Summary
Anterior segment optical coherence tomography provides noninvasive, high-resolution, cross-sectional images of anterior segment structures and serves as an effective adjuvant tool in the diagnosis and management of glaucomatous conditions, particularly primary angle closure disease.
Key words
optical coherence tomography – anterior segment – primary angle closure suspect – primary angle closure glaucoma – plateau iris9 Anterior Segment Optical Coherence Tomography in Glaucoma
9.1 Introduction to Anterior-Segment OCT
Optical coherence tomography (OCT) is a powerful diagnostic technology which produces high-resolution cross-sectional images of the internal microstructure of living tissues, first described in the posterior and anterior segments of the eye in the 1990s. 1 Prior to this technology, the main imaging modality for evaluating the anterior segment of the eye was ultrasound biomicroscopy (UBM). Imaging of the iridocorneal angle and anterior segment anatomy is of particular interest to those diagnosing and managing different forms of glaucoma. UBM use frequencies from 35 to 80 MHz while OCT uses low-coherence, near-infrared light, allowing for very high resolution images. Apart from the increased resolution of anterior segment OCT (AS-OCT), it also has the advantage of significant ease in obtaining images. AS-OCT is noncontact and can be performed with patients sitting upright, compared to UBM which requires an eyecup, coupling medium with direct contact to the eye, and supine positioning. Anatomically, UBM confers the advantage of being able to image structures posterior to the iris, which is a limitation of AS-OCT imaging. A direct comparison between AS-OCT and UBM found no significant differences in anterior chamber parameters measured with both devices and a more easily identifiable scleral spur with AS-OCT compared to UBM. 2
OCT technology was first described in 1991, 1 and though simplified, has been described as the optical equivalent of ultrasound imaging. Light is projected onto a biologic structure, in this case the eye, and the system detects reflected, nonscattered, coherent light. The two categories are time-domain OCT and the newer Fourier-domain, or spectral-domain, OCT. The former measures time-of-flight delay from light reflected off the tissue to that reflected from an adjustable, moving reference mirror. The latter uses a spectrometer to detect differences in light reflection between the tissue and a fixed reference mirror, using mathematical transformations to confer information about depth. Due to these differences, spectral-domain OCT provides higher image resolution and faster image acquisition. Swept-source OCT is an iteration of spectral-domain OCT and can be used for the anterior segment.
Image acquisition is similar to posterior segment imaging in that the patient is upright and no topical anesthesia is required. A wide palpebral fissure is required to fully image the anterior segment, and closed eyelids can affect image quality, particularly in the vertical meridian. This can be alleviated by manually opening the eyelids or using an eyelid speculum. The eye is aligned, the distance refraction is entered, and the patient is asked to focus on the internal fixation target at which point the images are obtained. Once images are acquired, geometric computational processes convert the optical distances from the images to physical distances taking refraction index variations from air, cornea, and aqueous humor into account. Since its initial application to the anterior segment in 1991, numerous advances have been made to AS-OCT.
9.2 Different AS-OCT Modalities and Systems
Compared to posterior segment OCT, AS-OCT typically uses a longer wavelength (1,310 nm compared to 830 nm). This longer wavelength further decreases scan acquisition time as well as decreases posterior segment exposure. This also allows improved penetration through structures that highly scatter light including the scleral and limbus.
Table 9‑1 is a list of specifications of historical and currently available AS-OCT imaging systems, a few of which are discussed in more depth below. 3
Visante, the first AS-OCT imaging system to employ a higher wavelength, provided higher resolution than its predecessors but not enough to accurately determine the location of the trabecular meshwork. For this reason, areas of angle closure are determined by contact between the iris and the angle anterior to the scleral spur, thus overestimating angle closure when compared to gonioscopy. Also, due to its time-domain technology, scan acquisition time is significantly longer than its spectral-domain equivalents. The slit lamp OCT was unique in its integration into the slit lamp biomicroscope, though it had the same time-domain related constraints and there was poor agreement between that and the Visante. 4
An update to spectral-domain OCT in the Cirrus, Spectralis, and CASIA systems was associated with increased scan quality and decreased scan acquisition time with improved visualization of the anterior chamber angle and the ability to image smaller structures including the trabecular meshwork, scleral spur, and Schwalbe’s line. The CASIA further has the advantage of being able to obtain a 360-degree image of the anterior chamber using 128 cross-sections obtained in less than 3 seconds. Increasing image quality has contributed to a growing body of literature identifying and studying specific structures and parameters.
