Introduction
In 2005, the Department of Veterans Affairs awarded more than $1 billion for otologic-related disabilities—the majority of which were due to impulse noise exposures and blast injuries. The ear is the body’s most sensitive pressure transducer, and as a result, otologic complaints are common after blasts. Fortunately, blast trauma is a rare occurrence in the United States. As a result, much of the civilian literature is drawn from the Boston Marathon bombings, Afghanistan War, and Iraq War. Unique to blast-related otologic trauma, acute management is often dictated by the tactical situation at hand and by the severity of other injuries. In this chapter, we will review evaluation and management patients exposed to otologic blast injuries based on military and civilian experiences as well as animal studies ( Table 5.1 ).
Otologic injuries: |
---|
Soft tissue injuries |
Auricular laceration |
Auricular hematoma |
Temporal bone fractures |
External ear injuries |
External auditory canal lacerations |
Tympanic membrane |
Middle ear injuries |
Ossicular chain discontinuity/fracture |
Inner ear injuries |
Cochlea |
Sensorineural hearing loss |
Tinnitus |
Vestibular system |
Vestibular organ damage |
Benign paroxysmal positional vertigo |
Postconcussive migraines/vestibular migraines |
Semicircular canal dehiscence |
Otologic blast injuries
Aural soft tissue trauma
Facial and neck injuries are common in warfare as these areas are incompletely protected by body armor and helmets. Even worse, civilians caught in war zones or terrorist attacks usually have no protection. Acute ear management is highly dependent on the severity of other nonotologic injuries, local resources, the nearest facility with surgical capabilities, and if the patient can be evacuated from the site of injury. History taking is usually abbreviated in this setting, and otologic injuries will not have priority compared with more emergent injuries. For example, the incidence of emergent surgeries and perioperative mortality rates were higher in Iraq than in Afghanistan, and as a result, US soldiers and civilians were less likely to be cared for by head and neck surgeons in Iraq.
During the initial trauma evaluation, a thorough examination of the pinna and surrounding soft tissue should be performed. Profusely bleeding soft tissue injuries should be addressed, and initial surgery should be limited to primary closure and soft tissue coverage of exposed bone. Mastoid ecchymosis (otherwise known as Battle’s sign) may be indicative of a skull base fracture and brain trauma. This usually takes at least one day to appear, so examination of the postauricular region should not be overlooked after the initial trauma evaluation.
The external auditory canal should be evaluated for any soft tissue lacerations and tympanic membrane perforations. If an otoscope or microscope is not available, a headlight, loupes, and nasal speculum may be used instead. This is often necessary even in the hospital since most initial exams are at the bedside. Many such patients, however, have other injuries that require acute management in the operating room. It often is possible to perform an operative microscope exam under anesthesia simultaneously, if not interfering with the other surgeons, and they usually are accommodating. During this time, remove as much foreign material (e.g., metal fragments, wood, dirt, pebbles) as possible. Saline irrigation may be used to clear blood and foreign debris.
Tympanic membrane perforation
If a tympanic membrane perforation is present, then unfurl the remnants to avoid cholesteatoma formation. These remnants can also be supported with absorbable foam or a paper patch, but this is not the time to attempt a formal tympanoplasty. Apply an ototopical fluoroquinolone if there is a moist or contaminated perforation or soft tissue injury to the external auditory canal. Topical aminoglycosides should be avoided since they may be ototoxic and risk sensorineural hearing loss and vestibulopathy. There is no need for topical antibiotics with a clean and dry perforation as this may delay healing. However, many of these patients also have acute sensorineural hearing loss, for which middle ear delivery of steroids (e.g., dexamethasone) may be indicated, although this too may influence closure of the tympanic membrane perforation.
