FIGURE 5.1. Effect of GH inhibition on ischemia-induced retinal neovascularization. A: Cross section of an eye from a nontransgenic littermate mouse, showing retinal neovascularization internal to the inner limiting membrane (arrows). B: Cross section of an eye from a GH antagonist G119K transgenic mouse. No vascular cell nuclei are apparent internal to the inner limiting membrane. C: Nontransgenic flat-mounted whole retina, showing extensive areas of retinal neovascularization (bright fluorescence, indicated in part with arrows) that is significantly reduced in the retinas from the GH antagonist G119K transgenic mice (D). (Reproduced from Smith et al. Science. 1997;276:1706–1709, with permission.)
IGF-1 regulation of retinal neovascularization is mediated at least in part through control of VEGF activation of p44/42 MAPK. IGF-1 acts permissively to allow maximum VEGF induction of new vessel growth.21 Inadequate levels of IGF-1 inhibit vessel growth despite the presence of VEGF (Fig. 5.2).
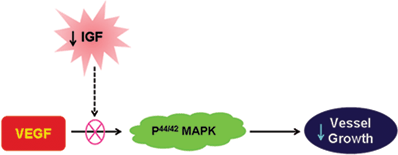
FIGURE 5.2. The relationship between IGF-I and VEGF and its effect on the growth of new blood cells.
Low Levels of Igf-1 and Phase 1 of ROP
IGF-1 is also critical to the first phase of ROP22 and to the normal development of the retinal vessels. After birth, IGF-1 is not maintained at in utero levels due to the loss of IGF-1 provided by the placenta and the amniotic fluid. We theorized that IGF-1 is critical to normal retinal vascular development and that a lack of IGF-1 in the early neonatal period is associated with a lack of vascular growth, resulting in subsequent proliferative ROP. We determined that IGF-1 is critical to normal blood vessel growth and retinal blood vessel development by examining IGF-1 null mice. We found that retinal blood vessels grew at a significantly slower rate in the IGF-1 null mice than in normal mice, a pattern very similar to that seen in premature babies with ROP. It was determined that IGF-1 controls maximum VEGF activation of the Akt endothelial cell survival pathway. This finding explains how loss of IGF-1 could cause ROP by preventing the normal survival of vascular endothelial cells.
These findings were confirmed in premature infants, where the mean IGF-1 was significantly lower in babies with ROP than babies without ROP.22,23 These results suggest that replacement of IGF-1 to uterine levels might prevent ROP by allowing normal retinal vascular development. If vascular dropout and cessation of normal vascular growth seen in phase 1 are prevented, the destructive second phase of vaso-proliferation will not occur (Fig. 5.3).
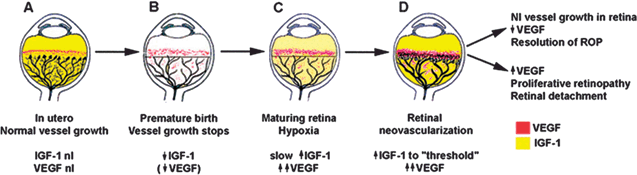
FIGURE 5.3. Schematic representation of IGF-I/VEGF control of blood vessel development in ROP. A: In utero, VEGF is found at the growing front of vessels. IGF-I is sufficient to allow vessel growth. B: With premature birth, IGF-I is not maintained at in utero levels and vascular growth ceases, despite the presence of VEGF at the growing front of vessels. Both endothelial cell survival (Akt) and proliferation (mitogen-activated protein kinase) pathways are compromised. With low IGF-I and cessation of vessel growth, a demarcation line forms at the vascular front. High oxygen exposure (as occurs in animal models and in some premature infants) may also suppress VEGF, further contributing to inhibition of vessel growth. C: As the premature infant matures, the developing but nonvascularized retina becomes hypoxic. VEGF increases in retina and vitreous. With maturation, the IGF-I level slowly increases. D: When the IGF-I level reaches a threshold at ≈34 weeks gestation, with high VEGF levels in the vitreous, endothelial cell survival and proliferation driven by VEGF may proceed. Neovascularization ensues at the demarcation line, growing into the vitreous. If VEGF vitreal levels fall, normal retinal vessel growth can proceed. With normal vascular growth and blood flow, oxygen suppresses VEGF expression, so it will no longer be overproduced. If hypoxia (and elevated levels of VEGF) persists, further neovascularization and fibrosis leading to retinal detachment can occur. (Reproduced from Hellstrom et al. Proc Natl Acad Sci U S A. 2001;98:5804–5808, with permission.)
