Summary
Retinal imaging for glaucoma diagnosis and management has largely focused on quantifying characteristics of the optic nerve head (ONH), the peripapillary retinal nerve fiber layer (RNFL), and the retinal ganglion cell complex (GCC); this chapter focuses on optical coherence tomography (OCT) of the macula in glaucoma patients. These measurements are followed longitudinally to assess for changes due to glaucomatous progression. OCT measurements of the peripapillary RNFL and/or GCC may be affected by image acquisition techniques, image artifacts, and patient characteristics—all of which can limit the utility of peripapillary imaging or ONH imaging in glaucoma management. OCT imaging of macula retinal ganglion cells (RGCs) can supplement the information obtained with peripapillary imaging and may offer some advantages, including less variation in the RGC measurement and better image segmentation. Segmentation and interpretation of macular imaging may be influenced by artifacts that are different from those that affect peripapillary images. Further, certain populations of patients may have improved glaucoma surveillance using macular OCT scans such as patients with advanced glaucoma or myopia. Thus, macular imaging may complement the use of ONH or RNFL imaging and increase confidence of OCT findings. In this chapter, we discuss the applications, advantages and disadvantages, and potential pitfalls of OCT imaging of the macula for glaucoma diagnosis, management, and longitudinal follow-up.
Key words
optical coherence tomography – macula – glaucoma – retinal nerve fiber layer imaging – retinal ganglion cell – glaucoma progression – imaging artifact4 Optical Coherence Tomography of the Macula
4.1 Retinal Imaging for Glaucoma
4.1.1 Glaucoma and Retinal Ganglion Cells
Ultimately, glaucoma is a collection of diseases that involve retinal ganglion cells (RGCs) dysfunction and/or loss. In patients with glaucoma, the structural changes of the optic nerve head (ONH) over time are due to RGC loss. Cell bodies of RGCs are found in the ganglion cell layer (GCL) of the retina, dendrites of RGCs are in the inner plexiform layer (IPL), and axons in the retinal nerve fiber layer (RNFL); RGC axons exit the eye through the optic nerve to synapse in the ipsilateral or contralateral lateral geniculate nucleus depending on where in the retina they originate. These three layers together (RNFL + GCL + IPL) are referred to as the ganglion cell complex (GCC).
However, glaucoma is not the only condition that affects the structure of the ONH or the peripapillary inner retina. Other findings include edema, pallor, and peripapillary abnormalities. ONH edema can occur in a variety of conditions; examples include elevated intracranial pressure, infection, inflammation, and acute ischemia. ONH edema leads to swelling of the RNFL, obliteration of the optic cup, and may be accompanied with retinal hemorrhages, cotton wool spots, or pallor. Optic disc pallor is another ONH finding that is not typical of glaucoma; this is more commonly seen in other optic neuropathies. ONH drusen may also change the contour of the optic nerve and mimic some of the visual field deficits seen in glaucoma and also affect peripapillary RNFL. Peripapillary atrophy around the optic nerve may be seen in variety of conditions including age-related macular degeneration, (pathologic) myopia, or in normal patients without other ocular comorbidities. Choroidal neovascular membranes can be seen juxtapapillary and may be a source of peripapillary RNFL thickening.
Similarly, GCC loss may also occur due to nonglaucomatous optic neuropathies or secondary to retrograde transneuronal degeneration, for example, after cerebrovascular accident or with an intracranial tumor (Fig. 4‑1). In glaucomatous optic neuropathy, however, there may be other clinical signs—optic nerve cupping, RNFL loss that respect the horizontal midline, optic disc hemorrhages, characteristic visual field changes on automated perimetry, etc.—that aid in the diagnosis of glaucoma.

