Summary
The relationship between structure and function of the optic nerve is a defining characteristic of glaucoma. This chapter describes how structure–function maps have been created and how they can aid the clinician in the care of glaucoma patients. The question of whether optical coherence tomography (OCT) damage precedes visual field loss in glaucoma is addressed. How structural changes are linked to functional changes in glaucoma is also discussed. Examples of structure–function relationships are provided.
Key words
optical coherence tomography – visual field testing – perimetry – HVF 24–2 – HVF 10–26 Structure–Function Relationship
6.1 Introduction
Glaucoma is a progressive optic neuropathy characterized by typical changes in the optic nerve head (ONH) and retinal nerve fiber layer (RNFL). 1 These structural changes usually lead to functional losses as measured by perimetric examination. Both structural and functional changes result from a common pathophysiological process, namely, loss of retinal ganglion cells (RGCs) and their axons. An understanding of the structure–function relationship in glaucoma, that is, how the changes in the ONH and RNFL are related to perimetric losses, is central to clinical practice. With the development of sophisticated imaging technologies, such as optical coherence tomography (OCT), it has been possible to detect very small structural changes in the optic nerve, macula, and RNFL, to the micrometer scale. However, from a patient’s standpoint, it is important to understand how such changes are related to, or predictive of, clinically relevant outcomes, as manifested by loss of vision.
6.2 Mapping Structural to Functional Loss in Glaucoma
In most patients with clearly defined glaucomatous damage, abnormalities on OCT imaging of the ONH and RNFL correspond topographically to abnormalities on standard automated perimetry (SAP), following a structure-to-function mapping. Several approaches have been used to derive such maps correlating regions of the ONH and RNFL to the locations tested by SAP. The most commonly used map was produced by Garway-Heath and colleagues. 2 The authors mapped localized RNFL defects seen on red-free photographs to the location of points on SAP in 63 patients with glaucoma. The authors then built a correspondence map of structure and function, which has been widely used in practice and is illustrated in Fig. 6‑1. An appreciation of these maps can help clinicians understand the functional implications of certain patterns of structural damage. In other words, given a certain structural abnormality where should one expect to see a visual field defect? The assessment of structure–function correspondence helps to determine whether a particular finding on an imaging examination or on the visual field test has a higher chance of being true or may be a false-positive. This is illustrated on Fig. 6‑2, which shows RNFL loss observed on spectral domain OCT (SD-OCT) and fundus photography in the inferior temporal region associated with the superior nasal visual field defect on SAP.


Several authors have proposed variations of the structure–function maps using different techniques to map the locations of structural abnormality to visual field defects. In general, the results of all these modeling approaches yield similar results and, from a clinical standpoint, the differences do not bear much relevance. In a recent study, Mariottoni and colleagues 3 used artificial intelligence (deep learning) to map locations of damage on SD-OCT to SAP. The study included 26,499 pairs of SAP and SD-OCT from 15,173 eyes of patients with glaucoma or suspected of having the disease. Using a deep learning neural network, the authors showed how specific localized RNFL defects on SD-OCT could be mapped to specific locations on SAP.
Investigating central visual field defects and macular glaucomatous damage in patients with glaucoma, Hood et al 4 described the “macular vulnerability zone” (MVZ), a region that would correspond approximately to the sector between 300 and 330 degrees in the inferior RNFL thickness profile. They associated defects in the MVZ to superior paracentral visual field defects. Fig. 6‑3 illustrates a glaucoma eye showing an inferior arcuate RNFL defect which extends to the macular region, affecting the MVZ. The SD-OCT macular examination shows central damage inferiorly. This corresponds to a superior visual field defect. Of note, the visual field defect was not prominent on the SAP 24–2 test greyscale, even though the pattern standard deviation (PSD) was statistically significantly outside normal limits, indicating the existence of a defect. On the 10–2 test, the defect is shown more extensively due to the higher density of points in the central region. Given that macular damage in glaucoma has been shown to occur more frequently than previously thought, this has led some investigators to suggest that 10–2 visual field tests should be performed routinely for glaucoma assessment. However, given inherent trade-offs in resources, and patient availability, such an approach may lead to a lack of enough 24–2 tests being acquired over time and inability to assess progression and, therefore, is not generally recommended.

Of note, superior localized RNFL defects resulting in small paracentral inferior visual field losses are relatively less common. Because of the relative anatomic positions of the optic nerve and macula, RNFL damage to the corresponding superior sector (30 to 60 degrees) actually tends to cause peripheral arcuate visual field defects inferiorly, rather than central macular defects. Fig. 6‑4 illustrates a case where a dense inferior paracentral defect is seen, associated with a localized superior RNFL defect in an eye with glaucoma. However, it can be seen from the total and pattern deviation plots of the visual field that the defect is actually more extensive than the greyscale plot suggests, actually affecting most of the inferior hemifield of the 24–2 test.

