Ocular Angiogenesis
Sampathkumar Rangasamy • Alexander Ljubimov • Sayon Roy • Paul McGuire • Arup Das
Ocular angiogenesis involves two important key steps: endothelial cell proliferation and migration. Hypoxia upregulates growth factors most of which cause cell proliferation. Increased growth factors also cause increased expression of proteinases and integrins that are important for cell migration. The proteinases facilitate the breakdown of the basement membrane and extracellular matrix (ECM), allowing cells to migrate. This chapter focuses on the structure and function of the basement membrane first, then the roles of proteinases and integrins in ocular angiogenesis, and how inhibitors of these molecules can be utilized to inhibit angiogenesis in the eye.
Basement Membrane Components: Structure and Function
Basement membranes are complex, multicomponent structures with diverse functions. Studies investigating ultrastructural changes in diabetic retinopathy (DR) have established thickened vascular basement membrane as one of the earliest structural abnormalities. Evidence suggests that this fundamental structural lesion develops, at least in part, from excess accumulation of basement membrane material. Increased syntheses of several vascular basement membrane components have been identified in diabetes. While the composition of basement membranes is still incompletely known, some of the major components, including fibronectin, collagen type IV, and laminin,1 are known (Table 7.1). These components attach to each other in a highly organized manner and form a continuous sheet beneath the endothelial cell layer. The sheet-like membranous structure provides support for attachment, spreading, migration, growth, repair, and differentiation of the overlying cells.2–4
Table 7.1.
ECM components in basement membrane
ECM Components | Effect of Diabetes or High Glucose (References) |
---|---|
Fibronectin | Increased (5–13) |
Laminin | Increased (14–16) |
Vitronectin | Increased (14,17,18) |
Entactin | Unknown (19) |
Bamin | Unknown (20) |
Dystroglycan | Unknown (21) |
Collagen I | Increased (13) |
Collagen II | Unknown (22,23) |
Collagen III | Increased (6,13) |
Collagen IV | Increased (5,15,16,24–28) |
Collagen V | Increased (13) |
Collagen VI | Increased (14,29–31) |
Elastin | Unknown (32) |
Tenascin | Increased (7,11,13) |
Chondroitin sulfate | Increased (33) |
Hyaluronic acid | Increased (34) |
Heparan sulfate | Increased (35) |
Decorin | Increased (8,36) |
Biglycan | Increased (8,37) |
Fibronectin is a glycoprotein that binds to integrins, collagen, fibrin, and heparan sulfate proteoglycans. It is a critical component of the basement membrane and is upregulated in diabetes. It consists of two similar monomers that are linked by disulfide bonds at their c-termini.38 Considered as molecular glue, it facilitates the attachment of basement membrane components into an organized scaffold. Collagen type IV is another major component of all basement membranes that is overexpressed in diabetes. The triple helical collagen fibrils attach to each other in a head-to-head orientation and form a network-like arrangement with over 20 breaks in the (Gly-X-Y)n pattern.39 The breaks contribute to the looseness with kinks that causes the collagen fibrils to form into a sheet. The collagen fibrils are reinforced through cross-links catalyzed by lysyl oxidase. Laminin is another major basement membrane component like fibronectin, and collagen is overexpressed in high glucose and diabetic conditions.40–42 It is comprised of three chains, and through specific binding sites attaches to other ECM components such as collagen type IV and proteoglycans. These ECM components with their specialized repeat sequences facilitate cell-cell attachment and cell-matrix interactions. Importantly, many of these basement membrane proteins consist of a series of independently folded polypeptide repeats, which may have relevance to basement membrane structure and function.
Sprouting Angiogenesis
Sprouting angiogenesis is a multistep process involving degradation of the basement membrane, invasion into stroma, endothelial cell proliferation, and formation of sprout and tubular structures. It is initiated when angiogenic growth factors activate receptors present on endothelial cells in preexisting vessels. The activated endothelial cells release proteinases (matrix metalloproteinases [MMPs] and urokinase) that locally degrade the basement membrane, allowing endothelial cells to escape and proliferate. Cells destined to migrate out through the degraded basement membrane form solid sprouts. As sprouts extend, endothelial cells migrate in tandem and this process is facilitated by integrins. The endothelial cells at the tips of vascular sprouts extended long filopodia. The sprouts then form loops and encircle to become a full-fledged vessel lumen (Fig. 7.1).