Detecting structural changes in RGC layers is particularly important in glaucoma care as these changes may be the earliest clinical signs leading to a diagnosis of glaucoma. They may also be the first signs to confirm glaucomatous progression in patients with known glaucomatous optic neuropathy. Significant structural changes (of up to 25–35% loss) can occur prior to visual field changes seen on automated perimety. 1 Visual field defects may occur years after optic nerve or RNFL changes. Clinically, structural changes at the ONH or defects of the RNFL can be seen by slit lamp ophthalmoscopy or direct ophthalmoscopy or comparison of optic disc photography over time. These findings include vertical elongation of the optic cup, enlarged optic cup, optic rim notching, acquired optic pit, and wedge defects of the RNFL (which are more easily visualized using a green light or “red-free” light). Retina imaging is capable of detecting more subtle or smaller structural changes throughout the posterior pole which are challenging or impossible to see by clinical ophthalmoscopy alone.
Noninvasive imaging of the peripapillary and macula RGCs can be accomplished by a variety of techniques, including scanning laser ophthalmoscopy (SLO), scanning laser polarimetry, and optical coherence tomography (OCT). Retinal thickness analyzers have been used to measure total macular thickness. In this chapter, we will discuss the uses of spectral domain OCT (SD-OCT). SD-OCT is the most widely used commercially available device type for imaging retinal tissue. SD-OCT offers superior resolution and more efficient image acquisition relative to its predecessor time-domain OCT. SD-OCT is capable of imaging the macula, peripapillary region, and the ONH. There are many research and clinical applications of SD-OCT technology described in the literature for patients with glaucoma.
4.1.2 RGCs and the Macula
Although the peripapillary region has been the area that glaucoma specialists initially focused on when evaluating for glaucomatous disease, the macula has become an important structure to image for both the diagnosis and management of glaucoma. Unlike the peripapillary region, glaucomatous changes of the macula are not detected on ophthalmoscopy the way that some structural changes of the ONH/peripapillary RNFL can be seen on both OCT and ophthalmoscopy. The glaucomatous changes in the macula are more subtle and diffuse, less focal, than closer to the optic nerve, as the RNFL spans a greater area in the macula than it does around the optic nerve. The use of imaging of the macula in patients with glaucoma is of greater importance since the clinical signs of glaucoma disease are not able to be seen by fundus ophthalmoscopy.
In the macula RGCs are plentiful, with roughly 50% of RGCs localizing to the anatomic macula; the span of RGCs constitutes over 30% of total retinal thickness. 2 Cell bodies of RGCs are greater than one cell thick in the macula. This means that RGC death in the macula leads to significant and measurable changes of the RNFL, GCC, and the total macular thickness on retinal imaging. This is an important advantage over peripapillary imaging, where changes in thickness over time are most sensitive to losses of RNFL (axons) and not RGC bodies.
Further, RGC loss in the macula intuitively correlates with visual field loss (on automated perimetry) than peripapillary RGC loss with visual field loss. The most common visual field testing locations are testing visual function in the macula surrounding the fovea (at the patient’s fixation). Mapping RNFL loss around the peripapillary region to visual field losses involves following RGC axons from the optic nerve to their origination in the macula. Central defects seen on central visual field testing are more easily identified when looking at macular OCT imaging as opposed to peripapillary RNFL OCT images since the locations of these defects reside near the fovea (Fig. 4‑2).

Use of macular OCT marks a transition of how imaging for glaucoma is performed; initially, documenting and supplementing the clinical examination, to currently, providing structural information previously unattainable from clinical examination alone. ONH and peripapillary imaging was performed as the mainstay of glaucoma imaging and was primarily used to highlight areas of loss and supplement optic nerve ophthalmoscopy. Macular OCT can be used to complement peripapillary imaging and may offer internal validation of changes seen on peripapillary imaging. When an OCT finding is seen on macula or RNFL peripapillary imaging and is able to be correlated with the other OCT imaging location, the user can have more confidence that the finding is “real” and not the result of an artifact. Macular OCT may also allow for the possibility of detecting changes early, prior to visual field loss. The inner layers of the macula can show significant thinning prior to visual field deficits on perimetry.