IGF-1 in Diabetic Retinopathy
Elevated IGF-1 levels
There is a long-standing (and complex) association between IGF-1 and diabetic retinopathy,24–26 with conflicting evidence that elevated levels of serum IGF-1 are associated with proliferative retinopathy (phase 2). The hypothesis that elevated levels of IGF-1 cause proliferative retinopathy is based in part on observations that patients with neovascular disease have very high vitreous levels of IGF-1.27–31 However, it is likely that IGF system components could accumulate in the vitreous because of local production and leakage from abnormal vessels.32,33 Specifically, it is thought that diffusion from serum plays an important role,34 and it has been suggested that increased levels of IGF-1 in the vitreous are the result and not the cause of neovascularization. This is based on the well-established increased permeability of the blood-retina barrier in diabetic patients. Circulating IGF and IGF-binding protein-3 (IGFBP-3) levels are 10 to 100 times higher than those measured in vitreous.34 Furthermore, patients with proliferative diabetic retinopathy show a significant positive correlation between serum and vitreous levels of IGF-1,35 and the increase in vitreous levels of IGF-1, IGF-2, and IGFBP-3 parallels the increase in vitreous of liver-derived serum proteins. Thus, it is generally accepted that diffusion from serum plays a key role. This accumulation is caused by a nonspecific increase in leakiness of the blood-retina barrier since these same elevations are found in patients with nondiabetic causes of leaky retinal vasculature.29,34
Some longitudinal studies have demonstrated that intensive insulin treatment in patients with poorly controlled hyperglycemia, which rapidly increases total serum IGF-1, is associated with accelerated diabetic retinopathy.36,37 However, the majority of investigations (cross-sectional as well as longitudinal) have found no significant correlation between circulating IGF-1 and the development of proliferative diabetic retinopathy.38–42 An animal study of normo-glycemic/normo-insulinemic transgenic mice overexpressing IGF-1 through an insulin promoter at supraphysiological levels in the retina developed loss of pericytes (Fig. 5.4.) and thickening of basement membrane of retinal capillaries.43 In older transgenic mice overexpressing IGF-1, neovascularization of the retina43 (Fig. 5.5) and vitreous cavity were observed, which was consistent with increased IGF-1 induction of VEGF expression.2 VEGF alone has been shown to cause these same effects in primates.3 These accumulated findings suggest that once proliferative neovascular vessels occur in the retina during phase 2, leaked serum IGF-1 may further promote the proliferation of retinal vessels through stimulation of VEGF. However, it has not been established that serum IGF-1 in the absence of leaky vessels causes proliferative disease. In diabetic patients with acromegaly and elevated IGF-1 in serum and in the vitreous, proliferative diabetic retinopathy is rare44 (Box 5.1).