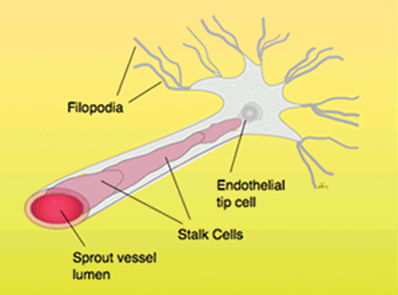
FIGURE 7.1. Sprouting angiogenesis exhibited by endothelial tip cells and their filopodia. Filopodia are highly enriched in actin filaments and respond to growth factor stimuli.
The nascent vessels continue to lengthen as cells migrating to the site of angiogenesis facilitate the extension and formation of the vessels. These cells lay collagen fibers into the core to provide an ECM for growth of the vessel lumen. Much attention in sprouting angiogenesis has focused on distinct cellular responses by specific endothelial cells present at the tip of the sprout. The tip cells sense and respond to guidance cues provided by growth factors such as vascular endothelial growth factor (VEGF)43 as they form filopodial extensions. Studies have shown that directed extension of endothelial filopodia is mediated by VEGFR2, which are highly expressed in endothelial tip cells.44,45 Importantly, studies indicate that the Dll4-Notch1 pathway, a downstream event in VEGF signaling, regulates tip cell activity and facilitates proper vascular patterning in the retina.46–48 The growth of new vessels from preexisting vessels involves complex interactions of several factors in which basement membrane plays a key role.
Proteinases in Ocular Angiogenesis
The process of angiogenesis in the retina and other tissues is characterized by distinct phases or activities including an initial response to locally produced angiogenic factors and signals.49 This event is followed by a rapid upregulation of matrix-degrading enzymes or extracellular proteinases that facilitate the breakdown of the capillary basal lamina and migration and subsequent invasion of activated endothelial cells into the surrounding extracellular tissues. Extracellular proteinases (matrix-degrading enzymes) not only help in the degradation of interstitial ECM and basement membranes but they also help in the recruitment of progenitor cells into the ECM during tissue remodeling.50 Proteinases are expressed by normal cells in tissue-remodeling events (wound healing) and also during pathological events such as cancer, DR, and age-related macular degeneration (AMD) (Fig. 7.2).
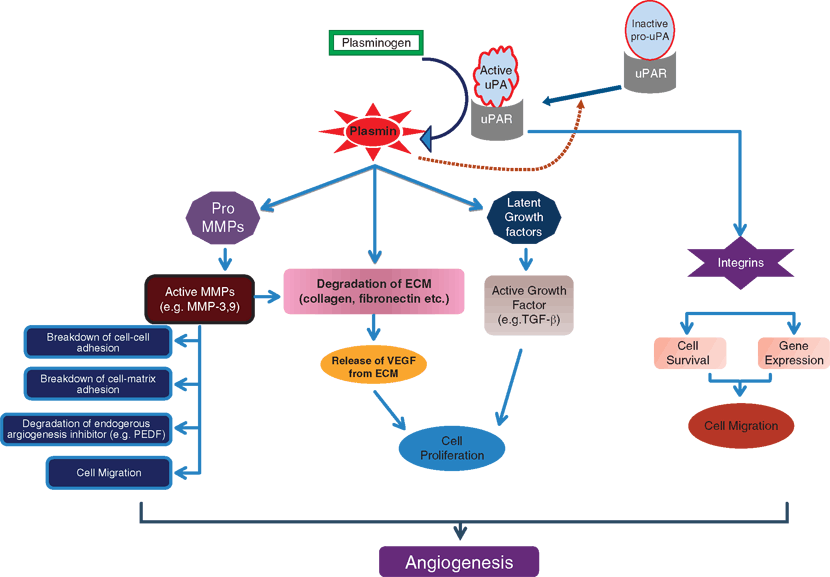
FIGURE 7.2. Role of proteinases in angiogenesis. Binding of urokinase receptor (uPAR) to inactive urokinase (pro-uPA) promotes the formation of active uPA. Active uPA then proteolytically converts the inactive zymogen plasminogen to active plasmin, which then breaks down ECM components or activates latent growth factors such as transforming growth factor-1 (TGF-1). Also, the active uPA-uPAR complex can integrate with integrins molecules and activate multiple signaling pathways, leading to cell migration. Plasmin activation also leads to the activation of pro–matrix metalloproteinases (pro-MMPs) leading to the formation of active MMPs, which can promote further ECM degradation and cell migration by its proteolytic activity. Activation of these proteases from the activated endothelial cells through the interaction between uPA and uPAR culminates in the angiogenesis process through the above-mentioned mechanisms.
