FIGURE 41.1 Box plots of BW (A) and BWSDS (taking GA and sex into account) (B). The three horizontal lines in each box marks the 25th, 50th, and the 75th percentiles. Horizontal lines outside the box mark the minimum and maximum values. In total 451 Swedish infants born from week 23 to 32 GA. (From Löfqvist C, Andersson E, Sigurdsson J, et al. Longitudinal postnatal weight and insulin-like growth factor I measurements in the prediction of retinopathy of prematurity. Arch Ophthalmol 2006;124:1711–1718; Hellström A, Hård A-L, Engström E, et al. Early weight gain predicts retinopathy in preterm infants: new, simple, efficient approach to screening. Pediatrics 2009;123:e638–e645; Löfqvist C, Hansen-Pupp I, Andersson E, et al. Validation of a new retinopathy of prematurity screening method monitoring longitudinal postnatal weight and insulinlike growth factor I. Arch Ophthalmol 2009;127: 622–627.) Infants with no ROP (stage 0) (n = 298), mild ROP (stages 1 and 2) (n = 106), and proliferative ROP (stage 3 and above) (n = 47; Reprinted from Hellström A, Ley D, Hansen-Pupp I, et al. New insights into the development of retinopathy of prematurity— importance of early weight gain. Acta Paediatr 2010;99:502–508, with permission.)
It thus appears that prenatal growth restriction may increase the risk for ROP, but data are inconsistent. One reason may be that growth restriction in the most immature babies, that is, those at the greatest risk of developing ROP, also reduces survival. SGA is then seen as a more important risk factor in more mature babies. One might speculate that impaired general growth also affects the eye and the developing retinal vasculature leaving a larger retinal area to be vascularized during an extrauterine third trimester. The larger avascular retina is then a greater hypoxic stimulus for subsequent release of angiogenic factors that cause proliferative ROP.
In 1953, Lubchenco et al. reported that retrolental fibroplasia, the end stage of ROP, occurred especially in infants not only of low birth weight but also with greater weight loss during the neonatal period, followed by a relatively fast weight gain. This observation sheds light on the importance of postnatal growth (16).
POOR POSTNATAL WEIGHT GAIN AS A RISK FACTOR FOR RETINOPATHY IN ANIMAL STUDIES
Animal studies have shown that poor postnatal weight gain is associated with increased risk for retinopathy. At birth, mice and rats born with normal weight have incomplete retinal vascularization, similar to premature human infants. Retinal vascularization continues to develop during the first few postnatal weeks in rodents. To study the effect of poor postnatal growth on ROP, the oxygen-induced retinopathy (OIR) model is used with the addition of extra pups to one dam to reduce nutrition to individual pups and cause poor weight gain.
More than 50 years ago, the Swedish researcher Bo Hellström found that starved mice with poor postnatal weight gain had poor retinal vascular development. He studied the influence of nutrition on the development of oxygen-induced retinal changes in mice, but first studied the effect of starvation on the retinal vascularization in pups raised in room air (17).
In starved animals in room air, the outgrowth of vessels of the nerve fiber layer was slightly delayed. Vessels extended to the ora serrata in 9 of 11 starved animals at 15 days of age, whereas, in normally fed animals, this occurred at 10 days of age. In addition, there were other developmental delays noted in retinal development including in the separation of the nuclear layers, the relative increase in the inner plexiform layer, the outgrowth of rods and cones, the development of secondary vitreous, and the involution of hyaloid vessels. In a later study of rat pups raised in room air, reduced nutrition accomplished by rearing extended litters of pups impaired total retinal growth, but the effect on the delay in vascularization of the retina was greater. The ratio of vascularized to total retinal area was 54% ± 8% in larger litters (18 pups/mother) compared to 67% ± 8% in smaller litters (10 pups/mother) (18). Thus, starvation per se appears to have a negative impact on both retinal vascularization and development.
In a study by Bo Hellström in 1956 (17), exposure to 98% to 100% oxygen caused a complete, or almost complete, inhibition of retinal vessel growth regardless of nutritional state of the pups. When mouse pups were transferred to room air, a vasoproliferative stage occurred, which was depressed if starvation continued. Only 2 of 14 animals with continued starvation and poor growth during the room air course developed pathologic neovascularization compared with 12 of 12 animals that were starved only during oxygen exposure. These findings support the notion that excessive loss of weight gain in the first few weeks of life followed by later catch-up growth, as commonly seen in preterm babies, is associated with increased risk of retinal neovascularization. In rat pups exposed to cyclic hyperoxia and hypoxia followed by room air exposure, retinal neovascularization occurred in 53% of rats in large litters (n = 25) versus 15% in smaller litters (n = 10). Total retinal area as well as the ratio of vascularized to total retina was smaller in rats raised in large litters. In addition, the median number of clock hours of neovascularization was 3.5 in large litters and 2.0 in small litters. Smaller ratios of vascularized to total retinal area were associated with a greater incidence and severity of abnormal neovascularization. This finding agrees with observations that the size of the peripheral avascular zone determines the incidence of neovascularization in rats exposed to variable oxygen (16,19,20).
