Organism
Stains
Culture media
PCR target
Confocal microscopy
Bacteria
Aerobic
Gram
Blood
16 s RNA
No, exc. specific patterns, e.g., infectious crystalline keratopathy
Giemsa
Chocolate
Acridine orange
Broth (thioglycollate, eugonic, etc.)
Anaerobic
Gram
Blood (anaerobic incubation)
16 s RNA
No
Giemsa
Broth (thioglycollate, eugonic, etc.)
Acridine orange
Mycobacteria
Acid fast
Lowenstein-Jensen
16 s RNA
No
Ziehl-Neelsen
7H11
Nocardia
Gram
Buffered charcoal agar with yeast
Hsp65
Yes
Acid fast
extract (BCYE)
16 s rRNA
Sabouraud’s dextrose agar (SDA) slant
Fungi
Yeasts
Giemsa
SDA with chloramphenicol or gentamicin
18 s rRNA
Yes
Periodic acid-Schiff
Brain Heart Infusion (BHI)
28 s rRNA
Calcofluor white
Blood (25 °C)
ITS
Filamentous molds
Giemsa
SDA with chloramphenicol or gentamicin
18 s rRNA
Yes
Calcofluor white
Brain Heart Infusion (BHI)
28 s rRNA
Acridine orange
Blood (25 °C)
ITS
Gomori methenamine silver
KOH prep
Virus
Herpes simplex
Direct fluorescence antigen (DFA)
Human cell line (A549, MRC-5, others)
Thymidine kinase
No
Tzanck
Serotyping
DNA polymerase
Adenovirus
Indirect immunofluorescence
Human cell line
Hexon
No
Serotyping
Fiber
Parasitic
Acanthamoeba
Giemsa
Non-nutrient agar with bacterial overlay
18 s rDNA
Yes
Calcofluor white
BCYE
Acridine orange
Sabouraud’s
KOH
Hematoxylin-Eosin
Microsporidia
Giemsa
Cell lines
16 s rRNA
Yes, uncommon
Calcofluor white
Monkey (Vero)
18 s RNA
Modified trichrome
Rabbit (RK-13)
ITS
Gram
Human (MRC-5)
Canine (Madin-Darby)
Clinical examination may also be helpful, although acanthamoebal and herpetic infections at various stages may mimic other pathogens as well as noninfectious etiologies of keratitis. Multiple studies have, however, confirmed the pitfalls of basing empiric treatment solely on the history and clinical appearance, but they may offer direction in the absence of positive studies (Dahlgren et al. 2007; Mascarenhas et al. 2012; Dalmon et al. 2012). Prior corticosteroid use will significantly alter the appearance and prognosis of any form of infectious keratitis and should be noted in context to its clinical appearance. Corneal scrapings offer minimal volume for examination and, therefore, need to be apportioned carefully to the studies that offer the highest probability of yield. Standard tests include Gram and Giemsa stains, direct plating of blood agar and chocolate agar for bacterial species, as well as Sabouraud’s dextrose agar (Fig. 2.1) without antifungal additives for fungal isolation and a broth or other media for anaerobic organisms (Bhadange et al. 2013). Superficial scrapings may be obtained either with a moistened calcium alginate swab or metallic instrument such as a platinum spatula or scalpel blade with reasonably equal yields (Benson and Lanier 1992).