Extracellular Proteinases
Two families of proteinases that have been well studied in this process include the serine proteinase, urokinase plasminogen activator (uPA), and members of a family of zinc-dependent endopeptidases called matrix metalloproteinases (MMPs).
The Urokinase Plasminogen Activator System (uPA/uPAR System)
The roles of this serine protease urokinase-type plasminogen activator (uPA) and its receptor (uPAR) in retinal choroidal neovascularization have been well documented in recent studies. uPA is produced as an inactive single-chain protein known as pro-uPA, which binds to uPAR and is activated by plasmin. Receptor-bound pro-uPA is more rapidly cleaved by plasmin than the unbound form. The uPA is present in cells in two molecular forms, a 54-kDa high molecular weight form and a 32-kDa low molecular weight form, which lacks the amino terminal fragment (ATF) of the protein.51,52 The ATF contains the growth factor and kringle domains of the protein that mediate binding to uPAR and play an important role in cell proliferation.53 The main function of the uPA is to convert the inactive zymogen form of the enzyme plasminogen to plasmin, a broad spectrum of proteinase, which can cleave variety of ECM components including collagen IV, fibronectin, and elastin including uPA. The invasive and migratory potential of endothelial cells is largely determined upon the pool of active urokinase available on the cell surface. uPA has also shown to directly activate the prohepatocyte growth factor/scatter factor (HGF/SF), and it also cleaves fibronectin and its own inhibitor, plasminogen activator inhibitor-1 (PAI-1), in a plasminogen-independent manner. The uPA/uPAR interaction represents a sensitive and flexible system to regulate proteolytic potential in endothelial cells. uPAR is a cell-surface molecule that interacts with many potential ligands.54 uPAR has also shown to be associated with several members of the INTEGRIN family, which plays an important role in cell adhesion and migration.55 This process is mediated through the low-density lipoprotein receptor– related protein (LRP), a multiligand receptor that can interact with both PAI-1 and uPAR. The uPA system also plays an important role in the activation of several MMPs and in the release and activation of growth factors stored in the ECM. The contribution of the uPA/uPAR system to angiogenesis has been studied in several animal models of tumor angiogenesis, choroidal and retinal angiogenesis (Box 7.1).
BOX 7.1 Urokinase in Retinal and Choroidal Angiogenesis
uPA is a protein that regulates the activation of plasminogen to the serine protease plasmin at the leading edge of cells. Binding of pro-uPA to uPAR allows activation of pro-uPA into the active enzymatic form (uPA) (Fig. 7.3), which then mediates the plasmin generation. UPA-mediated conversion of plasminogen to plasmin regulates many functions including the degradation of extracellular matrix (ECM) components and other extracellular membrane proteins. This activity also regulates cell proliferation, migration, and physiological and pathological tissue remodeling. uPAR has been implicated in various pathological angiogenesis such as in tumor, retinal and choroidal neovascularization (CNV). A newly designed peptide inhibitor of the urokinase system, A6 (an octapeptide that inhibits the interaction of uPA with uPAR), was shown to reduce the extent of retinal neovascularization and uPAR expression in the experimental animals.64 Also, systemic administration of the uPA/uPAR inhibitor, A6, dose dependently reduces laser-induced CNV. Also, in another study, it was found that the intravitreal injection of an adenoviral vector carrying the murine amino-terminal fragment (ATF) (targeting urokinase system) has been shown to inhibit retinal neovascularization in the OIR level.68
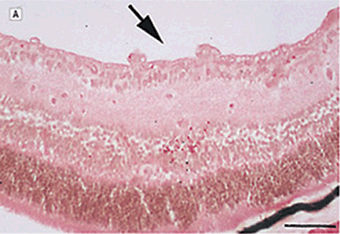