The detonation of one pound of trinitrotoluene (TNT) at a 15-feet distance is approximately 180 dB SPL. Blast pressures greater than five pounds per square inch (34.5 kPa) above atmosphere can rupture the tympanic membrane. A murine study that examined tympanic membrane perforation following exposure to 30 or 63 kPa blast waves reported significant and unanimous tympanic membrane damage at 63 kPa (mean perforation size of 57.8 ± 3.5% ( n = 5) of the total surface area). Smaller perforations (mean perforation size of 5.9 ± 1.7% of the total surface area) were observed in some animals exposed to the 30 kPa blast level. Tympanic membrane perforations are common after explosions and have been reported to be present in 5%–90% of explosion-wounded patients. , , Proximity to the blast and significant nonotologic injuries have been reported to be positive predictors of tympanic membrane perforation.
Spontaneous tympanic membrane perforation closure occurs in 38%–82% of patients , particularly with smaller perforations (<50% of the tympanic membrane). Surgical intervention via tympanoplasty may be necessary for persistent perforations, especially when there is conductive hearing loss and/or persistent otorrhea. These perforations should be given sufficient time to heal spontaneously, and it is possible for even large perforations to heal, or at least partially. Six weeks of observation is typical but can be extended if subsequent visits show that the perforation is getting smaller. These patients often need to wait this long anyway as they are being treated for other injuries, and some patients with multiple amputations have had upward of 40 surgeries. Tympanoplasty should be delayed as anesthesia induction and intubations risk displacing grafts in the middle ear. Also, many of these patients are on anticoagulants, and this should be taken into consideration. The success rates of tympanoplasties (∼82%-85%) for blast-related perforations are lower than non–blast-related perforations due to the size of the perforation (e.g., total or near-total), ossicular displacement, and inflammatory responses to foreign material , and this is despite normal eustachian tube function.
The same tympanoplasty techniques that are used for chronic ear surgery are applicable to blast-induced perforations. These perforations tend to be large so simple transcanal fascia underlays are usually insufficient. More advanced techniques such as lateral grafting, anterior tab pull-through, and the over–under technique are usually necessary. , Whatever technique is used, it is important to inspect the tympanum for implanted epithelium and cholesteatomas , ; endoscopes are useful adjuncts for examining the hidden recesses of the middle ear (retrotympanum, protympanum, etc.). It is also important to inspect for ossicular discontinuity and perilymphatic fistulas. Ossiculoplasty is usually best performed at a second stage given the concerns of cholesteatoma with keratin implantation following implosion injury.
Sensorineural hearing loss
Hearing loss and tinnitus often follow blast injuries to the ear. For example, among 36,917 Afghanistan and Iraq war veterans that underwent traumatic brain injury evaluations, 65.7% reported subjective auditory impairments.
Impulse noise (such as from rifles or supersonic blast waves from explosions) is more damaging than sustained noise exposure, as cochlear injury is both mechanical and biochemical. Blasts cause stereociliary bundle disruption that results in immediate outer hair dysfunction. It used to be thought that noise damage was limited to within the cochlea. This is the most immediate result, but it is now known that the damage is more extensive and progressive and can eventually cause auditory nerve degeneration. For example, in vivo cochlear imaging of mice exposed to blast waves revealed endolymphatic hydrops, hair cell loss, and cochlear synaptopathy. Another histological animal study demonstrated a loss of outer hair cells within the basal turn of the cochlea, decreased spiral ganglion neurons, and diminished afferent nerve synapses 1 week and 3 months after blast injury. These progressive effects are contrary to the traditional thinking that noise-induced hearing loss only occurs at the time of the injury. Furthermore, recent clinical data show that this is not just limited to the lab; noise-induced sensorineural hearing loss from military service accelerates what otherwise looks like normal hearing loss from aging. ,
Blasts may also cause temporary hearing threshold shifts without permanent hair cell damage, and yet there may be hearing damage despite this apparent audiometric recovery. This is because significant synaptic and neuronal loss may have occurred. Studies in chinchillas have revealed 20%–45% ribbon synapse loss in the mid and basal cochlea after blasts of 160–175 dB sound pressure levels. This phenomenon is called hidden hearing loss, and blast victims with hidden hearing loss often report hyperacusis and difficulty with speech-in-noise despite normal audiometric thresholds.