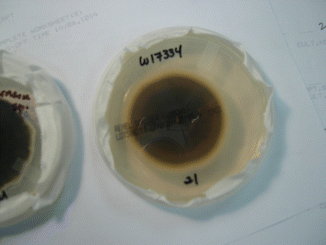
Fig. 2.1
A subcultured colony of an Alternaria alternata keratitis isolate on Sabouraud’s agar
2.2.1 Bacteria
The most common type of corneal infections in developed countries is caused by bacteria usually presenting as a single, suppurative lesion with significant pain, photophobia, and intraocular inflammation (Fig. 2.2). A history of ocular surface compromise, chronic corticosteroid use, ocular surgery, and/or contact lens wear should suggest first a bacterial etiology. Besides simple detection of bacteria, a Gram stain should be helpful in directing therapy since the activity of most antibacterials act on the bacterial cell wall type identified by a Gram-positive or Gram-negative result. Unfortunately, numerous studies have shown a poor correlation with subsequent culture results making the Gram stain unreliable in this regard (Sharma et al. 2002). In an untreated ulcer, standard testing methods should yield an organism in about 70 % of cases (Bourcier et al. 2003). Acid-fast stains may be more helpful in detecting atypical mycobacteria in the setting of chronic corticosteroid use in ocular surface disease or, more recently, LASIK surgery (Fig. 2.3). These lesions are minimally necrotic with small raised white lesions that are gritty on corneal scraping. Pain is often significant in these patients. Lowenstein-Jensen media and 7H11 agar slants offer an environment conducive to the isolation of these organisms. Nocardia, a filamentous bacterium, is also partially acid fast and is most often detected with Gram or acid-fast stains in about 65 % of cases and grow slowly on charcoal agar with a yeast extract additive (BCYE) (DeCroos et al. 2011). The lesions may have a wreath-like appearance with raised edge and an irregular base. Antibiotic sensitivity testing should be performed on all isolates so that the information is available if a patient does not respond appropriately to empiric therapy.
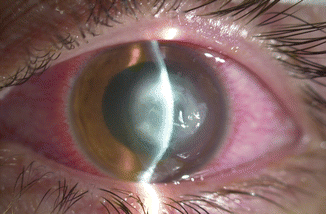
Fig. 2.2
An acutely suppurative contact lens-related Pseudomonas corneal ulcer
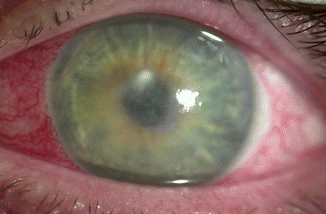
Fig. 2.3
Atypical mycobacteria after LASIK with limited inflammation sequestered in the corneal interface
2.2.2 Fungi
The presentation of fungal keratitis is species dependent with the most common filamentous mold, Fusarium, presenting early with significant pain and proportionally less inflammation than a comparable bacterial ulcer but eventually becoming more infiltrative and characterized by a hypopyon, endothelial plaque, satellite lesions, as well as severe pain and injection (Fig. 2.4). Fusarium may rapidly and directly penetrate into the deep stroma and past an intact Descemet’s membrane to enter the intracameral space. However, slower-growing species of Alternaria or Beauveria may be much more indolent with less inflammation, less pain, and a more superficial appearance. Candida spp. are the most common overall fungal keratitis pathogen in the more temperate climates of North America and present initially with a creamy-white, superficial infiltrate, but its pseudohyphae may penetrate into deep stroma (Figs. 2.5 and 2.6).
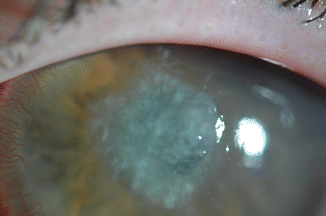
Fig. 2.4
Fusarium keratitis in a contact lens wearer
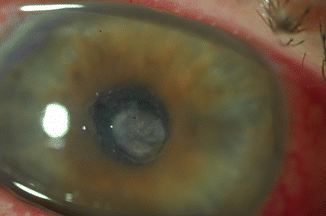
Fig. 2.5
Candida ulcer in a patient on chronic topical corticosteroids
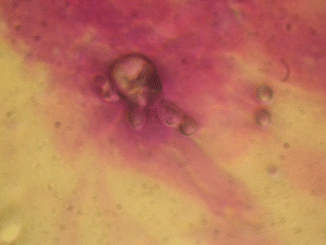
Fig. 2.6
Budding Candida sp. on a Diff-Quik smear (original magnification 100×)
Although yeast and filamentous molds may both be detected on Gram stain, molds are more readily identified on stains that highlight cell walls such as a Giemsa stain and KOH preps where hyphae are easily detected (Fig. 2.7). Similarly, acridine orange and calcofluor white stains have been shown to be more sensitive in detecting larger pathogens like fungi and acanthamoeba but require an epifluorescent microscope to excite the stain (Gomez et al. 1988). The majority of ocular fungal pathogens can be isolated with Sabouraud’s dextrose agar or Brain Heart Infusion Agar with non-antifungal additives, e.g., chloramphenicol or gentamicin, since these additives do not affect the saprophytic fungi usually nonpathogenic in other organs (Bhadange et al. 2013). Alternatively, fungal keratitis pathogens will also grow on standard blood and chocolate agar plates incubated at 36 °C. Antifungal sensitivities have been poorly reflective, historically, of clinical drug efficacy, but recent refinement of CLSI testing methods and break points have improved correlation of in vitro and in vivo antifungal activity, the knowledge of which can be critical to a successful outcome (Oechsler et al. 2013).