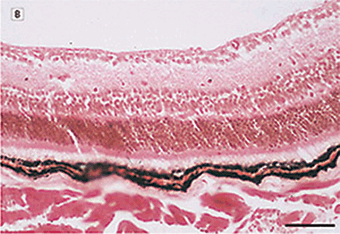
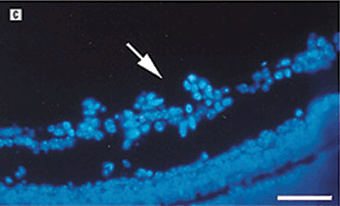
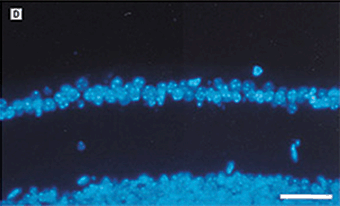
FIGURE 7.3. Use of a MMP inhibitor suppresses the development of retinal neovascularization. A: Hematoxylin-eosin–stained cross section from the retina of a mouse exposed to 75% oxygen for 5 days followed by room air for an additional 5 days. Capillary tufts are present on the vitreal side of the inner limiting membrane, characteristic of the angiogenic response in this tissue (arrow ). B: Representative hematoxylin and eosin–stained section from the retina of an experimental mouse treated with BB-94m 1 mg/kg, on postnatal days 12, 14, and 16. C: Similar section from an experimental animal stained with diamidinophenylindole showing individual endothelial cell nuclei that belongs to new vessels (arrow ). D: Similar section from the retina of a BB-94–treated mouse stained with diamidoniphenylindole showing a significant reduction in the number of neovascular nuclei. Only a single endothelial cell nucleus is present on the vitreal side of the inner limiting membrane. Scale bars: A and B, 166 µm; C and D, 113 µm. (Reproduced from Das et al. Arch Ophthalmol. 1999;117:498–503, with permission.)
Matrix Metalloproteinases
The MMPs are a family of zinc-dependent proteases, originally identified for their ECM-degrading capabilities. At least 25 different types of MMPs have been identified to date. Based on their substrate specificity and cellular localization, MMPs are grouped into the collagenases (MMP-1, MMP-8, and MMP-13), the gelatinases (MMP-2 and MMP-9), the stromelysins (MMP-3, MMP-10, MMP-11), and the nontraditional MMPs (matrilysin/MMP-7, metalloelastase or MMP-12) and the membrane type MMPs (MT-MMPs) (Box 7.2).50,56
BOX 7.2 Matrix Metalloproteinases in Retinal and Choroidal Angiogenesis
MMPs are a family of highly homologous protein-degrading zinc-dependent enzymes endopeptidases, collectively referred to as metzincins. This family currently includes more than 25 members that can be divided into collagenases (MMP-1, MMP-8, and MMP-13), gelatinases (MMP-2 and MMP-9), stromelysins (MMP-3 and MMP-10), matrilysins (MMP-7 and MMP-26), and the membrane-type MMPs (MMP-14 to MMP-17 and MMP-24). MMPs are important in normal biological processes including wound healing, angiogenesis, and embryonic development. MMPs also play a major role in pathological processes such as inflammation, cancer, and eye diseases. The most important functional activity of MMPs is its ability to degrade virtually all extracellular matrix (ECM) components. Activation of MMPs will lead to degradation of matrix and cell-cell adhesion, there by promoting cell migration and angiogenesis. In retinal angiogenesis studies, use of broad-spectrum MMP inhibitor, BB-94 (1 mg/kg), has been shown to suppress retinal neovascularization.64 An orally administered MMP-inhibitor N-BPHA has been shown to reduce the CNV in an animal model.69 Also, the use of a small molecular weight synthetic MMP inhibitor, AG3340 (Prinomastat), was found to inhibit CNV in the rat model, when injected at the time of induction (prevention study).70
There are at least five distinct types of MT-MMPs (MMP-12, MMP-15, MMP-16, MMP-17, and MMP-21), and MT1-MMP has been studied in relation to its ability to regulate focused matrix lysis and the activation of MMP-2. In addition to their capacity to degrade a large variety of ECM molecules, MMPs are known to process a number of bioactive molecules. MMPs regulate a variety of cell behaviors such as cell proliferation, migration, differentiation, apoptosis, and host defense. In relation to retinal neovascularization, MMPs are implicated in the angiogenic process for their involvement in endothelial cell migration and invasion and posttranslational modification of antiangiogenic growth factor receptors (Fig. 7.3).