Timely audiometric evaluations are important to quantify and establish an objective verification of hearing loss. Unfortunately, audiometry often is not available in a combat theater, but treatment should not be delayed and should be initiated if there is a clinical suspicion of a sensorineural hearing loss. Even at Walter Reed Army Medical Center (2007–12), most initial audiograms were performed at the bedside and with background noise because it was impractical (or impossible) to transport polytrauma patients into a sound-proof booth. As usual, a Weber tuning fork exam is helpful to confirm a low-frequency sensorineural hearing loss, but one should be aware that it does not rule out higher-frequency hearing losses. Noise-induced hearing loss typically causes decreased high-frequency hearing sensitivity and an audiometric “noise” notch at 4000 Hz due to the transfer function of the ear. Blast-induced hearing losses not only affect these frequencies the most but also tend to involve the low- to mid-frequency thresholds with a flatter audiogram pattern. The presence of a tympanic membrane perforation should increase suspicion and empiric treatment of sensorineural hearing loss; a study conducted at the Walter Reed National Military Medical Center reported an incidence of sensorineural hearing loss of 36% in patients with blast-induced tympanic membrane perforations that required tympanoplasty. The strongest predictor of severe or profound hearing loss is the presence of ossicular chain discontinuity. Even when there is intact tympanic membrane, empiric steroid treatment should be considered if there is new-onset tinnitus or subjective hearing loss.
Steroids are the mainstay treatment for acute acoustic trauma. , Steroids may be otoprotective by scavenging oxygen free radicals and inhibiting lipid peroxidation. Animal studies have demonstrated a strong, acute otoprotective effect from topical steroids after impulse noise exposure or drill trauma. When sensorineural hearing loss is suspected, it is important to initiate high-dose oral steroids (e.g., 60 mg prednisone daily for 14 days, followed by a taper) or weekly intratympanic steroid injections (e.g., 0.4 mL of dexamethasone 10 mg/mL). This strategy mirrors the management of sudden sensorineural hearing loss. As such, American Academy of Otolaryngology—Head and Neck Surgery Clinical Practice Guideline recommends initiating steroids in patients with sudden sensorineural hearing loss within 2 weeks of symptom onset. Intratympanic steroid therapy can be offered to patients with incomplete recovery from sensorineural hearing loss 2–6 weeks after the onset of their symptoms. There is no evidence to suggest corticosteroid delivered intratympanically is more beneficial than systemic treatment in the case of moderate-to-severe idiopathic sudden sensorineural hearing loss, but this so far has not been studied for noise-induced hearing loss. Regardless, oral steroids may be more logistically feasible to administer in a combat environment. On the other hand, systemic steroids may hinder wound healing among polytrauma patients, so intratympanic steroids are sometimes a better option in a multidisciplinary setting.
Other compounds that have been studied in laboratory animals exposed to acoustic trauma include acetyl- l -carnitine and N -acetylcysteine (NAC) , 2,4-disulfonyl α-phenyl tertiary butyl nitrone, astragaloside IV, leupeptin ; Src-protein kinase inhibitors, AM-111, caroverine, and magnesium. However, none of these have come into common use, and at present, they should only be considered adjuncts to steroids rather than replacements.
Noise injury has been shown to increase calcium levels in hair cells and stimulate reactive oxygen species production, , resulting in the activation of cell death pathways. Accordingly, antioxidants have been studied as therapeutics to reduce reactive oxygen species levels in the hair cells after acoustic trauma. Eleven military officers who received oral NAC after an indoor shooting session received a small protective effect in hearing thresholds compared to controls. Unlike some of the other compounds, NAC is often available in military pharmacies because it is the antidote for acetaminophen overdose, and also a common respiratory therapy treatment. Given the low risk-to-benefit ratio of steroids and NAC, there should be a low threshold for initiation NAC with steroids after a blast injury.
Auditory hair cells may be more sensitive to further insults after a blast injury. Therefore, ear protection and minimization of hazardous noise exposure is critical. Any hearing loss should be followed up in the long term with annual audiograms and an otolaryngologist as needed. Many blast-injured patients require hearing aids, but there are cases that have ultimately required cochlear implants due to the severity of sensorineural hearing loss.