There are some limitations to implementation. OCT scanning of the macula will add time to imaging acquisition protocols depending on the protocol and OCT machine manufacturer. Similar to RNFL protocols, macular imaging requires patient cooperation and fixation. Macula images may be susceptible to a variety of artifacts that can be related to patient characteristics, glaucomatous related changes, and other ocular comorbidities. In some cases, artifacts seen on the macula scan are not seen on the peripapillary scan and vice versa. Common artifacts seen on macula imaging are discussed later in this chapter.
4.2 OCT Image of the Macula
4.2.1 Devices and Segmentation
Currently, there are five major manufacturers of commercially available SD-OCT devices: (1) Cirrus from Carl Zeiss Meditec (Jena, Germany); (2) Spectralis from Heidelberg Engineering (Heidelberg, Germany); (3) 3D OCT-2000 from Topcon Medical Systems (Tokyo, Japan); (4) Avanti Widefield OCT from Optovue (Fremont, CA, USA); and (5) RS-3000 Advance 2 from Nidek (Gamagori, Japan). Three of these devices acquire raster scans of the macula in a square pattern (Spectralis, RS-300 Advance, 3D OCT-2000) and two acquire an ellipse or circle pattern of the macula (Cirrus, Avanti). The manufacturers report results as either total retinal thickness and/or a combination of RNFL, GCL, and IPL. There is no standard as to which size or shape is best for discriminating glaucomatous changes in the macula. Users should be aware of different shapes and sizes that are available for imaging the macula using SD-OCT.
To quantify RNFL, GCC, or total macular thickness measurements, automated segmentation software is used. Some manufacturers allow for the user to manually correct individual scan segmentation errors using their software. Segmented macula layers can discriminate glaucomatous and healthy control eyes. 3 Total macular thickness measurements are reproducible and segmented by OCT software. Total macular thickness may be more accurately segmented when compared with RNFL or GCC layers, due to the high reflectivity of the internal and external limiting membranes. However, total macular thickness measurement can be affected by nonglaucomatous pathologies, and may be influenced to a greater amount, compared to RNFL/GCC measurements, by pathologies that affect retinal thickness outside of the GCC. GCC segmentation may improve diagnostic ability of macular scans, and some studies have found improved diagnostic ability of macular GCC over total macular thickness. 4 GCC measurements are also highly repeatable by commercially available machines. The ratio between GCC and total macular thickness may also be an important measure in early glaucoma. Artifacts influence segmentation of the macula in ways that may differ from peripapillary RNFL scans; this depends on the source and location of the artifact and is discussed later in this chapter.
4.2.2 Correlation between Visual Field and Macular OCT
The function of the RGCs in the macula can be tested by automated perimetry—more so in center-weighted visual field tests, such as the Humphrey Visual Field (HVF) 10–2 pattern. This structure–function relationship is keenly important for the diagnosis of glaucoma. In the most common pattern of standard automated perimetry, the HVF 24–2 with a size III stimulus, nearly 230 ganglion cells are tested by a stimulus that excites a point near the fovea, and 10 ganglion cells are stimulated by a stimulus on an edge point. 5 The 24–2 pattern vastly under samples the center macula region; the center of the macula is the region with greatest range of RGC thickness. It is notable that estimates correlating structure and function have determined that a 52% loss of RGCs correlates with a 3 dB loss on the visual field. 5 This highlights the importance of using OCT for diagnosis of preperimetric disease glaucoma.
Pathologies of the RGCs in the macula inferior to the fovea will affect the superior visual field and pathologies of the superior macula will affect the inferior visual field. In normal eyes, perifoveal macular thickness is correlated with visual field sensitivity on automated perimetry. 6 The mapping of macular structure to visual field is easier to visualize than the relationship between peripapillary RNFL and visual field. However, techniques to combine visual field and OCT data into combined probability maps will likely continue to help doctors understand the structure–function relationship in their patients with glaucoma. 7 These techniques will also be invaluable to patients to understand their condition and results of their visual field and retinal OCT testing.