Endogenous Inhibitors of Proteinases
Tissue Inhibitors of Metalloproteinases
MMP activity is in part regulated by the Tissue Inhibitors of Metalloproteinases (TIMPs), which bind MMPs and inhibit their activity. Four TIMPs have been identified, which have overlapping activities with respect to their inhibition of most soluble MMPs.50,57 More specificity exists regarding MT-MMPs, MMP-19, and proteolytically active ADAMs (proteins containing A Disintegrin and A Metalloproteinase domain) and ADAMTSs (proteins containing A Disintegrin and A Metalloproteinase domain with a Thrombospondin type 1 motif). TIMP-2 and TIMP-3 are good inhibitors of MT1-MMP, MMP-19, and ADAM-17, while TIMP-1 is poor in this respect. TIMPs also have biological effects unrelated to metalloproteinase inhibition. TIMP-3, but neither TIMP-1 nor TIMP-2, is involved in binding to the VEGF receptor-2 (Kinase insert Domain Receptor [KDR]) and competes for the binding of VEGF to this receptor. Overexpression of TIMP-3 can induce apoptosis. TIMP-1 and TIMP-2 display antiapoptotic properties and indirectly induce cell signaling. A point mutation of the TIMP-3 gene has been implicated in Sorsby’s fundus dystrophy, an autosomal dominant macular disease similar to wet macular degeneration, but with earlier onset of symptoms.58,59
Plasminogen Activator Inhibitors
The proteolytic activity of uPA is regulated by PAIs, which are members of the serine proteinase inhibitor (SERPIN) family. PAI-1 and PAI-2 have been found to interact with urokinase in 1:1 ratio to inhibit enzyme activity and cause enzyme/inhibitor internalization and turnover.60 PAI-1 has been shown to be a strong prognostic marker for several cancer types.61
Proteinases in Retinal Neovascularization
Significant upregulation of uPA (both the 54 and 32 kDa isoforms) along with increases in secretion and activation of MMP-2 and MMP-9 were observed in the retinas of animals with neovascularization.62 These results suggest that proteolytic activity and its regulatory mechanisms might play an important role in the angiogenic process. Examination of proteinases in epiretinal neovascular membranes removed surgically from humans with proliferative diabetic retinopathy (PDR) showed a similar increase in the levels of uPA and pro and active forms of MMP-2 and MMP-9 as compared to normal retinas.63
In an animal model of hypoxia-induced retinal neovascularization, it was found that the expression of the urokinase receptor (uPAR) was required to mediate an angiogenic response. uPAR-/- mice demonstrated normal retinal vascularity but showed a significant reduction (by 73%) in the extent of pathological neovascularization as compared to wild-type controls (Fig. 7.4).64 Also the expression of uPAR mRNA was upregulated in experimental animals during the active phase of angiogenesis and uPAR protein was localized to endothelial cells in the superficial layers of the retina.