Acidosis in the neonatal period results in acidosis-induced retinopathy (AIR), which has similar pathologic features as OIR models. AIR induces more retinal neo-vascularization in undernourished than in normally fed rat pups (21).
Poor postnatal weight gain in a mouse OIR model was also associated with a delayed onset and prolonged course of neovascularization in association with increased retinal vascular endothelial growth factor (VEGF) expression. In addition, electroretinogram responses were attenuated with significantly reduced amplitudes corresponding to bipolar cell and inner retinal function compared with medium or extensive weight gain (22).
POOR POSTNATAL WEIGHT GAIN AS A RISK FACTOR FOR RETINOPATHY OF PREMATURITY IN CLINICAL STUDIES
At very preterm birth, the supply of nutrients and growth factors provided by the maternal/fetal interaction is suddenly lost, and general growth, as well as vascular growth, slows down. In addition, it is likely that the relative hyperoxia of the extrauterine environment together with supplemental oxygen causes obliteration and regression of already formed vessels as seen in animal studies (23). Poor postnatal growth in very preterm infants is a major problem and results in reduced weight gain relative to age and sex (24).
In an early paper on postnatal weight gain and ROP, investigators reported that a reduced rate of weight gain was associated with increased ROP severity in four surviving quintuplets with identical GAs and similar BWs (25). In infants born at GA < 30 weeks, an association between poor weight gain in g/kg/day and ROP stage 3 or more was reported. Infants with no or mild ROP (n = 68) gained a mean of 10.9 g/kg/day in the first 6 weeks of life compared with 9.6 g/kg/day in those with severe ROP (n = 43). However, the difference was not apparent until the third week of life, and statistical significance was only reached during the third and fifth weeks. The authors calculated that for each 60 g below the expected weight at 6 weeks, there was a 20% increase in the risk of stage 3 ROP (26). Reduced absolute weight gain (g/day) but not relative weight gain (g/kg/day)—which is more relevant—during the first 6 weeks of life was reported in association with the development of threshold ROP compared with GA-matched controls (11). In a Brazilian study, poor weight gain during the first 6 weeks of life in infants born with BW ≤ 1,500 g and GA ≤ 32 weeks was reported to be an important and independent risk factor for severe ROP (27). Weight gain relative to BW was calculated as the difference in the infant’s weight measured at 6 weeks of life and the BW divided by the BW. Weight gain at 6 weeks that was <51.2% of BW was associated with a threefold increase in risk of severe ROP. Very low birth weight infants are expected to have regained weight, approximately 50% of their BW, after 6 weeks of life (28). In the EXPRESS study, low weight SDSs at 36 weeks’ postmenstrual age (PMA) were highly associated (P < 0.001) with ROP of stage 3 or worse (12), providing support that poor postnatal weight gain up to 36 weeks’ PMA with later catch-up growth is a strong risk factor for ROP, whereas appropriate postnatal weight gain through 36 weeks’ PMA had lower risk.
Insulin-like growth factor I (IGF-I) promotes anabolism and is essential for growth during the third trimester and for normal vascularization of the human retina (29). In a study of 84 infants born between 24 and 32 weeks’ GA (30), low serum IGF-I values correlated both with the presence of ROP and increased ROP severity. The mean ± SEM serum IGF-1 level during 30 to 33 weeks PMA was lowest (IGF-1 of 25 ± 2.41 µg/L) in patients with severe ROP, intermediate (29 ± 1.76 µg/L) with moderate ROP, and highest (33 ± 1.72 µg/L) with no ROP. In addition, the duration that low IGF-I levels were present correlated with increased severity of ROP. The age at which serum levels of IGF-I reached 33 µg/L was 52 ± 7.5 days for severe ROP, 44 ± 4.8 days for moderate ROP, and 23 ± 2.6 days for no ROP. Other prematurity-related morbidities, such as bronchopulmonary dysplasia, intraventricular hemorrhage (IVH), and necrotizing enterocolitis (NEC), were also correlated with low IGF-I values.
Postnatal Weight Gain and Prediction of Severe ROP
The relationship between postnatal weight gain and ROP can be used very early in the neonatal course to predict the risk for severe ROP. The value of this prediction would be that care could be concentrated on those infants more likely to develop severe ROP and fewer examinations done on infants at low risk. In Figure 41.2, postnatal weight development is presented as SDSs in 129 infants with no, mild, and proliferative ROP, respectively, from two populations (13,15).