Imaging around the optic disc does offer some advantages over imaging of the macula using OCT. Both acquisition locations, the RNFL and the macula, are known to correlate with glaucoma severity. Earlier studies found greater ability to distinguish glaucomatous and normal eyes using ONH or peripapillary OCT protocols compared to macular imaging in patients with glaucoma and normal tension glaucoma. 8 , 9 Visual field abnormalities also correlated better with peripapillary RNFL changes rather than macular OCT scan parameters. 10
Changes in macula thickness do correlate with structural optic nerve changes, peripapillary RNFL thickness, and visual field. Performance of segmented macular volume and macular layers—macula nerve fiber layer (mNFL) or macular GCC—to distinguish healthy, glaucoma suspect, and glaucoma subjects (with different stages of disease) has been shown to be similar to peripapillary RNFL. 11 Macular scans can also be used to distinguish early from advanced glaucomatous disease. The relationship between visual field and OCT in different stages of glaucoma were similar whether they were evaluated by macular scans or peripapillary RNFL. 12 Macular thickness is also known to correlate with hemifield loss respecting the horizontal midline on visual field. This is similar to RNFL OCT changes and their relationship to visual field tests. Of note, the topographical relationship between OCT of the macula and visual field, and the comparison of probability maps of automated perimetry and OCT of the macula, are more direct and intuitive to the interpreter.
4.2.3 Complementing Peripapillary RNFL Scans with Macular OCT Scans
RGC death will lead to a decrease in RNFL thickness on retinal imaging. Thus changes in RGC macular thickness will also correlate with RNFL measurements on OCT (both macular and peripapillary) scans. This complementary nature of macular OCT to ONH or peripapillary OCT parameters is one of the greatest strengths of macular imaging.
Glaucomatous defects respect the horizontal midline (or the line that connects the fovea to the center of the ONH) and follow the RNFL bundles in an arcuate curvilinear path from macula RGC back to the optic nerve. Importantly, this pattern of loss can help differentiate glaucomatous changes from retina thickness measurement changes from other pathologies; examples include vascular occlusion, ischemic optic neuropathies, and macular degeneration. Macular scans are also easy and require minimal additional time to acquire in patients who are already having imaging around the optic nerve. Dilation is not required in most patients. The relationship between macula thickness and GCC also varies with disease stage.
Artifacts that affect the optic nerve and peripapillary are usually different from those that affect the macula. This allows the macula scans to complement the RNFL OCT, especially when peripapillary artifacts limit interpretation of peripapillary scans or when peripapillary OCT scans are uninterpretable, such as with myopic changes. Importantly, studies have found that RNFL analysis around the ONH can miss macular damage (seen on macular OCT, or 10–2 pattern visual field), which could represent early damage or progression of glaucoma; up to one out of three OCT reports from peripapillary RNFL can miss macular damage. 13 This highlights the importance of using macular scans, especially in patients who are not routinely undergoing a visual field testing dedicated to the central macula. Patients with low or normal tension glaucoma in particular may present with central defects. These defects can be easily missed or overlooked on traditional 24–2 strategy visual field testing and may not easily be seen on RNFL OCT reports. Other types of glaucoma may also have central visual field defects missed on traditional visual field testing strategies and may benefit from macular OCT scans.
Another consideration is that RNFL reports are typically presented as sectoral maps. This can lead to pathologic focal defects from glaucoma being “averaged” out in a sector or global indices. Sometimes this is coined “green” disease as reports show sectoral data in the color green to indicate that they are normal when analyzed using data from a normative database, when in fact there is focal RNFL loss that is pathologic. The macula SD-OCT reports are typically not presented in this manner for scans done using a glaucoma protocol for image acquisition and analysis; they are presented without sectoral or global summary indices. This allows the end user to see glaucomatous patterns (such as arcuate or paracentral) of loss more easily. Careful analysis of the data in OCT raw scans is still needed to ensure these subtle (yet significant) changes are not overlooked.