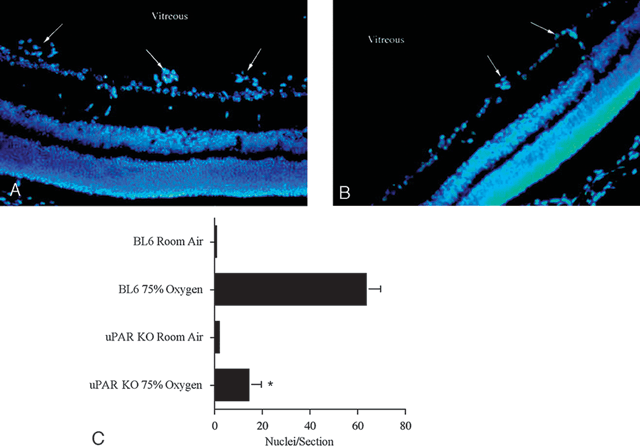
FIGURE 7.4. Absence of the urokinase receptor uPAR reduced the extent of retinal neovascularization in the mouse. A: Representative section of the retina from an experimental oxygen-treated P17 C57BL6 mouse demonstrating numerous neovascular tufts on the surface of the retina (arrows.). B: A similar section from an experimental oxygen-treated P17 uPAR-/- mouse with many fewer vascular tufts (arrows). C: Quantitation of neovascularization in C57BL6 and uPAR-/- mice. The uPAR-/- mice demonstrated 73% less neovascularization compared with the normal C57BL6 mice. Values are the mean ± SEM for n = 4 mice in each group (eight eyes, 15–20 sections/eye). *Significantly less than in C57BL6 mice, p < 0.01. (Reproduced from McGuire et al. Invest Ophthalmol Vis Sci. 2003;44:2736–2742, with permission.)
In the retinas of normal mice, TIMP-2 mRNA and protein levels have been found to increase steadily between postnatal days 13 to 17. This was in contrast to retinas of mice with hypoxia-induced retinal angiogenesis, in which TIMP-2 mRNA and protein remained low and significantly less than in retinas of “room air” controls.65 Thus, a temporal correlation between proteinases (MMP-2 and MMP-9 and MT1-MMP) and TIMP-2 was seen in retinas with neovascularization as compared to controls.65
Proteinases in Choroidal Neovascularization
Real-time PCR studies have shown the upregulation of uPA and uPAR in the choroidal tissues of mice with laser-induced choroidal neovascularization (CNV) as well as in CNV membranes from patients with AMD.66,67 The uPAR localized to endothelial cells of fibrovascular tissue within the CNV complex in the laser-induced NV model. Studies in single-gene-deficient mice have shown that the absence of uPA, tPA (tissue plasminogen activator), or plasminogen significantly decreased the development of experimental CNV and that this effect could be explained by a modulation of MMP activity in the laser-induced wounds.
Inhibition of Retinal and Choroidal Neovascularization
Inhibition of Retinal Angiogenesis by MMP Inhibitors
Systemic injection of a broad-spectrum MMP inhibitor, BB-94 (1 mg/kg), in the murine model has been shown to suppress retinal neovascularization by 72%64 (see Fig. 7.3). The retinas of BB-94-treated animals demonstrated a significant decrease in the levels of active forms of MMP-2 and MMP-9 compared to controls. In a mouse model of oxygen-induced retinopathy (OIR), the extent of preretinal neovascularization was drastically reduced in MMP-2-/- (75%) and MMP-9-/- mice (44%) at postnatal day 19, compared to wild-type control mice. The functional association of MMP-2 and αvβ3 on the cell surface of angiogenic blood vessels points to the ability of MMPs to regulate cell adhesion-and integrin-mediated behavior.
Inhibition of Retinal Angiogenesis by Inhibitors of the uPA/uPAR System
A peptide inhibitor of the urokinase system, A6 (an octapeptide that inhibits the interaction of uPA with uPAR), was able to reduce the extent of retinal neovascularization and uPAR expression in the experimental animals64 (Fig. 7.4). Intravitreal injection of an adenoviral vector carrying the murine amino-terminal fragment (ATF) has been shown to inhibit retinal neovascularization by 78% in the OIR level.68 These results suggest that inhibition of the urokinase receptor might be a promising target for antiangiogenic therapy in the retina.
Inhibition of Choroidal Neovascularization by MMP Inhibitors
In an animal model of laser-induced CNV, an orally administered MMP-inhibitor, N-biphenyl sulfonyl-phenylalanine hydroxamic acid (BPHA), has been shown to reduce neovascularization.69 Similarly, intravitreal injection of a nonpeptide small molecular weight synthetic MMP inhibitor, AG3340 (Prinomastat), was found to inhibit CNV in the rat model, when injected at the time of induction (prevention study).70 However, once the new vessels are formed, the drug did not help in reducing the CNV (regression study). A clinical trial, an oral MMP inhibitor, AG 3340 (Agouron Pharmaceuticals), in wet ARMD (Age Related Macular Degeneration) patients did not show any effective results.