Hair cell repair is critical for auditory function. Thus, hair cell regeneration and viral gene therapy have been an active source of investigation for sensorineural hearing loss. In Zebrafish, epigenetic factors LSD1 and HDAC seem to be required for hair cell regeneration. , However, hair cells in mature mammals do not regenerate, and paths of regeneration may be different than from those of development. As a result, morphologic and transcriptome profiles may differ across species and maturity; there has been no effect of HDAC inhibitors on Atoh1-mediated conversion in the adult mouse cochleae. Posttranscriptional regulation (e.g., microRNA-induced gene silence) may play a role in hair cell development and regeneration, especially the miRNA 183 family , miRNA 124, and let7. Mizutari et al. found that introducing Notch inhibitor LY411575 in vivo in noise-damaged mouse cochleae activated Atoh1 and induced new hair cell formation with an 8-decibel hearing improvement. However, this finding was shown in neonatal mice and not in adult mammals. Combination therapies targeting multiple factors and epigenetic pathways hold promise for hair cell regeneration in mature mammalian cochleae following noise-induced sensorineural hearing loss.
Secondary effects of sensorineural hearing loss include tinnitus. Blast-induced tinnitus is common among veterans; approximately 49% of patients with blast-related injuries from war report tinnitus, as compared with a prevalence of 10%–15% within the normal population. The degree of severity can be influenced by stress, anxiety, and depression —which are common mental health disorders in the veteran and military population. , As a result, blast-exposed veterans may suffer greatly from the effects of tinnitus.
Currently, there is no cure or clinically recommended drug or device treatment for tinnitus. Sound-based therapy and cognitive behavioral therapy are mainstay management strategies to reduce the potentiation of tinnitus signal. Animal models have been widely used for pharmaceutical treatment research for tinnitus. Unfortunately, successful pharmacologic treatments in animal models (e.g., memantine, esketamine, AUT00063) have failed to share the same level of efficacy in humans. Studies adopting a bimodal neuromodulation approach toward tinnitus have reported a significant reduction in tinnitus symptom severity. Cochlear implants have also been shown to reduce tinnitus, particularly in those with a fluctuating type of tinnitus, greater tinnitus handicap, and shorter duration of tinnitus prior to implantation. In recent years, technological innovation has spurred the development of digital smart therapy platforms for personalized tinnitus modulation.
Vestibular injuries
Vestibular dysfunction is a frequent complaint among blast-exposed service members and civilians. , The most direct cause is labyrinthine concussion, which is thought to be acute damage to the vestibular organs from a pressure wave within the intralabyrinthine fluids. , Presenting symptoms are typical of an acute unilateral vestibulopathy and include vertigo, nausea, vomiting, and spontaneous nystagmus, all of which may be exacerbated with head movements. Murine studies have observed histological changes to the vestibular organs after blasts ; there was a significant loss of stereocilia in the cristae ampullaris and the macule after exposure to a 63-kPa peak blast wave (akin to an improved explosive device [IED] injury). As with any acute vestibular injury, these symptoms typically improve over weeks to months through vestibular compensation. Physical therapy may help blast-exposed patients with peripheral vestibular injuries, particularly older adults who may have difficulties with vestibular compensation due to age-related declines in neuroplasticity.
Explosions were the most common cause of traumatic brain injuries (TBIs) among military service members in the recent Middle East wars, accounting for approximately 80% of cases. Consequently, many patients with blast injuries have a mix of central and peripheral vestibular dysfunction, such as postconcussive syndrome combined with a labyrinthine injury. These can be difficult to separate from one another clinically although there are sometimes localizing signs. For example, a pure upbeat positional nystagmus cannot be explained by peripheral causes alone, but this is often seen (using videonystagmography goggles) after a mild TBI. Patients with pure upbeat positional nystagmus after a trauma therefore should be screened for TBI (assuming no nicotine, which also causes an upbeat nystagmus). This is also true for other central findings that may be seen during a bedside exam or during videonystagmography. These include pure torsional nystagmus, direction-changing nystagmus, poor fixation suppression of nystagmus, overshoots with saccades, and dysconjugate eye movements.
