Wisconsin, USA) and sequenced using the previously described primers on an automated sequencer (ABI-3730 DNA Analyzer Sequencer System; Applied Biosystems, Thermo Fisher Scientific, London, UK) at CRAG (Centre de Recerca Agrogenòmica, Generalitat de Catalunya, Barcelona, Spain). The sequences obtained were compared with those available in the GenBank, EMBL, and DDBJ databases with the gapped BLASTN 2.0.5 program obtained from the NCBI information server ( http://www.ncbi.nlm.nih.gov/BLAST ). The minimal percentages to identify genus and species were used as recommended by the CLSI document MM18-A.
To confirm the 16S rDNA PCR results, molecular analysis by real-time quantitative PCR (qPCR) was performed at LEMC (Escola Paulista de Medicina, Universidad Federal de Sao Paulo, Sao Paulo, Brazil), as previously described. The universal primers NT-341Fw and 16S-522Rv were used to amplify a fragment of approximately 192 bp containing the V3 region ( Supplemental Table 2 ). A primer set and a probe for the β-globin gene was used as the internal control. The qPCR mix (Power SYBR Green PCR master mix; Life Technologies) was pretreated using 0.3 U of RQ1 RNAse-free DNAse (Promega) for 30 minutes at 37 C to remove contaminating bacterial DNA, followed by 50 minutes at 95 C to inhibit the DNAse activity. Amplification was performed on a real-time PCR system (model 7500; ABI) with the following conditions: 50 C for 2 minutes and 95 C for 10 minutes followed by 40 cycles at 95 C for 15 seconds and 60 C for 60 seconds. Melting curve analysis was performed after amplification, by heating samples gradually from 68 C to 95 C at a rate of 0.5 C/s. The amplification of clinical samples was performed in the presence of a negative control, including all PCR reagents, except the DNA template and positive control samples, with 10 ng of S epidermidis DNA. After the reaction, a 15 μL PCR aliquot was purified with a PCR purification kit (QIAquick; Qiagen). DNA molecules were sequenced in both directions with dye-termination chemistry (Big Dye Terminator; 3000 Genetic analyzer; ABI). The sequences obtained were edited (SeqMan; DNASTAR, Madison, Wisconsin, USA) and searched for similarity in GenBank using the BLAST (BLASTN algorithm) program with automatically adjusted parameters. The minimal percentages needed to identify genus and species were used, as recommended by CLSI document MM18-A.
Statistical Analysis
Results were compared globally using the nonparametric Kruskal-Wallis test (SPSS for Windows, version 22.0; SPSS Inc, Chicago, Illinois, USA). Then the Mann-Whitney nonparametric test was used to compare pairs of materials. Two-sided P values ≤.05 were considered statistically significant.
Results
Decontamination Procedure Efficacy
The efficacy of chemical decontamination with 5% povidone-iodine was controlled (n = 20). Initial treatment with the antimicrobial maintenance solution eliminated the external microbiota in half of the globes (n = 10) selected for decontamination control efficacy. The microbial flora found on the ocular surface before treatment was similar to the microbial flora found in the conjunctiva and eyelids of healthy eyes. More than 1 species was isolated in most studied globes; commensal species predominated, with 7 isolates of coagulase-negative staphylococci (33.3%), 6 isolates of Corynebacterium species (28.6%), and 2 isolates of Micrococcus spp. (9.5%). Other noncommensal microorganisms, including S aureus and Streptococcus spp., were present less frequently. The 5% povidone-iodine solution completely decontaminated 90.0% of ocular globes (n = 9), with an efficacy of 95.2% in eliminating commensal species, including the most prevalent ones ( Supplemental Table 3 ; Supplemental Material available at AJO.com ). The 5% povidone-iodine was very effective at decontaminating the ocular surfaces of globes donated for transplantation.
Microbiological Analysis of Intraocular Fluids
Microorganisms were not detected in either the aqueous or vitreous humor samples (n = 69 per intraocular fluid) cultured by conventional microbiologic techniques.
