FIGURE 5.1 Illustration of relationship among VEGF expression, astrocytes, and retinal vasculature in mouse models of retinal vascularization and OIR of prematurity. Left: Astrocytes (green) migrate from optic nerve head into the superficial layer of retina. In response to “physiologic hypoxia” that is generated as differentiating cells consume more oxygen, the astrocytes express VEGF (orange). VEGF recruits growing vessels (red). As the developing vasculature reaches the edge of the superficial layer, VEGF expression becomes apparent in the INL close to the optic nerve head and vessels descended from the superficial layer to form the deep vascular plexus. VEGF is expressed radially and the deeper vasculature layer develops more peripherally. Right: Experimental hyperoxia prior to the completion of normal vascular development leads to down-regulation of VEGF expression. The absence of VEGF causes the regression of the immature, forming vessels, resulting in the obliteration of the central retinal vessels. Once the animal is returned to normoxia, the nonperfused tissue becomes relatively highly hypoxic, inducing robust secretion of VEGF. In response to this VEGF stimulation, new vessels are formed and grow toward the vitreous in structures termed vascular tufts, a characteristic of pathologic neovascularization. (Reproduced from Saint-Geniez M, D’Amore PA. Development and pathology of the hyaloids, choroidal and retinal vasculature. Int J Dev Biol 2004;48:1049, with permission.)
Vasculogenesis and Angiogenesis
Vessel formation takes place via vasculogenesis and angiogenesis. Vasculogenesis is de novo development of vasculature that involves the proliferation, differentiation, and organization of blood vessels from endothelial cell precursors, angioblasts. Angiogenesis involves migration and proliferation of endothelial cells from an existing blood vessel, thus sprouting and forming a new blood vessel.
The evidence for vasculogenesis is based on non-specific Nissl staining, ADPase, and lectin staining that reveal a population of spindle-shaped cells at the leading edge of developing retinal vessels; these are considered to be “angioblasts.” These cells are positive for CD39 (ecto-ADPase) and CXCR4 (3,7) but not for other typical markers of endothelial precursors such as CD31, CD34, NADPH diaphorase, and VEGFR1/2 (1,8–10). Spindle-shaped proliferating astrocytes (Ki67+/GFAP+) have been identified at 18 and 20 WG in advance of developing human retinal vessels (11). In addition, close association between Pax2+/GFAP− astrocyte precursors and Pax2+/ GFAP+ immature retinal astrocytes with developing vessels (12) suggests the critical role of astrocytes in vessel growth guidance. Further support for angiogenesis in the development of the primary retinal vasculature development comes from mouse models. The expression of PDGF receptor alpha, a marker of astrocyte, was observed in spindle-shaped cells. Moreover, transgenic mice expressing GFP under the control of the GFAP promoter reveal extensive astrocyte invasion into the retina before retinal vascular development. This controversy is due, in part, to the lack of more specific markers for retinal endothelial precursors. Moreover, it is quite likely that the mechanism by which the superficial retinal vasculature forms varies among species. While a definitive answer awaits for further studies, it is well accepted that the inner plexi are formed via angiogenesis.
The Fovea
One of the unique features of primate retina is the fovea, a structural depression in the retina temporal to optic disc. The fovea is composed of high-density cone photoreceptors and is specialized for high-resolution vision. In addition, the retinal vasculature is absent from this specialized area so that there are no vascular structures present to deflect light and no blood cells moving across the fine-grain foveal cone mosaic. During retinal vascular development, radially growing vessels appear to avoid this area that is termed the FAZ. Rather the choriocapillaris supplies oxygen and nutrition as well as the removal of metabolic waste (2,4).
Other Cell Types in Vascular Development
The microvasculature in the retina is comprised of the endothelial cell tube, abluminal pericyte, and associated astrocyte processes (Fig. 5.2).
FIGURE 5.2 Schematic diagram of the cell types associated with retinal blood vessels. A monolayer of vascular endothelial cells (in white) forms the innermost layer of retinal blood vessels and is the primary site of the blood–retinal barrier. Note the branching of the mature vessel to form a new vessel, called angiogenesis. Pericytes (in light gray) ensheath the endothelial layer of the newly formed vessel and maintain endothelial cell quiescence, facilitate blood–retinal barrier formation, and regulate blood flow. Astrocytes (in dark gray) are star-shaped cells associated with retinal blood vessels on the superficial layer and contribute to endothelial tight junction formation.
Pericytes
Following the formation of the early capillary plexi by the endothelium, mural cells are recruited and mediate the process of vessel maturation. Mural cells, the collective term for smooth muscle cells and pericytes, surround and ensheath the newly formed vessels. Mural cells associated with arterioles and venules have a higher expression of α-smooth muscle actin than pericytes and enable the contractile feature of those cells. Pericytes, the mural cells that are associated with capillaries, express lower levels of α-smooth muscle actin but higher desmin (an intermediate filament protein) than smooth muscle cells. However, their abluminal location on the vessel wall is consistent with a role for the pericyte in regulating blood flow (10,13).