FIGURE 41.2 Postnatal weight development in 129 infants from two study populations. (Löfqvist C, Andersson E, Sigurdsson J, et al. Longitudinal postnatal weight and insulin-like growth factor I measurements in the prediction of retinopathy of prematurity. Arch Ophthalmol 2006;124:1711–1718; Löfqvist C, Hansen-Pupp I, Andersson E, et al. Validation of a new retinopathy of prematurity screening method monitoring longitudinal postnatal weight and insulinlike growth factor I. Arch Ophthalmol 2009;127:622–627.) (Reprinted from Hellström A, Ley D, Hansen-Pupp I, et al. New insights into the development of retinopathy of prematurity—importance of early weight gain. Acta Paediatr 2010;99:502–508.)
With current screening criteria based on GA and sometimes BW, only 5% to 10% of infants who undergo repeated painful and stressful eye examinations develop severe ROP requiring treatment. Therefore, more efficient ways to detect infants at risk are needed.
The finding that risk of severe ROP is increased in infants with low serum levels of IGF-I, which is essential for growth during the third trimester, suggested that early identification of infants at risk for severe ROP might be possible using the variables IGF-I and weight gain. Hence, the algorithm Weight IGF-I Neonatal ROP (WINROP) was constructed to detect deviations of any child from the expected weight gain of those infants who developed no ROP. Initially in the same way, deviations from the IGF-I level were also used in WINROP using online statistical surveillance (13) (www.winrop.com). Each week after birth, weight and IGF-I levels are entered into the algorithm, and if the accumulated deviation reaches a certain level set to include all infants at risk of severe ROP, an alarm is shown that indicates that the child is at high risk for proliferative ROP. The algorithm is based on initial data from 79 Swedish infants born at GA < 32 weeks, 38 with no or mild ROP, and 13 with proliferative ROP. Validations have been completed in other cohorts. WINROP was first validated in 50 infants born at GA < 31 weeks in Lund, Sweden. In this cohort, WINROP correctly identified 9/9 infants who developed proliferative ROP on average 9 weeks (1.1 to 21.6 weeks) later (100% sensitivity) with a specificity of 54% (15).
Serial analyses of serum IGF-I are costly and not universally available. Furthermore, weight gain also reflects IGF-1 levels. Therefore, studies were performed to determine if predictions of severe ROP could be accurately made using weight gain only. To determine if WINROP could be used without IGF-I values, a retrospective study was undertaken in which weekly weights of 353 infants were entered into WINROP. Infants with hydrocephalus, which causes nonphysiologic weight gain due to accumulation of fluid in the head, were excluded. Using the level of accumulated deviation in weight gain that triggers the alarm before 33 weeks’ PMA to predict stage 3 ROP, the sensitivity of WINROP was 100% and the specificity 84.5%. As in the initial WINROP study, the alarms came well before the development of stage 3, severe ROP. For 35 infants who developed proliferative ROP, the chronologic median age when there was an indication of high risk for proliferative ROP was 3.1 weeks (range 2 to 10 weeks), and the median time from the alarm to diagnosis of stage 3 ROP was 7.7 weeks (range 1 to 16 weeks) (Fig. 41.3). It was estimated that by using WINROP, 76% of infants fulfilling screening criteria in this population would not need any eye examinations (14). The algorithm requires validation in many different populations since ROP varies throughout the world. It can be used in clinical practice as an adjunct to standard care to attend most to infants with an alarm and to reduce traditional screening in infants with no alarm.
FIGURE 41.3 Time after birth for alarm, first observed proliferative ROP (diagnosis), and treatment for children with proliferative ROP (n = 35). (Reprinted from Hellström A, Hård A-L, Engström E, et al. Early weight gain predicts retinopathy in preterm infants: new, simple, efficient approach to screening. Pediatrics 2009;123:e638–e645, with permission.)
WINROP using postnatal weight gain alone has been tested in the United States (31), Brazil (32), Switzerland (33), and Mexico (34). The sensitivity and specificity in different populations of infants are presented in Table 41.1. Further studies are being performed in the United States, China, and England. In Gothenburg, other Swedish cities, and Boston, WINROP was useful as a tool to reduce the number of eye examinations performed in infants without alarms. At our clinic in Gothenburg, more mature infants (GA = 30 weeks) without alarms are examined at PMA 35 to 37 weeks and examined no further if no ROP is found at that time.
TABLE 41.1
The sensitivity and specificity of WINROP (using weight only) in predicting severe ROP in different populations of infants
WINROP, Weight IGF-I Neonatal ROP; ROP, Retinopathy of Prematurity.