Inhibition of Choroidal Neovascularization by Inhibitors of the uPA/uPAR System
Systemic administration of the uPA/uPAR inhibitor, A6, in mice has been shown to significantly reduce laser-induced CNV by 94%, and the response was found to be dose dependent67 (Fig. 7.5). Thus, studies in both retinal neovascularization and CNV show that the uPA/uPAR system plays an important role in this process, and the uPA/uPAR system may represent a novel therapeutic target in development of the antiangiogenic therapy for ocular angiogenesis (Box 7.3).
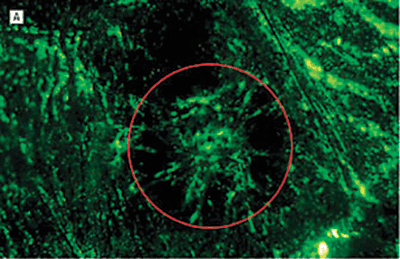
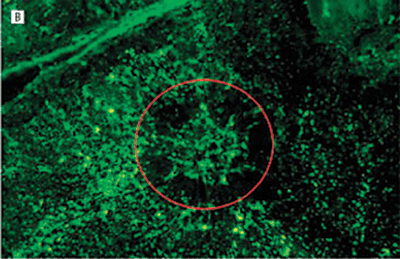
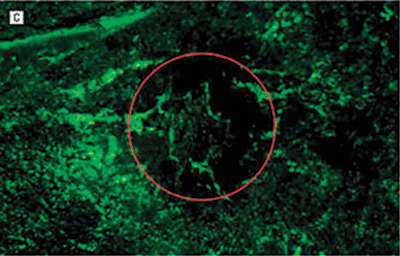
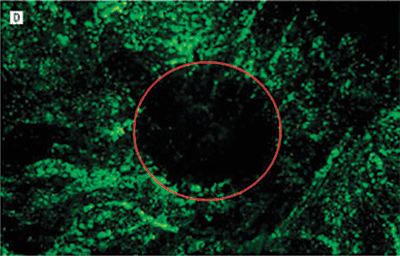
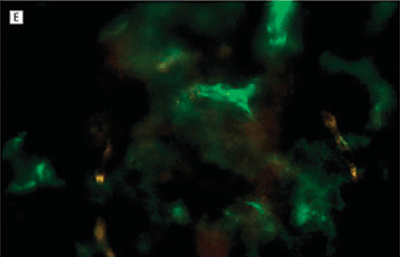
FIGURE 7.5. Treatment prevents neovascularization in the laser-induced model of CNV. Representative images of RPE–choroid whole mounts infused with fluorescein isothiocyanate–conjugated dextran 14 days after laser induction of CNV. Images are from mice treated with phosphate-buffered saline or Å6 peptide dosed at differing frequencies. The red circle roughly outlines the area of the laser burn. The fluorescence in the center of the burn area demonstrates the extent of the new vessel formation under the retina. The surrounding fluorescence represents the normal choroidal vasculature. A: Mouse treated with phosphate-buffered saline twice a day for 14 days. B: Mouse treated with the Å6 peptide, 100?mg/kg twice a day once a week. C: Mouse treated with Å6, 100?mg/kg twice a day every 3rd day. D: Mouse treated with Å6, 100?mg/kg twice a day every day. E: A higher magnification image of the central region in section D. Only a few fluorescein isothiocyanate–conjugated dextran-labeled blood vessels could be seen in this area. (Reproduced from Das et al. Arch Ophthalmol. 2004;122:1844–1849, with permission.)