Light and Electron Microscopy Studies
IOLs observed by binocular magnifying loupe were classified according to the biomaterial of the lens (PMMA, silicone, hydrophobic acrylic, or hydrophilic acrylic). When analyzed by SEM, most of the IOLs showed cellular epithelial aggregates and crystalline fiber remnants. Bacterial biofilm was absent in 81.2% (n = 56) and probable in 18.8% (n = 13) of IOL surfaces studied ( Table 1 ). IOLs classified as having probable biofilm showed isolated or aggregated cocci (about 1 μm) on the surfaces of 35.0% of PMMA IOLs (n = 7), 28.6% of silicone IOLs (n = 2), 11.5% of acrylic hydrophilic IOLs (n = 3), and 6.3% of acrylic hydrophobic IOLs (n = 1) ( Figures 1–4 ). Significant difference in the prevalence of cocci on acrylic hydrophobic biomaterial and PMMA ( P < .042) was present. Interestingly, probable biofilm formation was also more evident in the periphery of all IOL optic surfaces ( Figures 1–4 ). In 4 of the 13 IOLs, the cocci were embedded by some extracellular debris ( Figure 4 ). The prevalence of suspected biofilm formation on the surface of IOLs is summarized in Table 2 .
IOL Material | Total | Absent | Probable |
---|---|---|---|
N | N (%) | N (%) | |
PMMA | 20 | 13 (65.0) | 7 (35.0) |
Hydrophilic acrylic | 26 | 23 (88.5) | 3 (11.5) |
Hydrophobic acrylic | 16 | 15 (93.8) | 1 (6.3) |
Silicone | 7 | 5 (71.4) | 2 (28.6) |
TOTAL | 69 | 56 (81.2) | 13 (18.8) |
IOL | IOL Material | Biofilm on IOL by SEM Observation | Microbial-like Structures on IOL by SEM Observation | Sample | PCR Sample | PCR | q-PCR | |
---|---|---|---|---|---|---|---|---|
18S | 16S | 16S | ||||||
2 | Silicone | Probable | Mainly cocci and few rods | 1 | Aqueous | NA | NA | NA |
2 | Vitreous | NA | NA | NA | ||||
5 | Acrylic hydrophobic | Probable | Mainly cocci and few rods | 3 | Aqueous | NA | NA | NA |
4 | Vitreous | NA | NA | NA | ||||
8 | PMMA | Probable | Mainly cocci surrounded by ocular tissue remains | 5 | Aqueous | NA | NA | NA |