Posttraumatic headaches and postconcussive migraines are highly prevalent among this patient population. Even in cases of mild head trauma, disequilibrium was reported to persist for at least 2 or more years in approximately 18% of TBI patients. Any blast victim with symptoms of disequilibrium of moderate or severe intensity lasting 5 min to 72 h should be screened for the Bárány Society and International Headache Society’s diagnostic criteria for definitive or probable vestibular migraines. Vestibular migraine management often includes avoidance of triggers, dietary and behavioral modifications, sleep hygiene, over-the-counter supplementation, and/or antimigraine medications.
Benign paroxysmal positional vertigo (BPPV) is also common after ear and head traumas. , It is therefore important to evaluate any patient with dizziness after head trauma with Dix-Hallpike maneuvers, assuming no neck injuries (and then cervical vertigo is another possibility). Personal experience suggests Dix-Hallpike maneuvers should be performed even if the symptoms do not sound like classic BPPV, as it is not unusual to see a positive response (with the typical upbeat and geotropic torsional nystagmus) in trauma patients with atypical symptoms.
Patients that report autophony after blast exposure should undergo a temporal bone CT scan and vestibular evoked myogenic potential testing to evaluate for superior semicircular canal dehiscence syndrome. It is not unusual for superior semicircular canal dehiscence to become symptomatic after head traumas. For example, there is a case report on a 26-year-old service member who was near two IED blasts during his deployment, and then had new onset of oscillopsia and vertigo in response to loud and low-pitch sounds. The hypothesized etiology was pressure-induced mobilization of (presumably thin) bone overlaying the superior semicircular canal. Surgical plugging of such a dehiscence is no different than with nontrauma cases, but the perioperative management may be more complicated by comorbidities such as TBI and migraine.
Perilymphatic fistula is another cause of hearing loss and vestibular dysfunction. However, the authors have only one surgically confirmed case (and several negative explorations) from the Walter Reed and Boston Marathon bombing experiences, so this is possible but seems to be uncommon. Many of these patients require prolonged bedrest, so perhaps breaches of the otic capsule heal without causing subsequent symptoms.
Vestibular screening should be conducted even if a blast-exposed individual appears relatively unscathed. For example, a study evaluating 100 patients after blast injury or exposure demonstrated that individuals without severe extremity injuries were more likely to suffer from imbalance and vertigo than those with severe extremity injuries. However, this is in part explained by the higher prevalence of TBI in the latter group. Also, symptoms often take time to manifest and may go unnoticed while these patients are confined to hospital beds or light activities. Furthermore, autonomic deconditioning is another consequence of prolonged bedrest, so it is common for these patients to be lightheaded with standing or incur cervicogenic dizziness once they start to resume more routine activities. This can result in referrals for suspected vestibular dysfunction. It fortunately tends to resolve spontaneously, but this can take time.
Conclusion
Otologic injuries are common after blast trauma. Acute management of otologic injuries among the military personnel and civilians is influenced by the combat terrain and proximity to facilities with surgical capabilities and specialty care. Blast-exposed patients are likely to suffer from long-term effects of hearing loss, tinnitus, tympanic membrane perforations, and dizziness—and should be followed by an otolaryngologist for chronic care and management.