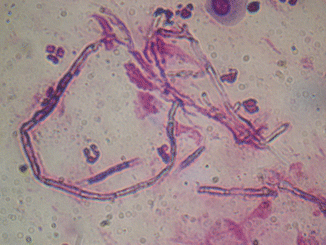
Fig. 2.7
Diff-Quik stain of Fusarium sp. hyphae from a corneal scraping (original magnification 100×)
2.2.3 Virus
A diagnosis of herpetic viral disease is most commonly based on the history and clinical appearance. Herpes simplex keratitis is due more commonly HSV 1, associated with above-the-waist disease, but HSV 2 is also commonly isolated (Fig. 2.8). Patients may have a history of oral or genital herpes simplex as well as a past history of vesicular lesions in and around the eye. Although recurrent disease may surface from latency established from a primary infection from any dermatome served by the trigeminal ganglion, recurrences from prior corneal infection is usual. Epithelial HSV keratitis demonstrates a characteristic dendritic pattern with terminal bulbs, leaving an imprint on the anterior stroma after resolution. Geographic lesions may also occur with both stained by Rose Bengal of fluorescein dyes. Primary disease is almost always epithelial, but recurrences may be an epithelial or stromal interstitial keratitis with a conjunctival limbitis, endotheliitis, or uveitis less common. Patients with atopic keratoconjunctivitis are especially susceptible to severe, bilateral disease. The need for microbiologic confirmation is restricted to neonates and to those patients where the diagnosis is in question. Samples may be obtained through epithelial debridement, swab, or impression cytology of the superficial lesion and submitted for culture, immunofluorescence (IFA), or polymerase chain reaction (PCR) detection. Culture is the least sensitive method while both PCR and IFA have been shown to have similar sensitivities (60–80 %) and high specificities for the detection of HSV-1 in ocular samples (Farhatullah et al. 2004). Serologic testing may be helpful in naïve patients, especially children to rule out HSV as a potential pathogen, but a significant majority of adults have had previous exposure making a positive test unhelpful.
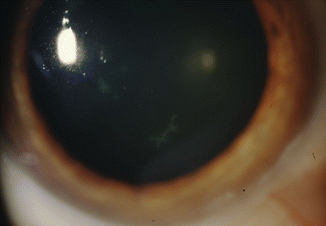
Fig. 2.8
HSV epitheliitis seen in a chronically systemically immunosuppressed chemotherapy patient
Other herpesviruses including herpes zoster (HZV), Epstein-Barr virus, and cytomegalovirus have been described to cause keratitis. Herpes zoster may manifest as pseudodendritic lesions (poorly branching, absent terminal bulbs), mucoid plaques, stromal keratitis, and/or uveitis characteristically leaving sectoral iris atrophy. Whether some or all of these entities represent immune response or active viral disease is controversial, but virus has been detected in late dendritic disease of HZV which are highly responsive to antiviral therapy (Pavan-Langston et al. 1995). Epstein-Barr virus more commonly causes a nummular keratitis but may also manifest as subepithelial infiltrates or a deep stromal keratitis. Acute and convalescent titers of Epstein-Barr-related IgM and IgG and EBV early antigen may be helpful in this setting. Monoclonal antibodies and PCR of corneal scrapings have been reported but are rarely done (Pflugfelder et al. 1990). Adenoviral epidemic keratoconjunctivitis may similarly be isolated and genotyped from swabs of the ocular surface but is seldom performed except in the setting of tracking large outbreaks of disease. A rapid in office antigen test has been available with a reasonable sensitivity (88 %) and specificity (91 %) to guide treatment and isolation of certain patients (Sambursky et al. 2006).