9.3 AS-OCT Identification of Anterior Segment Structures and Parameters
Many different angle parameters have been described to standardize the measurements obtained for clinical and research purposes, though longitudinal studies are needed to validate the diagnostic significance of these parameters. 5 Compared to UBM, there is a high level of correlation between anterior chamber angle measurements taken with UBM or older AS-OCT, though UBM angle parameters have been reported to be smaller, potentially due to pressure from the eyecup during UBM image acquisition.
Generally, AS-OCT measurements can be obtained with excellent repeatability and reproducibility with regard to angle parameters. With regards to image segmentation and quantification, there is still quite a bit of variability, and automated algorithms are needed. The Zhongshan Angle Assessment Program provided a semiautomatic algorithm to calculate various parameters, though this, like other measurements of angle parameters, is based on the ability to manually identify the scleral spur and the angle recess. A proposed method for automatic AS-OCT structure segmentation, measurement, and screening is promising, but not currently commercially available.
Identification of the scleral spur may vary among different graders and lead to differences in manual and semiautomatic measurements of the same angle parameters. In most situations, the system-generated automatic marking of these two landmarks is not accurate and needs manual correction. Cues including high pixel reflectivity or a subtle change in the contour of the inner scleral margin can help identify the scleral spur on AS-OCT images, though poor image quality or anatomically narrow angles can affect these and prevent accurate identification of the scleral spur. 6 A study using the Visante reported being unable to identify the scleral spur in as high as 25% of scans. 6 A similar study using Visante found similar rates of scleral spur identification, though were able to further stratify that the rates of identification were even lower in the superior and inferior quadrants. 7 Table 9‑2 is a list of commonly measured and reported anterior segment parameters (Fig. 9‑1).

9.4 AS-OCT in Different Glaucomatous Conditions
AS-OCT is most helpful for patients with glaucoma with its objective qualitative and quantitative assessment of the anterior chamber angle. This is important in many conditions, but most revelatory in primary angle closure disease (PACD), a spectrum of conditions which includes acute primary angle closure, primary angle closure, primary angle closure suspect (PACS), and primary angle closure glaucoma (PACG). In these conditions, there is apposition between the peripheral cornea and the iris, blocking the trabecular meshwork and causing a narrow drainage angle. Gonioscopy remains the gold standard for diagnosing and following these conditions, but AS-OCT can serve as a useful adjunct. Although anterior segment imaging provides useful information in assessing PACD, it should not be considered a substitute for gonioscopy. 5
9.4.1 Primary Angle Closure Suspect (PACS) (Fig. 9‑2)
PACS is defined as at least 180 degrees of iridotrabecular contact with nonvisualization of the posterior trabecular meshwork on gonioscopy, absence of peripheral anterior synechiae (PAS), intraocular pressure (IOP) ≤21 mmHg, and absence of glaucomatous optic neuropathy. Iris area and iris volume are AS-OCT metrics which have been described as a potential differentiator between PACS and PACG, with patients with PACG having thicker irides. Lens vault (LV) is a similar metric seen more in PACG compared to PACS, representing anterior displacement of the lens, a surrogate for iridolenticular contact leading to angle crowding. There is also growing interest in using deep learning as a tool to screen for and diagnose patients with PACD and has promising implications for future diagnostics.