4.2.4 Asymmetry Analysis
In the HVF test, one of the parameters reported is the glaucoma hemifield test, which compares sets of data points across the horizontal midline for asymmetry. In this same spirit, asymmetry reports of macular OCT data allow for comparison across the horizontal midline (intra-eye comparison) and also comparison between eyes (inter-eye comparison). In this case the comparison across the horizontal midline is done across the line that connects the fovea to the center of the ONH. Since glaucoma is a disease characterized by asymmetry (both between eyes and within an eye), these plots can highlight early or mild-to-moderate changes that may not be as easily seen on peripapillary RNFL sectoral maps. Quadrant analysis of macular asymmetry also correlates to functional declines in visual field sensitivity. 14
Intra-eye comparison often tends to be more helpful than inter-eye comparison. Since glaucomatous changes respect the horizontal midline, early signs can be seen as asymmetry on these hemifield comparisons. They may appear in arcuate patterns in patients with glaucoma. These intra-eye comparisons are more helpful than comparing to a normative database.
4.3 Macular OCT and Glaucoma Management
4.3.1 Diagnosis and Progression
For the diagnosis of glaucoma, OCT of macula can be a useful tool. The doctor looks for the characteristic OCT patterns that correlate with the typical “visual field type” patterns seen in glaucoma, that is, assessing OCT scans of the macula for arcuate or paracentral RNFL/total macular thickness losses (relative to the other hemifield or to the other eye) or overall loss of RNFL volume. These losses will also respect the line that connects the fovea to the center of the optic nerve which is the anatomic equivalent to the horizontal midline on the visual field. In the same way that visual field deficits typically “point” toward the optic nerve, one may observe “grooves” or losses of the RNFL as a bundle can be followed back to the optic nerve. The contour of the overlying retinal vessels can be affected and are seen on three-dimensional reconstructions of images to follow the contour of these “grooves.” These findings are similar to the RNFL wedge defects seen as loss of retinal sheen on retinal photos or on ophthalmoscopy.
Paracentral defects can be seen and can be one of the most helpful uses of the OCT macula map (Fig. 4‑2). The topographic map created in this central annulus of the macula shows deep paracentral defects in what is usually the thickest portion of the macula. Thus, it can be easier to detect a deficit that may otherwise be averaged out on the RNFL plot. Altitudinal RNFL defects of the macula can also be seen in more advanced glaucoma, but care should be taken to consider nonglaucomatous pathologies such as prior vascular occlusion or ischemic optic neuropathy. Similarly, in cases with patterns of loss that do not respect the fovea to optic nerve line (the RNFL raphe) or involve only the temporal edge of the macula map, nonglaucomatous pathologies should be considered.
Comparison of OCT maps over time can aid in the diagnosis of glaucomatous progression. Subtraction maps of OCT macula scans can highlight arcuate-shaped or paracentral defects that deepen over time, consistent with progression of disease. These defects can often be tracked back to the optic nerve. Also, corresponding defects can be seen on OCT peripapillary RNFL change plots or RNFL raw scans for confirmation. Macular scans have been shown to outperform peripapillary RNFL when it comes to detecting progression. 15 They may also hold an advantage in patients with advanced glaucoma, as the RNFL may have limited thickness to detect change, while the macula, and its greater population of RGCs, may be in a more dynamic range.