References
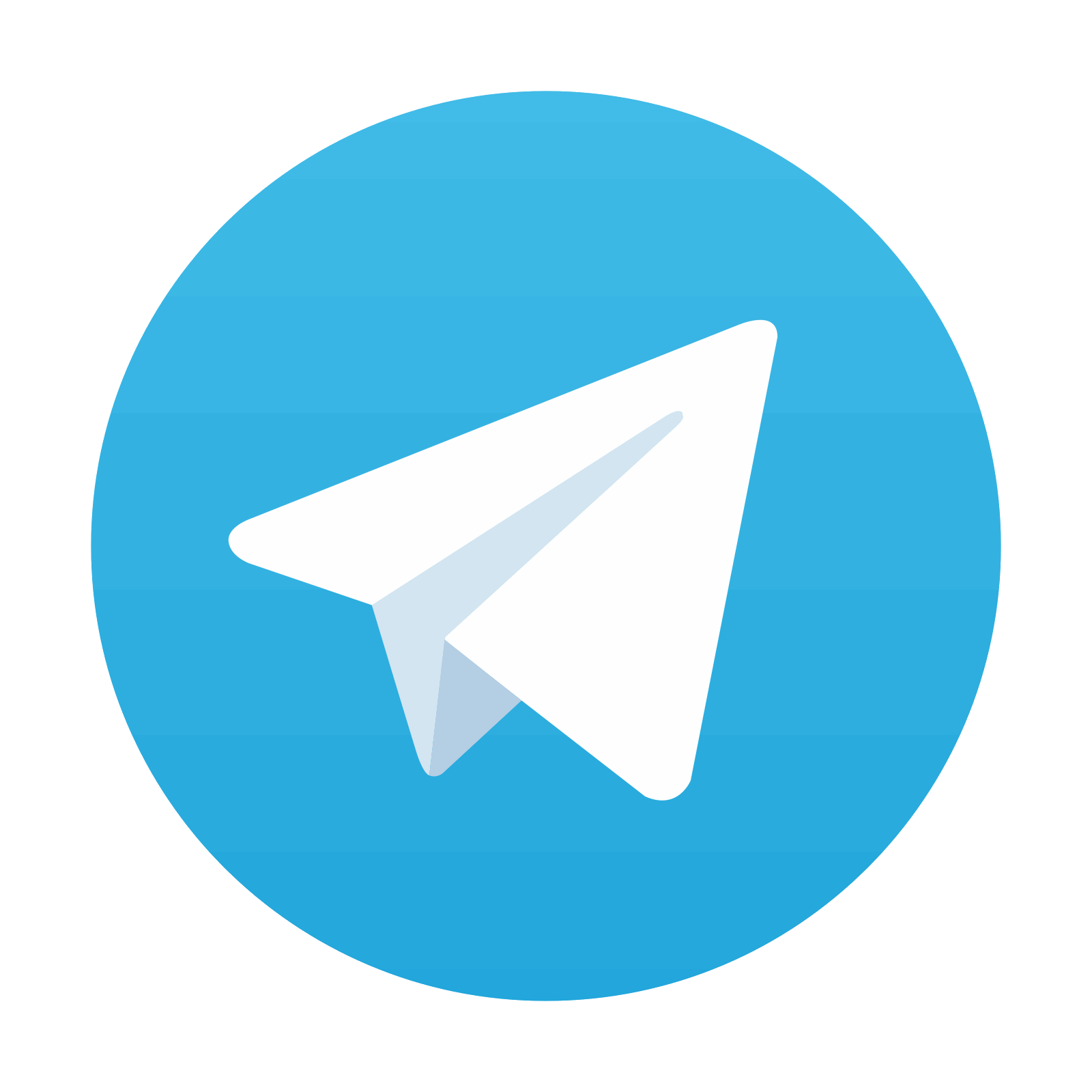
Stay updated, free articles. Join our Telegram channel

Full access? Get Clinical Tree
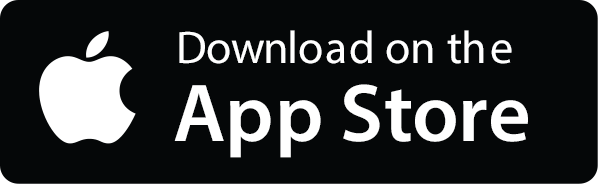
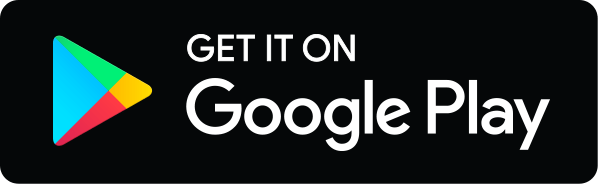