In addition to regulating blood flow, mural cells maintain endothelial cell quiescence by inhibiting their proliferation and migration and facilitating formation of tight junctions between endothelial cells (4,14–16). The mature retinal vascular network has highly regulated inner blood–retinal barrier, allowing the delivery of nutrition and oxygen for neural cells while meeting the anatomical requirements and minimizing the disturbance of visual signal transduction among neural cells (17). At the front of newly forming capillary beds, mural cell investment is low, leaving those endothelial cells vulnerable to regression, growth factor stimulation, and remodeling (10). Moreover, loss of pericytes leads to instability of mature vessels (18,19). Retinal capillaries have higher pericyte coverage than brain capillaries, suggesting stricter regulation of retina vasculature compared to brain capillaries (20).
Astrocytes
In the retina, there are two types of neuroglia cells, astrocytes and Müller cells. The association of neuroglia cells with retinal vascular development has been extensively studied. In humans, staining of the developing retina for GFAP reveals a population of cells extending toward the peripheral avascular area several hundred microns ahead of the vascular front. Those astrocytes proliferate and immigrate from the optic nerve, lie in front of the developing primary vascular plexus, and act as a template for retinal vessel growth (11). In contrast to the mouse retina, where astrocyte precursors migrate from the optic nerve head and distribute throughout the retina before retinal vascular development, in humans, astrocytes migrate into the retina coincident with retinal vascular development (6,11). As the retinal vessels begin to develop, they differentiate radially from optic nerve to periphery by retinal ganglion cell–derived PDGFα and guide vessel growth (21). A scaffold of astrocytes with low GFAP expression lies under the mature retinal vessels and is thought to facilitate the formation of endothelial tight junctions (22–24).
Consistent with the absence of the retinal vasculature, there is also no astrocyte invasion into the FAZ, further supporting the critical role of astrocytes in retinal vascular development (11). Müller cells are located in the INL and guide the formation and maturation of deeper vessel layers (25).
Microglia
Microglia migrate into the retina along with the developing vessels but are positioned behind the vascular front in humans at approximately 14 WG (23). Distinct from the subpopulation of microglial cells that invade the retina before vascular development around 10 WG, these cells express the macrophage marker S22 in addition to microglial markers, CD45 and MHC I and II, and remain in close contact with vessels during development as well as after they have matured (26,27). The microglia have been shown to play a variety of roles including immune surveillance and phagocytosis during vascular remodeling.
MOLECULAR MEDIATORS OF RETINAL VASCULAR DEVELOPMENT
Physiologic Hypoxia
The role of hypoxia in retinal vascular development was suggested by several characteristics of normal vascular development.
1. A retinal vasculature develops in retinas whose thickness is outside the range of oxygen diffusion. The typical thickness of vascularized mammalian retinas is 200 to 300 µm. Species whose retinas are 150 µm or less remain avascular and are supported by diffusion from the choroidal circulation (28). The avascularity of the primate FAZ is consistent with this, as the foveal depression is about 150 µm (2).
2. Development of the neural retina, which causes a local increase in metabolic demand, also begins at the optic disc and follows in central-to-peripheral fashion. Thus, retinal vessel formation follows the pattern of neural retinal development, thereby relieving the local metabolic demand (29).
3. Regions of the retina with higher oxygen supplies have a lower density of retinal vessels. For instance, there is a 100- to 150-µm-wide periarterial capillary-free zone that gradually diminishes in the transition to branching arterioles and capillaries.
Taken together, these observations support the concept of “physiologic hypoxia” as the driving force of retinal vascular development. They also suggest the existence of a retina-derived “vasoformative factor” (30), since shown to be vascular endothelial growth factor (VEGF), that is associated with the metabolic changes (oxygen) and that mediates cell proliferation and regulate retinal vessel formation. In fact, it is the disruption of physiologic hypoxia when premature infants are administered high oxygen (such as when retinopathy of prematurity [ROP] was first described in the 1940s) or experience other oxygen stresses that lead to the pathology referred to as the ROP (covered in detail in Chapter 42).
This phenomenon, coupled with the fact that the mouse retina is avascular at birth (see above), has permitted the development of a murine model of oxygen-induced proliferative retinopathy (31). In this model, postnatal mice are placed into a hyperoxic environment, which leads to a disruption of the hypoxia-induced signal and impaired vessel growth/remodeling. Upon return of the mice to room air, the tissue senses hypoxia and a compensatory up-regulation of VEGF occurs with associated vessel growth (Fig. 5.3).
FIGURE 5.3 Vaso-obliteration and neovascularization tufts in the oxygen-induced retinopathy (OIR) model. Isolectin B4 staining of retinal vasculature in mouse under normoxia or in different stages of the OIR model. Left panel: Normal vasculature of a mouse under normoxia at P13; note the normal arteries, veins, and capillaries; middle panel: the retinal vasculature of a mouse at P12 that has been exposed to hyperoxia during P7 to P12; note the vaso-obliteration in central region of the retina; right panel: the retinal vasculature of a mouse at P17 that has been exposed to hyperoxia from P7 to P12 and returned to normoxia from P12 to P17; note the neovascular tufts in the periphery of the retina. (Courtesy of Magali Saint-Geniez, Schepens Eye Research Institute.)