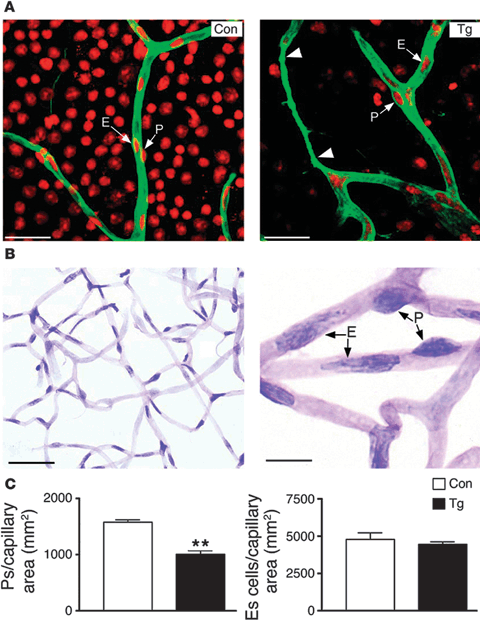
FIGURE 5.4. Pericyte loss in retinal capillaries from transgenic mice overexpressing IGF-1. A: Confocal analysis of retinal capillaries in flat-mounted retinas immunohistochemically marked with anticollagen IV (green). Nuclei were counterstained with propidium iodide (red). Acellular capillaries, lacking pericytes and endothelial cells, were observed (arrowheads) in transgenic retina. The images are single confocal sections. B: Retinal digest preparations were obtained. A representative area is shown (left panel). Endothelial cell nuclei are placed within the vessel wall, while pericyte nuclei are placed more laterally on the vessel wall (right panel). C: The number of pericytes (left) and endothelial cells (right) per square millimeter of capillary area was determined as indicated in Methods. Results are mean ± SEM of five mice in each group. **p < 0.01. E, endothelial cell; P, pericyte. Scale bars: 35 μm (A), 43 μm (B, left panel), and 11 μm (B, right panel). (Reproduced from Ruberte et al. J Clin Invest. 2004;113:1149–1157, with permission.)
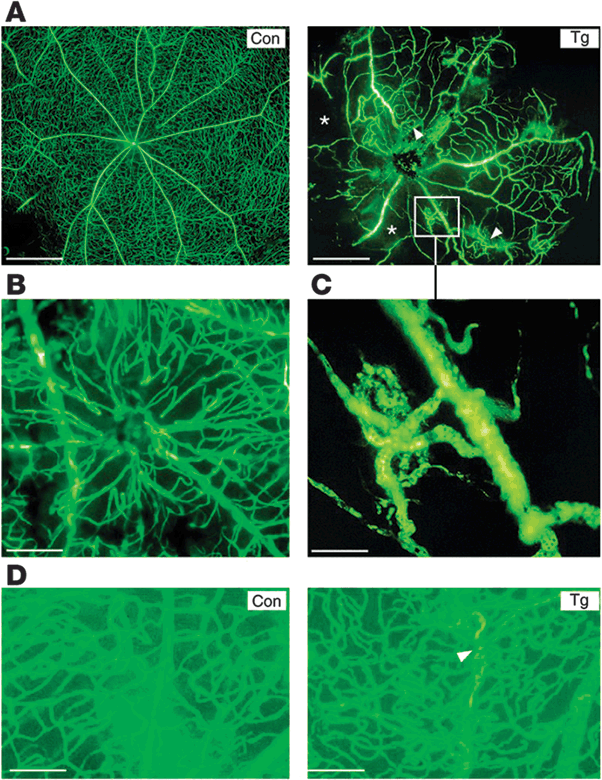
FIGURE 5.5. IRMAs (intraretinal microvascular abnormalities) and neovessels in transgenic retina. A: Flat-mounted, FITC-dextran–perfused retinas from transgenic eyes showed extensive areas of nonperfusion (asterisks) and neovessels (arrowheads and inset). B: Capillary IRMA inside the retina is shown. C: Neovessel originating from a venule (magnification of inset in A, right panel). This vessel had more endothelial cell nuclei after staining with propidium iodide and analysis with laser confocal microscopy (data not shown). D: Intraretinal dense area of neocapillaries in transgenic retina originating from a central feeding vessel (arrowhead). Scale bars: 630 μm (A), 188 μm (B), 87 μm (C), and 105 μm (D). (Reproduced from Ruberte et al. J Clin Invest. 2004;113:1149–1157, with permission.)