6 | Vitreous | NA | NA | NA | ||||
16 | PMMA | Probable | Mainly cocci surrounded by ocular tissue remains | 7 | Aqueous | NA | Massilia timonae | Massilia timonae |
8 | Vitreous | NA | Massilia timonae | Massilia timonae | ||||
19 | Acrylic hydrophobic | Absent | No | 9 | Aqueous | NA | NA | NA |
10 | Vitreous | NA | NA | NA | ||||
21 | Acrylic hydrophilic | Probable | Mainly cocci surrounded by ocular tissue remains | 11 | Aqueous | NA | NA | NA |
12 | Vitreous | NA | NA | NA | ||||
24 | Acrylic hydrophilic | Probable | Mainly cocci and few rods | 13 | Aqueous | NA | NA | NA |
14 | Vitreous | NA | Propionibacterium acnes | Propionibacterium acnes | ||||
27 | PMMA | Probable | Mainly cocci and few rods | 15 | Aqueous | NA | Moraxella catharralis | Moraxella catharralis |
16 | Vitreous | NA | NA | NA | ||||
28 | PMMA | Probable | Mainly cocci and few rods | 17 | Aqueous | NA | NA | NA |
18 | Vitreous | NA | Propionibacterium acnes | Propionibacterium acnes | ||||
30 | PMMA | Probable | Mainly cocci surrounded by ocular tissue remains | 19 | Aqueous | NA | Massilia timonae | Massilia timonae |
20 | Vitreous | NA | Massilia timonae | Massilia timonae | ||||
45 | PMMA | Probable | Mainly cocci and few rods | 21 | Aqueous | NA | NA | NA |
22 | Vitreous | NA | NA | NA | ||||
46 | PMMA | Probable | Mainly cocci and few rods | 23 | Aqueous | NA | NA | NA |
24 | Vitreous | NA | NA | NA | ||||
49 | Silicone | Probable | Mainly cocci and few rods | 25 | Aqueous | NA | NA | NA |
26 | Vitreous | NA | NA | NA | ||||
53 | PMMA | Absent | No | 27 | Aqueous | NA | NA | NA |
28 | Vitreous | NA | NA | NA | ||||
65 | Acrylic hydrophobic | Probable | Mainly cocci and few rods | 29 | Aqueous | NA | NA | NA |
30 | Vitreous | NA | NA | NA |
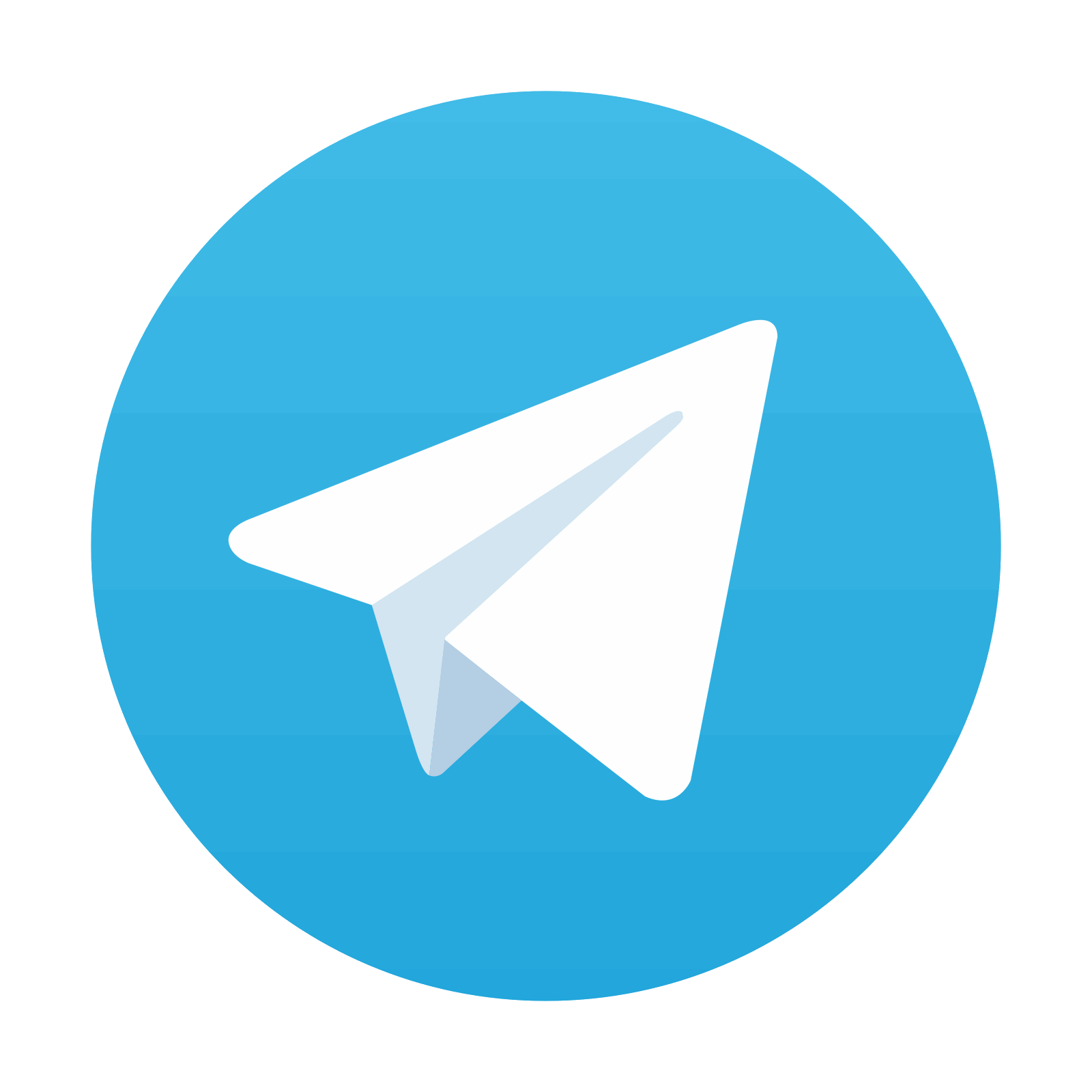
Stay updated, free articles. Join our Telegram channel

Full access? Get Clinical Tree
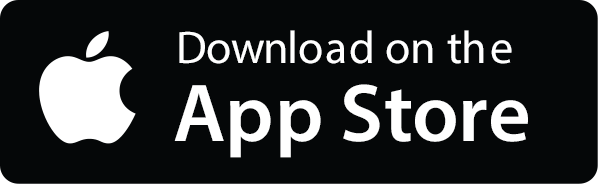
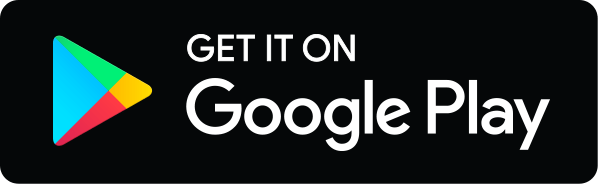