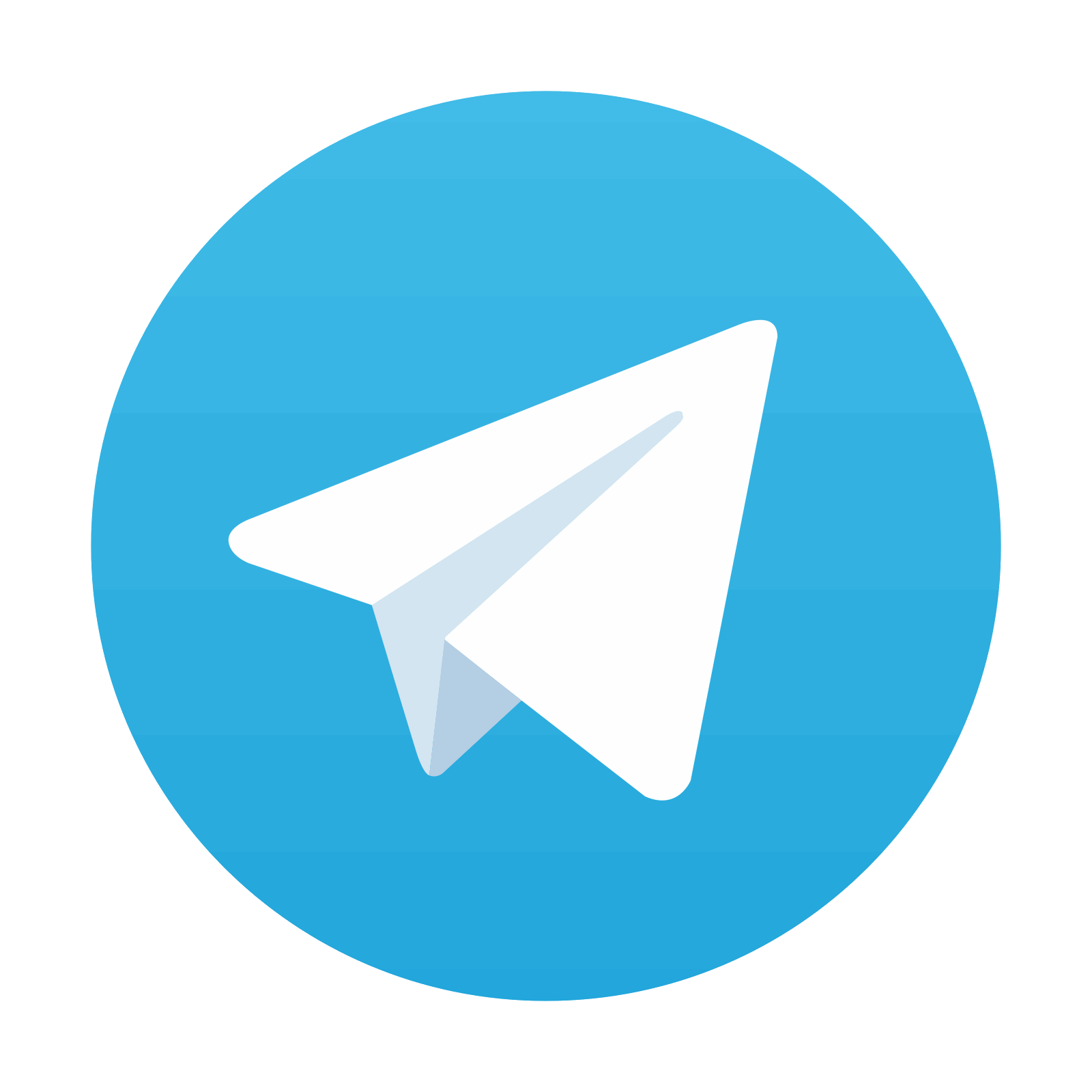
Stay updated, free articles. Join our Telegram channel

Full access? Get Clinical Tree
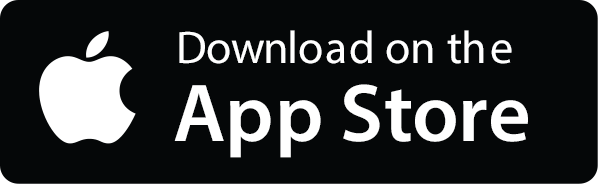
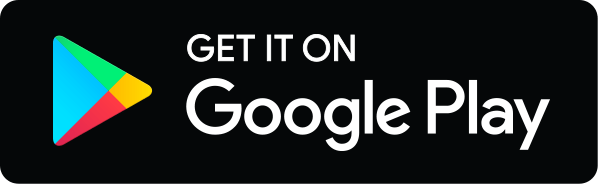