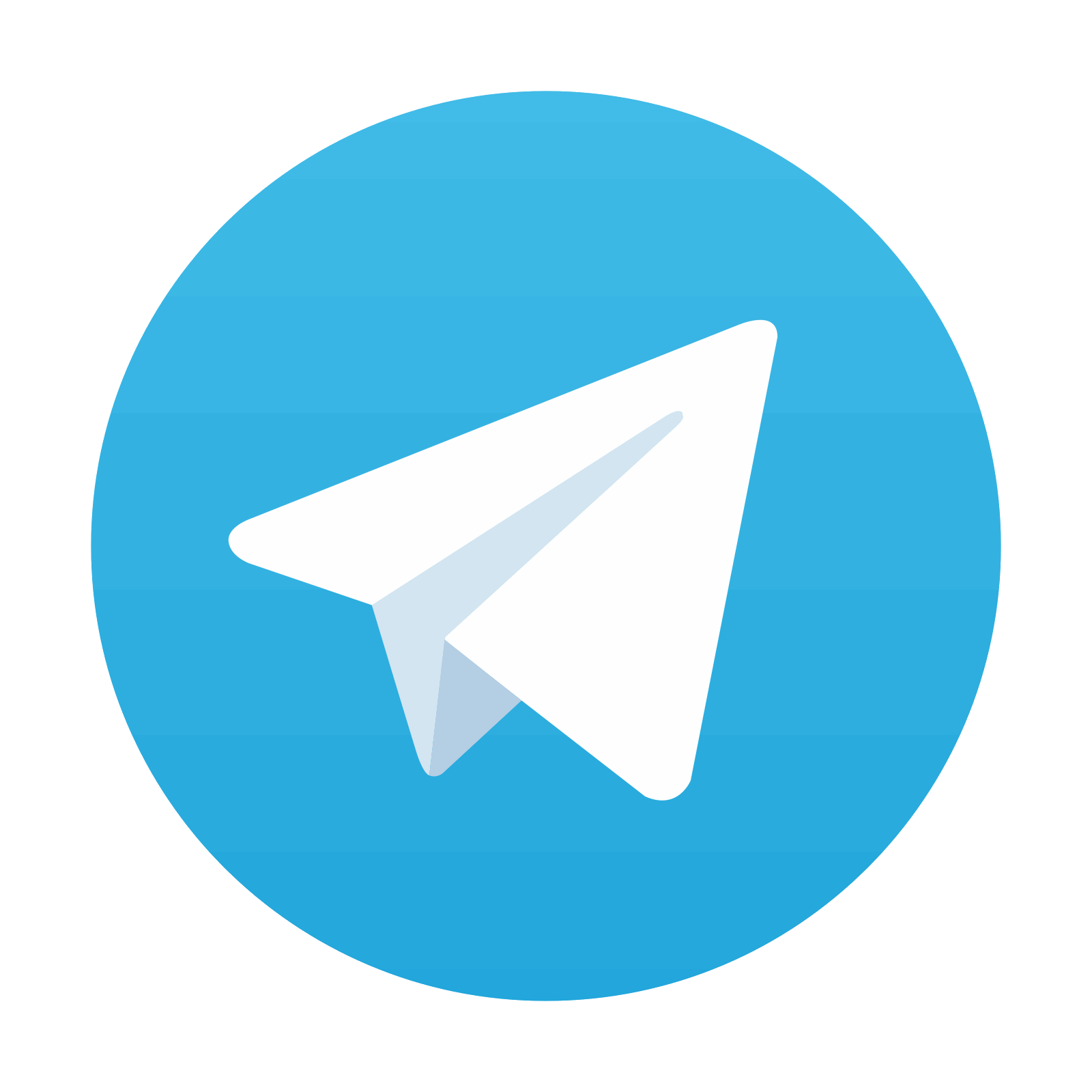
Stay updated, free articles. Join our Telegram channel

Full access? Get Clinical Tree
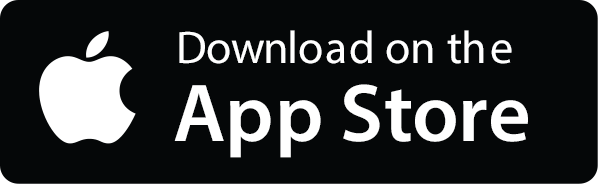
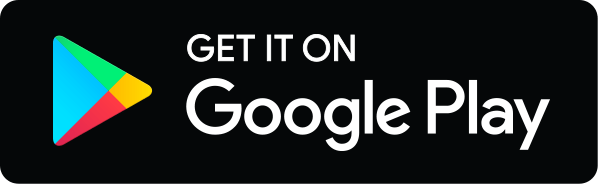