Anatomically in the fovea, ganglion cell bodies are spatially displaced from their corresponding photoreceptors. This is relevant for structure–function mapping in the macular region because it creates a small spatial offset between the location of the photoreceptors that detect the centrally tested visual field locations (e.g., using the 10–2 test grid) and the location of expected corresponding RGC loss. This displacement has been previously studied by several authors and has recently been incorporated into processes for structure–function analysis in the macular region in glaucoma. According to Hood and co-authors, 4 improved concordance between structural and functional measures in the macula may be obtained by applying displacement of the visual field locations according to anatomical estimates of Henle fiber length. It should be noted, however, that for most eyes with glaucoma such differences have relatively little impact on actual clinical decision-making.
6.2.1 Does OCT Damage Precede Visual Field Loss in Glaucoma?
There has been a longstanding debate about whether signs of structural damage precede functional evidence of glaucomatous damage. Initial work by Quigley and colleagues suggested that up to 40% of RGCs could be lost before signs of damage are observed on standard achromatic perimetry. 5 In fact, signs of structural damage at the level of the ONH, RNFL, or macula may be seen very often before clearly detectable and statistically significant visual field defects are observed on SAP. This is illustrated in Fig. 6‑5, where loss of RNFL was detected by OCT many years before the clear appearance of a reproducible visual field defect of SAP. It should be noted, however, that the idea that an eye must always lose 40% of its RGCs to develop a visual field defect is misleading. In fact, most often this is not the case. Previous histological work in monkeys has shown that visual field defects may appear in the presence of much smaller losses. The pattern of neural rim and RNFL loss may be an important factor in determining how much loss of RGCs may need to occur before a visual field defect becomes detectable. Eyes with localized RNFL defects or rim notching will often present early localized visual field defects. In contrast, in eyes with diffuse loss of neuroretinal rim, SAP defects may not be detectable for a long time and these eyes are often found to have major structural damage by the time the first visual field defect becomes apparent. This occurs because SAP parameters usually target for detection of localized, rather than diffuse, visual field defects, in an attempt to reduce confounding effects of media opacities. This is illustrated in Fig. 6‑6, which shows examinations from a 45-year-old patient with glaucoma. It is possible to see extensive and diffuse neuroretinal rim thinning and RNFL loss on the OCT. The visual field, however, shows normal PSD and glaucoma hemifield test, as well as unremarkable pattern deviation plot. However, the total deviation is indicative of diffuse loss of sensitivity, which is corroborated by the mean deviation (MD) with P < 1%. Importantly, this young subject had no media opacities or other reasons for diffuse loss of sensitivity, indicating that such loss was related to the extensive diffuse structural damage from glaucoma. Contrast these findings to those of previous examples shown in this chapter.