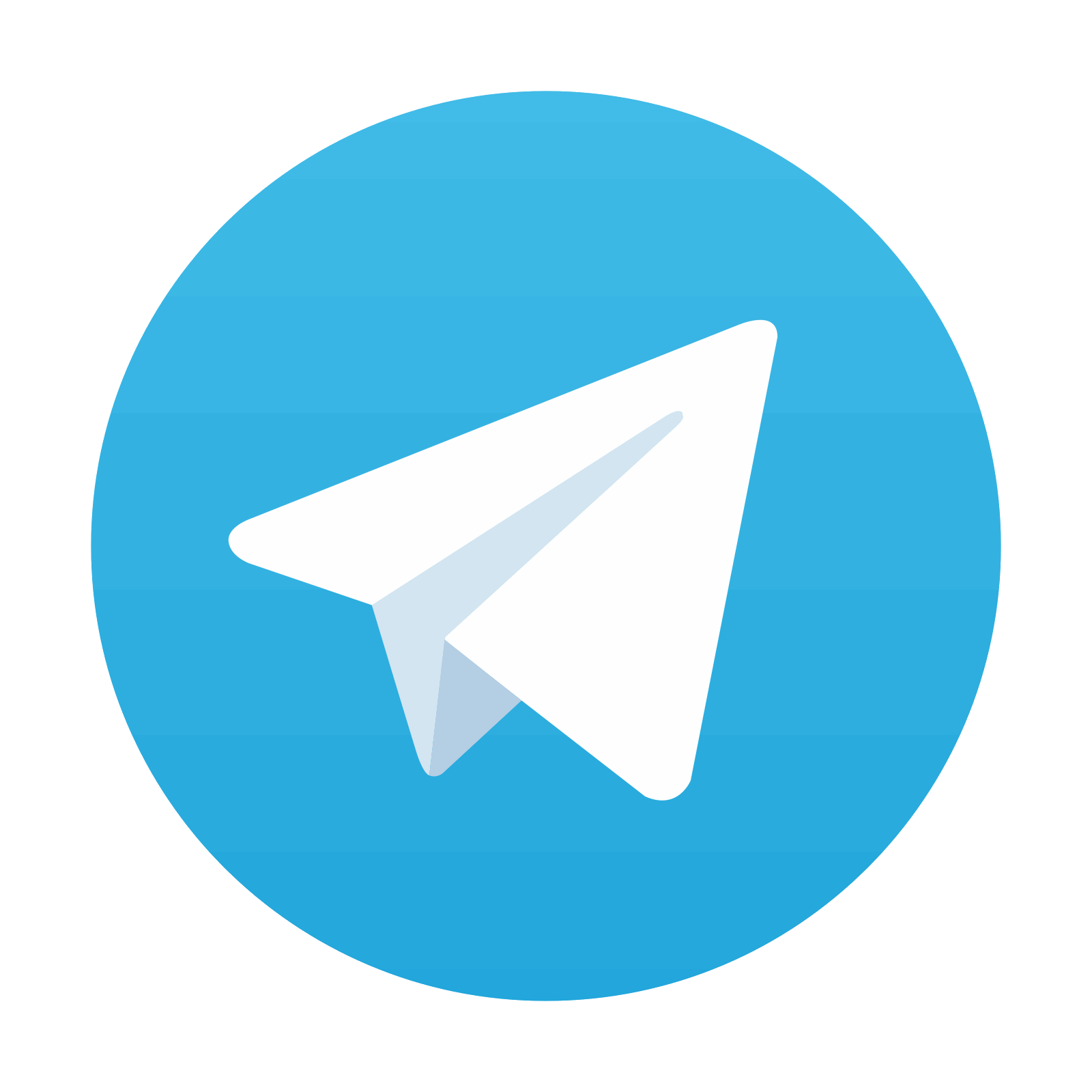
Stay updated, free articles. Join our Telegram channel

Full access? Get Clinical Tree
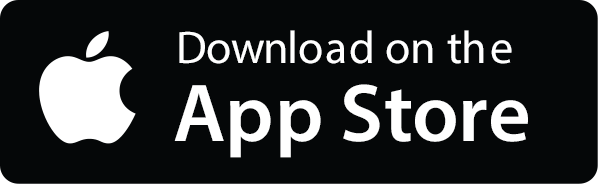
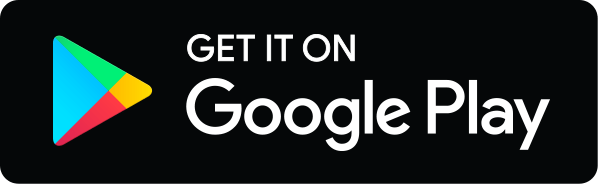