2.2.4 Parasitic
A number of different parasitic organisms can cause corneal disease. Parasites that are primarily spread to the cornea from endogenous sources include Onchocerca, Leishmania, and Trypanosoma are a devastating source of corneal blindness worldwide. They manifest as peripheral stromal interstitial keratitis at their site of entry, although frank corneal ulceration may sometimes occur. Acanthamoeba represents the most common ocular parasitic pathogen in Western world. Contact lens wear (>90 %) is the strongest risk factor for Acanthamoeba keratitis in that region with nearly 20 cases per million contact lens wearers per year afflicted in the UK with similar numbers now in the USA (Tu and Joslin 2010). Additional risk factors include exposure to contaminated domestic or environmental water while wearing or caring for the lenses and other hygiene related factors (Tu and Joslin 2010). The infection presents in many forms including a diffuse or pseudodendritic epitheliopathy, anterior stromal keratitis, interstitial keratitis, a ring infiltrate, and/or perineural infiltrates leading to confusion with other infectious and noninfectious processes (Fig. 2.9). Pain in the early stages is minimal but increases with the presence of perineural disease or deep stromal keratitis. Corneal scrapings can be subject to Giemsa stain, acridine orange, KOH preps, and calcofluor white for diagnosis with reasonable sensitivity and specificity (Fig. 2.10). Culture on non-nutrient agar with a bacterial overlay (higher yields obtained with Enterobacter than E. coli) or charcoal agar is relatively simple, but sensitivities are generally low between 35 and 50 %. Microsporidia keratitis has been increasingly described in immunocompetent individuals as causing an epithelial/subepithelial keratitis and a stromal interstitial keratitis. These patients usually have some history of exposure to soil or water and have been predominantly described in South Asia. Because it is an obligate intracellular organism, culturing microsporidia is difficult, requiring canine kidney cells for growth. Histologic stains have, therefore, been relied upon to make the diagnosis.
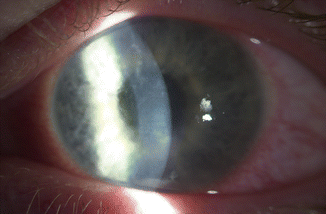
Fig. 2.9
Acanthamoebal epitheliopathy with perineuritis
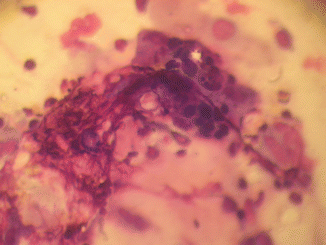
Fig. 2.10
Acanthamoebal cysts on Diff-Quik stain demonstrating classic “pores” in the cyst wall (original magnification 100×)
2.2.5 Corneal Imaging and Corneal Biopsy
Confocal microscopy refers to the precise timing of illumination and imaging at a specific focal point or plane to achieve high degrees of magnification, minimizing motion blur and light scatter. The cornea is ideal for this type of imaging because of its relative clarity and lack of reflective elements, to highlight areas of abnormality. Resolution of these instruments are in the 1–2 μm range, making it impossible to image most bacteria and viruses, but specific patterns, e.g., infectious crystalline keratopathy where a biofilm insinuates itself through cornea lamellae, owl eye cells in CMV endotheliitis, and filamentous bacteria (Nocardia) can be recognized (Elmallah et al. 2006; Vaddavalli et al. 2006;. Kobayashi et al. 2012). The greatest utility of the instrument in corneal infectious disorders has been to detect larger pathogens which do not cause dense suppuration such as fungi, acanthamoeba, and, to a lesser extent, Microsporidia (Sagoo et al. 2007). Both cysts and trophozoites can be seen, but the cysts are most characteristic observed as a double-walled cyst or a paired “coffee bean” appearance isolated or in chains (Fig. 2.11). The detection of Acanthamoeba keratitis has been shown in some centers to have a high sensitivity (>90 %) and high specificity ( 100 %) when compared to culture and other microbiologic methods (Tu et al. 2008a) but has also been reported to be significantly lower in other facilities (Hau et al. 2010). Similar results have been reported in the detection of the slender, septate branching of corneal fungal pathogens (Vaddavalli et al. 2011; Tu and Park 2007) as well as yeast. Confocal microscopy is invaluable in imaging deeper lesions without easy access for scraping such as Candida interface keratitis after endothelial keratoplasty (Lee et al. 2011).