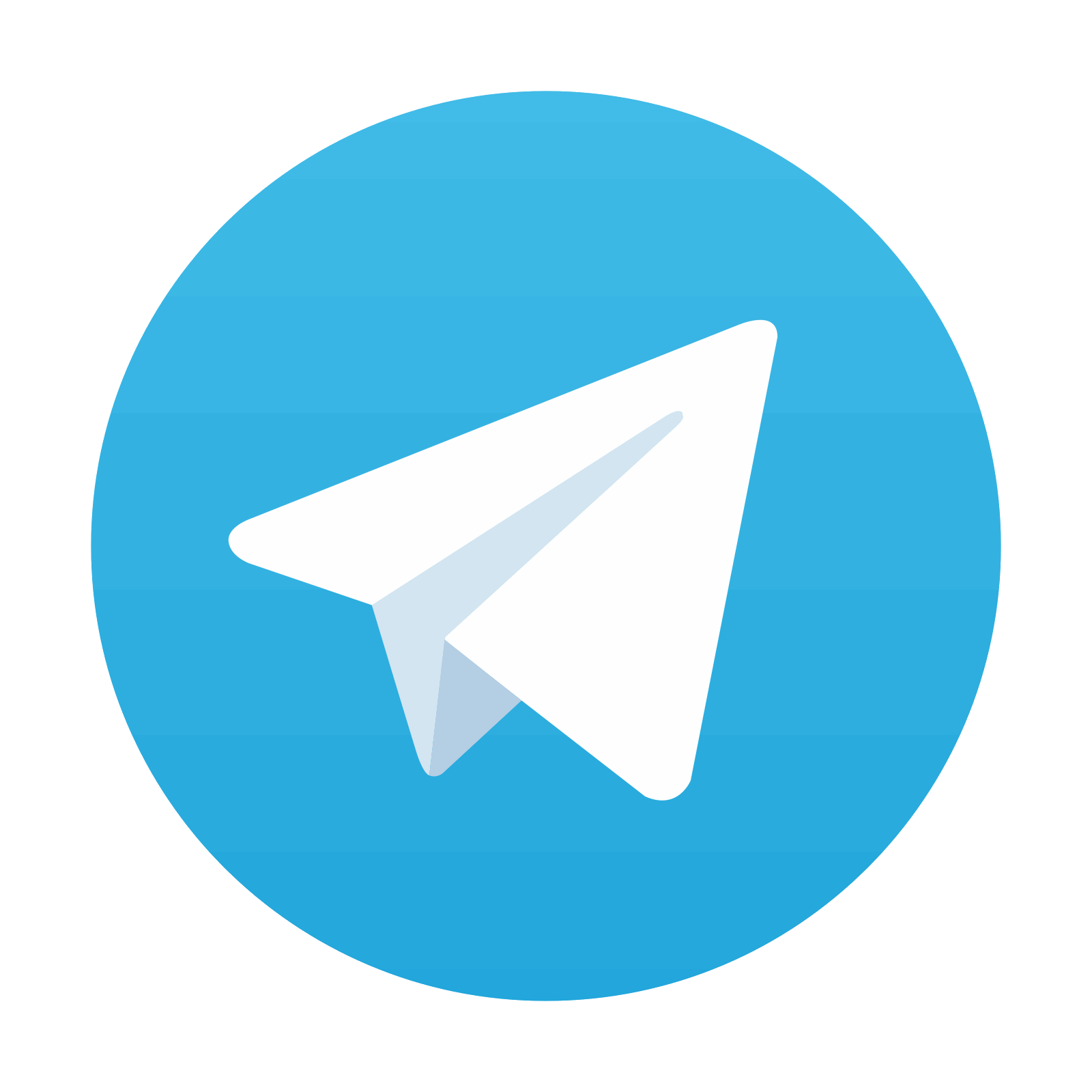
Stay updated, free articles. Join our Telegram channel

Full access? Get Clinical Tree
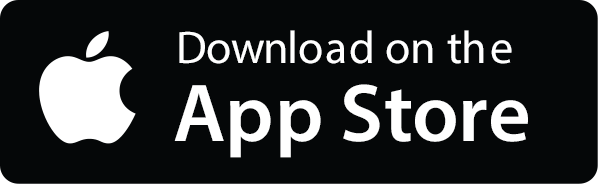
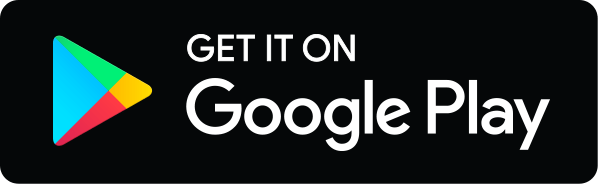