Of note, in some eyes, the reverse may occasionally happen, that is, visual field defects may be seen on standard perimetry in the absence of statistically significant abnormalities on imaging tests. In the Ocular Hypertension Treatment Study (OHTS) and European Glaucoma Prevention Study (EGPS), a significant proportion of eyes with ocular hypertension developed visual field loss as the earliest sign of glaucoma, as defined by the study endpoints. However, it is likely that most of these eyes actually already had signs of structural glaucomatous damage at baseline that were not clearly detected by subjective assessment of disc stereophotographs. Due to the wide normative range of RNFL thickness values, in some eyes it is possible to see the presence of repeatable visual field defects in the absence of clearly statistically significant OCT damage. Whether structural or functional change occurs first also depends on the chosen endpoints and how they are measured. Some disagreement might be always expected due to the asynchronous temporal relationship between RGC’s functional and structural decline in the glaucomatous process. The concept of “ganglion cell dysfunction” (rather than death) has also been proposed to explain why, in some patients, perimetric defects precede identifiable structural changes. In early stages of ganglion cell insult, cells may become dysfunctional, leading to a reduction in visual field sensitivity, so that “measured structure” may not be representative of functioning ganglion cell or axonal number. In spite of the above, there is no contradicting the fact that retinal nerve fiber layer abnormalities are present in many cases with normal visual fields. The reasons for this finding are more extensively explained in a few previous reports 6 and discussed below.
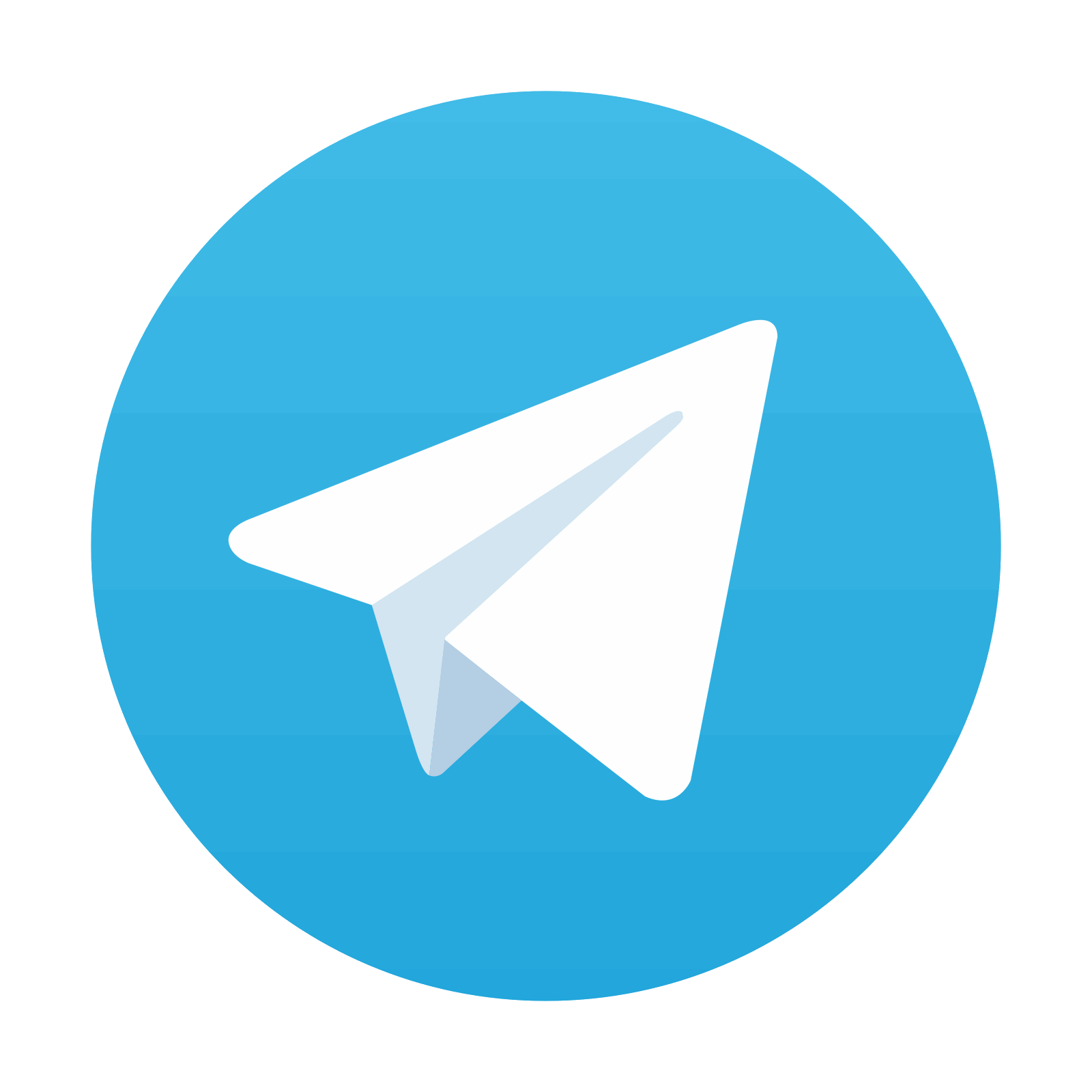
Stay updated, free articles. Join our Telegram channel

Full access? Get Clinical Tree
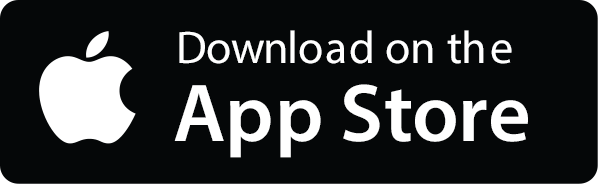
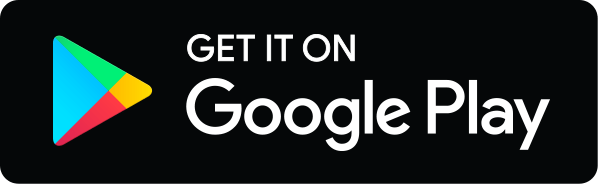