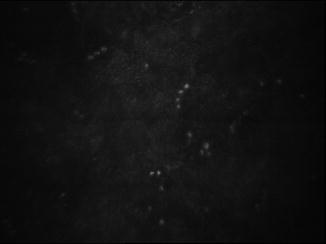
Fig. 2.11
Confocal microscopy of Acanthamoeba exhibiting the classic paired “coffee-bean” appearance on a slit-scanning instrument
Although confocal microscopy has largely supplanted corneal biopsy in many institutions, it remains a relevant technique for refractory or deep keratitides that otherwise defy identification. A number of techniques have been described, but all involve dissection of a sample of solid corneal tissue either from the anterior lamellae or from mid- to deep stroma using a flap created manually or via a femtosecond laser (Kim et al. 2008). Specimens are sent for histopathology and microbiologic studies with a success of greater than 40 % in identifying a causative organism in a recent review (Younger et al. 2012). Corneal scarring or perforation can be significant risks to corneal biopsy.
2.2.6 Molecular Diagnosis
Molecular diagnosis refers to identification of pathogens from their molecular signature, primarily DNA or RNA which by definition is unique to each organism. Utilizing primers either specific to an organism, a class of organisms, or universal primers, a single copy of DNA can be multiplied a million times over a period of hours, allowing its easy sequencing. Once its sequence has been determined, it can be matched fully or partially to previously sequenced organisms for identification. Because of its high sensitivity, however, false-positive results can be seen because of contamination at any stage of specimen recovery or with organisms that may be present or nonviable but not pathogenic. Extraneous compounds such as topical anesthetic or fluorescein can decrease the sensitivity of the assays (Goldschmidt et al. 2006). Quantitative and real-time PCR with appropriate validation can reduce the number of false-positive test results. It is most useful in detecting organisms not normally present in the eye and/or is difficult to confirm by other means. For example, PCR of tears or corneal scrapings directed toward the HSV polymerase gene has largely supplanted culture for herpes simplex ocular disease because of culture’s low sensitivity for an organism which is not normally found in the eye (Farhatullah et al. 2004; Satpathy et al. 2011). Identification of bacterial pathogens is based on their 16 s ribosomal DNA sequences (Prabhasawat et al. 2010), while a more significant experience with the 18 s ribosomal RNA region for the diagnosis of fungal keratitis has proven its utility (Vengayil et al. 2009; Ferrer and Alio 2011). Recent development of acanthamoebal PCR should lead to its increasing study and validation (Thompson et al. 2008; Goldschmidt et al. 2009).
2.3 Management
Unlike many other organ systems, comparatively small alterations in corneal structure and clarity can profoundly affect lifelong visual function. This combined with the relative inability of the corneal immune response to limit corneal infection makes it imperative to rapidly eliminate pathogens and modulate the corneal immune response to retain as much vision as possible. Empiric therapy is, therefore, directed not only against the most likely responsible pathogens but also against those pathogens most rapidly destructive of the corneal stroma specifically Gram-positive and Gram-negative bacteria. Although other pathogens may be more common in certain regions and mechanisms of injury, atypical bacteria, acanthamoeba, microsporidia, filamentous molds, and yeast are all comparatively slow growing, whereas bacteria may destroy the cornea in a matter of hours.