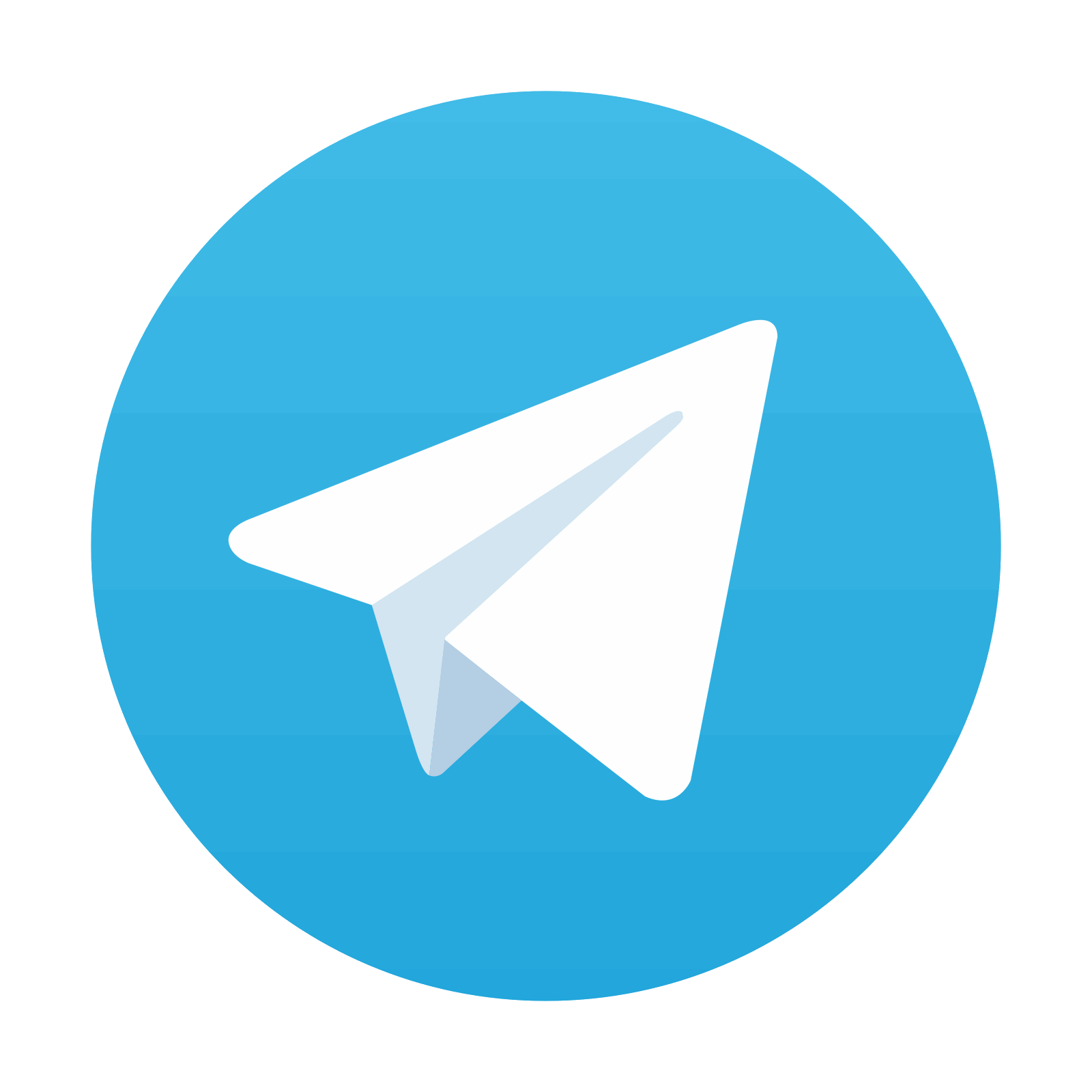
Stay updated, free articles. Join our Telegram channel

Full access? Get Clinical Tree
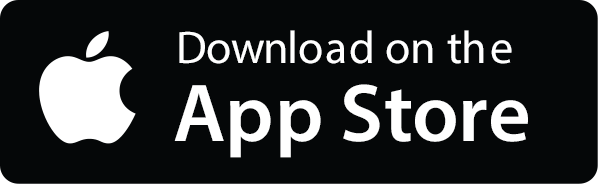
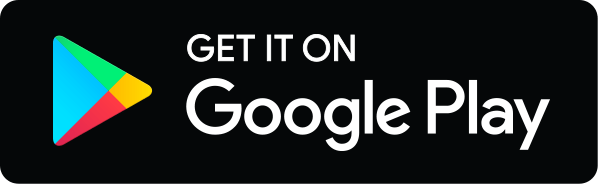