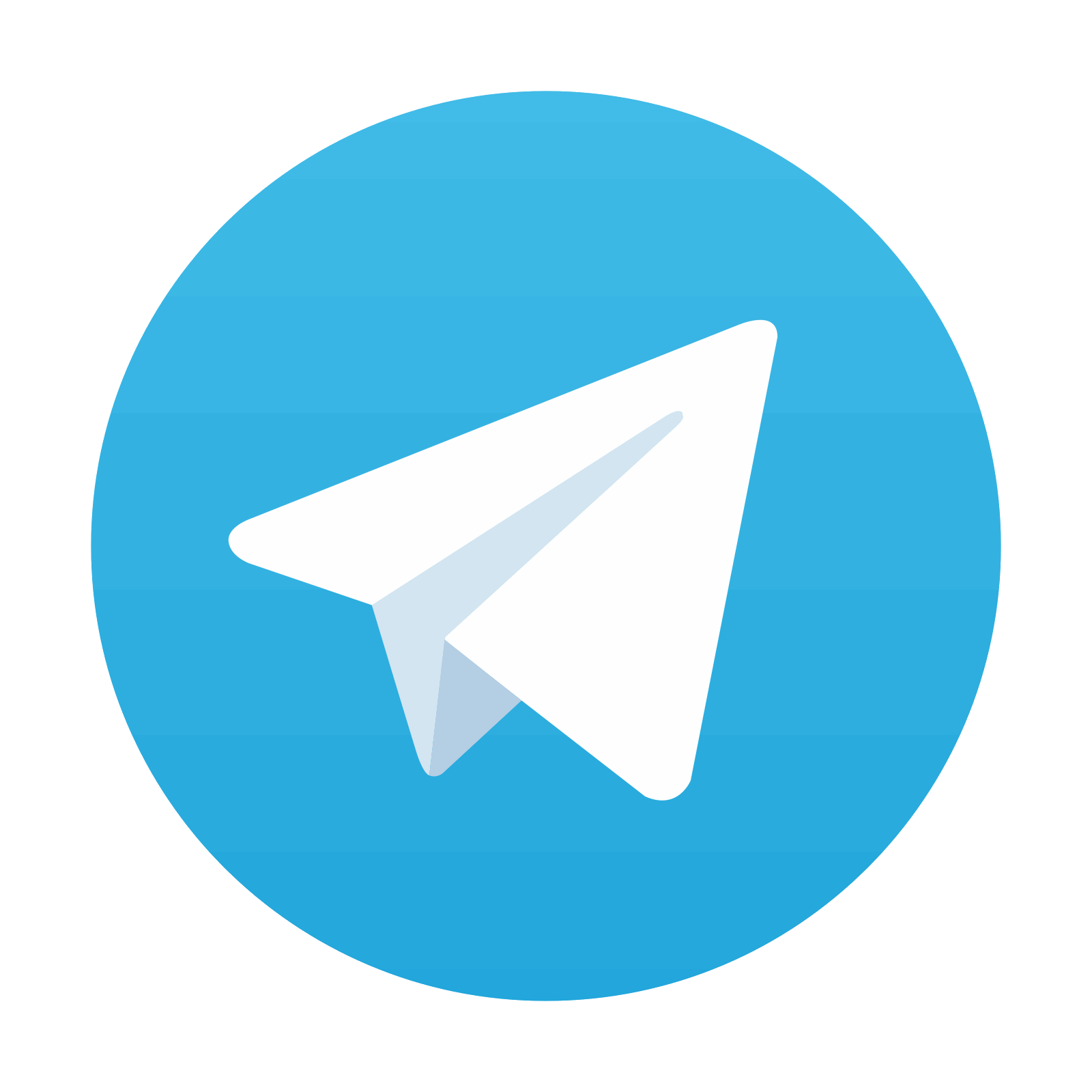
Stay updated, free articles. Join our Telegram channel

Full access? Get Clinical Tree
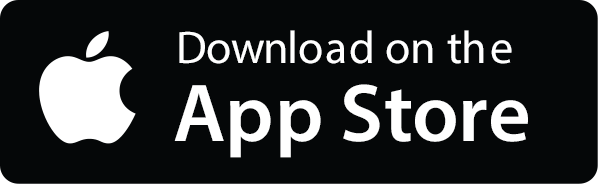
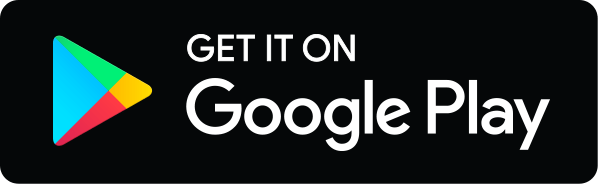