Since the availability and success of single-drug empiric therapy in the early 1990s, corneal scrapings for culture and smear are no longer routinely employed as part of the initial management of a routine bacterial corneal ulcer. However, microbiologic yields are highest before initiation of any antimicrobial therapy and should be strongly considered in large or deep ulcers, ulcers near the visual axis, ulcers failing prior therapy, and ulcers with either an atypical appearance or history at presentation. Suspicion for a larger, atypical organism at any stage should prompt consideration of confocal microscopy, although corneal biopsy should be reserved for later in the process because of its more invasive nature and higher risk of complication. Ongoing therapy should be directed by initial response or lack of response to initial therapy and directed by microbiologic examination when available.
2.3.1 Bacteria
Since the commercial availability of broad-spectrum fluoroquinolones in the early 1990s, single-drug therapy of presumed bacterial keratitides has been shown to have similar outcomes to multiple, fortified drug therapy (O’Brien et al. 1995; Constantinou et al. 2007). Single-drug therapy has the advantage of availability, easier compliance, and less time off of work, but some studies have pointed to a higher rate of corneal perforation presumably related to its activation of matrix metalloproteinases (Constantinou et al. 2007; Mallari et al. 2001). Although they are considered broad spectrum, having activity against both Gram-positive and Gram-negative bacteria, fluoroquinolones are commonly divided into second (ciprofloxacin, ofloxacin), third (levofloxacin), and fourth (moxifloxacin, gatifloxacin, besifloxacin) generations which predicts their specific antibacterial efficacy. Of these, only besifloxacin has pursued the FDA indication, but more extensive clinical studies of infectious keratitis have sufficiently established the particular spectrums of efficacy of the other fluoroquinolones in bacterial corneal ulceration. In general, the best Gram-negative activity, including Pseudomonas, is seen with the second-generation fluoroquinolones, while the fourth-generation fluoroquinolones have added some Gram-positive and atypical mycobacterial coverage. Other antibiotics need to be “fortified” to achieve effective corneal concentrations and combined together to afford the broad-spectrum coverage needed for empiric therapy. This usually consists of topical vancomycin or cephalosporin prescribed with an aminoglycoside like gentamicin or tobramycin.
Inducible antibiotic resistance is increasingly seen in both systemic and ocular infections. For corneal isolates specifically, methicillin-resistant Staphylococcus aureus (MRSA) has been increasingly reported in postsurgical corneal ulceration, and the laboratory resistance of Pseudomonas sp. to fluoroquinolones is rising secondary to both local and systemic use (Ray et al. 2013; Fintelmann et al. 2011). Despite laboratory resistance, topical ophthalmic antibiotics generally deliver sufficient concentrations of drugs to be clinically effective, but infections may be slower to resolve (Wilhelmus et al. 2003). While MRSA demonstrates high levels of fluoroquinolone resistance, vancomycin still remains almost universally effective against ocular pathogens. Vancomycin-resistant enterococcus is a rare but increasingly reported pathogen for infectious keratitis but may be sensitive to newer antibiotics like linezolid (Tu and Jain 2013). Similarly, 4th-generation fluoroquinolones have some activity against atypical mycobacteria, but primary therapeutic options remain topical fortified amikacin or clarithromycin with recent reports of linezolid sensitivity. Nocardia spp. are sensitive to fluoroquinolones but may also respond to amikacin and topical sulfamethoxazole-trimethoprim.
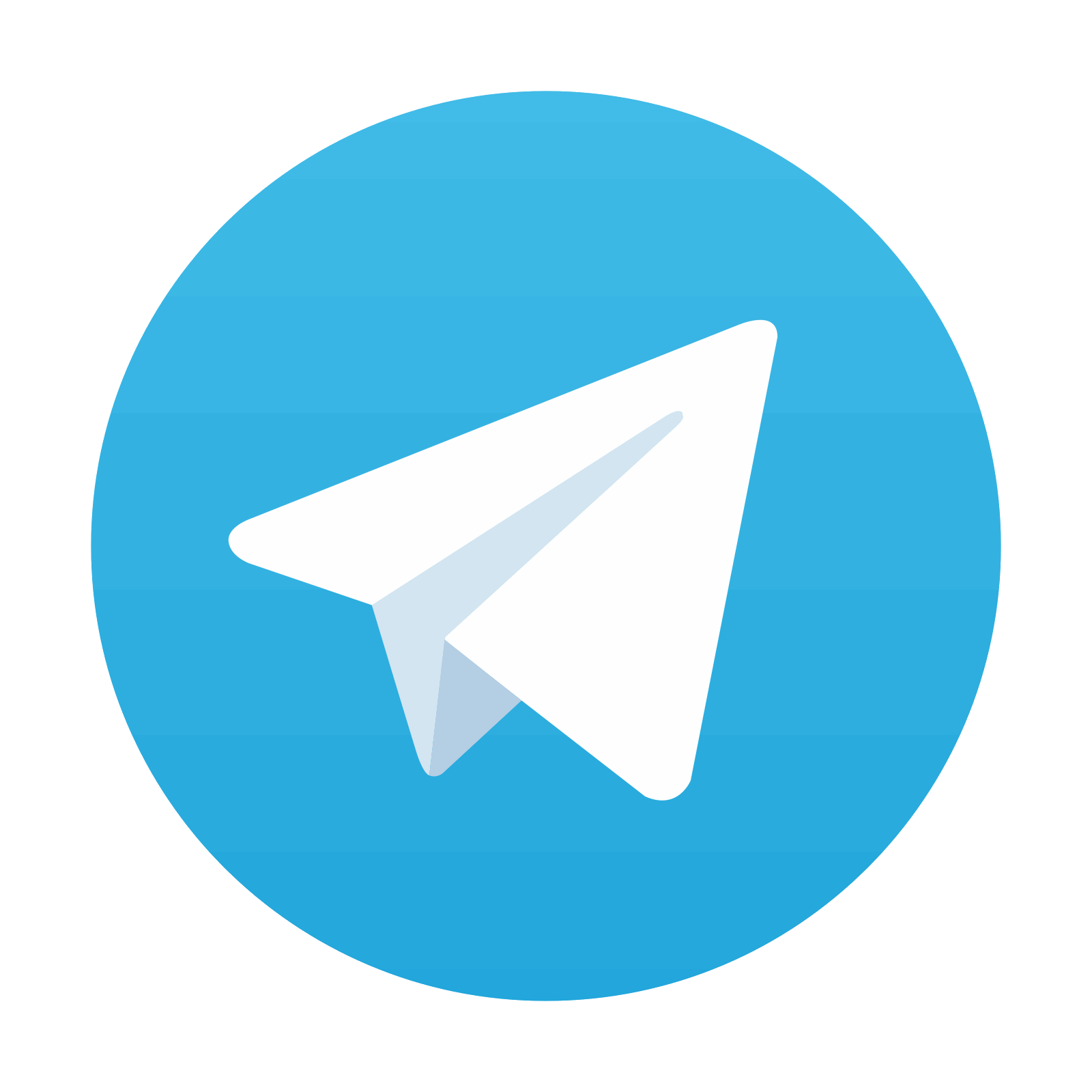
Stay updated, free articles. Join our Telegram channel

Full access? Get Clinical Tree
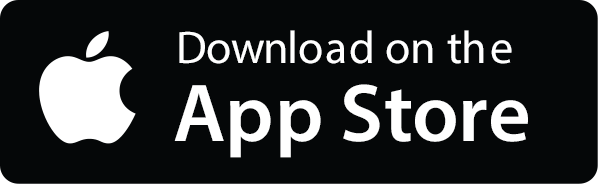
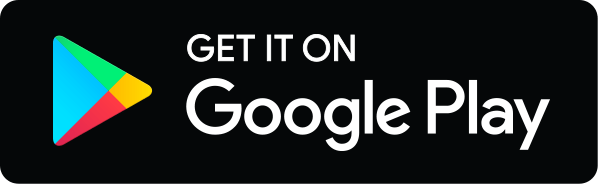