FIGURE 4.1. Summary diagram of VEGF expression in the developing retina. (Reprinted from Stone et al. J Neurosci. 1995;15:4738–4747, with permission.)
In addition to the well-studied transcriptional induction of VEGF and other hypoxia-regulated genes such as erythropoietin,46 mRNA stability is another mechanism for increasing message, and hence protein, levels. As is the case for many other growth factors and cytokines, the half-life of the VEGF mRNA in cultured retinal pigment epithelium (RPE) is quite short—at 45 minutes—and incubation of cells under hypoxic conditions leads to a dramatic increase of the half-life to 8 hours.47 This effect has been shown to be mediated by a region of the 3′ untranslated region of the VEGF transcript. Using a tumor cell system, RNA-binding/electrophoretic mobility shift assays identified a single 125-bp AU-rich element in the 3′ untranslated region that formed hypoxia-inducible RNA-protein complexes.48 Moreover, two proteins of 90-88-kDa and 60-kDa were found to specifically bind this hypoxia stability region element.
Although it is clear from both experimental OIR models and from human pathology (i.e., ROP) that VEGF is required for the formation of retinal vessels, the retinal vasculature in the adult appears to be less dependent on sustained VEGF signaling than other vascular beds. As described below in the “Role of VEGF in the Adult Retina,” robust systemic VEGF neutralization did not result in changes in the inner retinal vasculature though significant vascular regression was noted in many other vessel beds. A clue into the mechanism(s) that may mediate this relative VEGF-independence may come from the unique association of the pericyte with the retinal microvasculature (Box 4-1) and (Fig. 4.2).
BOX 4.1 Role of VEGF in the Adult Retina
The use of immunohistochemistry and mice with a LacZ reporter introduced into the VEGFR2 locus has revealed the expression of VEGFR2 in specific cells throughout the retina.2 VEGFR2 is expressed by the retinal microvascular EC and by astrocytes in the ganglion cell layer, on Müller cells and on photoreceptors. On the other hand, use of mice with a LacZ reporter in the VEGF locus shows VEGF to be produced by scattered cells in the ganglion cell layer and by Müller and amacrine cells. The proximity between the VEGF-producing Müller cells and VEGFR2-expressing photoreceptors is indicative of a paracrine interaction. A possible role for VEGF in the adult retina was addressed by systemically expressing a VEGF-neutralizing soluble Flt-1 via adenoviral delivery. Interestingly, the inner retinal vessels appeared unaltered at the light and ultrastructural level as well as in terms of barrier functions.2 In contrast, there was significant thinning of the inner and outer nuclear layers that was associated with significant apoptosis and with reduced visual acuity. Defects in the RPE layer were also evident in the form of vacuolization, separation form the photoreceptor layers, and breakdown of intercellular junctions. These findings provide strong support for a role for VEGF as a neuroprotectant for photoreceptors and suggest that long-term intravitreal should be conducted with caution.
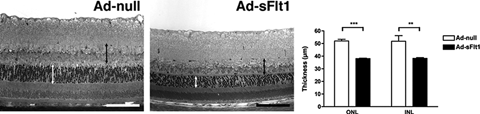
FIGURE 4.2. Decreased retina thickness and loss of visual function in sFlt1-expressing mice. A: Semithin epon sections from retinas of experimental mice expressing sFlt1 for 28 days showed substantial thinning of the INL (black double arrowheads line) and the ONL (white double arrowheads line) (n = 3 for Ad-null and n = 4 for Ad-sFlt1). (Reproduced from Saint-Geniez M, Maharaj AS, Walshe TE, et al. Endogenous VEGF is required for visual function: evidence for a survival role on muller cells and photoreceptors. PLoS One. 2008; 3:e3554. with permission.)
Of all microvascular beds examined, the retinal microvasculature has the highest ratio of pericytes to ECs with one pericyte for each EC.49 The concept that the presence of the pericyte might stabilize the EC is suggested by the fact that pericyte loss is known to precede the development of proliferative diabetic retinopathy (PDR). To gain insight into how the pericyte might serve a stabilizing role, coculture models were used to examine the nature of the interactions between EC and undifferentiated mesenchymal cells (i.e., pericyte precursors). These studies revealed that proliferating EC synthesize and secrete PDGF-B, which stimulates mesenchymal proliferation50 and acts as chemoattractant, directing the migration of undifferentiated mesenchymal cells toward the nascent vessel.51 It was also demonstrated that upon contact with the endothelium, the mesenchymal cells were induced to differentiate toward a pericyte/smooth muscle cell phenotype and to produce VEGF52 via contact-activated TGF-ß1, events that contribute to microvessel stabilization. Thus, it is possible that the VEGF that is locally produced by some pericytes (not all retinal pericytes make VEGF) is not accessible to the sFlt1 used in the experimental models.2 Moreover, the TGF-β that is generated upon local EC-pericyte interactions and the angiopoietin-1 that is produced by pericytes may also contribute to making the retinal vascular the stable vascular bed that it appears to be.
VEGF has similarly been demonstrated to mediate the formation of the choriocapillaris. RPE-specific deletion of VEGF led to the absence of the choriocapillaris, micropthalmia, and loss of visual function.53 Mice heterozygous for RPE-specific VEGF deletion displayed significant defects in the RPE, in spite of normal choroidal vessel formation. The use of mice in which the RPE was null for HIF-1alpha indicated that VEGF expression in RPE was not mediated by hypoxia-induced transcriptional activation. The latter is not a surprising finding, however, since VEGF expression by RPE begins very early during ocular development when, in contrast to retinal vascularization, retinal differentiation has yet to commence.54
Role of VEGF in Ocular Angiogenesis
Under normal circumstances, the blood vessels of the retina, though metabolically active, are quiescent with respect to growth.55 Studies of the labeling index of retinal capillary EC indicate that the turnover time for these cells (i.e., the time it takes to replace the entire population) is in the range of years.55 To put this in perspective, the turnover time of EC in tumor blood vessels is days to weeks.56 However, there are a variety of retinal pathologies that have rapid vessel proliferation as a component including PDR, retinal venous occlusion, and ROP. These pathologies are all characterized by the presence of nonperfused, and therefore hypoxic, retinal tissue as a precedent to the neovascularization. Realizing the central role that ischemia plays in the pathogenesis of the disease process and knowing that local oxygen levels can regulate VEGF expression, a number of laboratories, using various animal models, have examined the role of VEGF in neovascular retinopathies.57
Using a modification of a model of retinal ischemia-induced iris neovascularization,57 VEGF was examined in the cynomologous monkey retina, which had been rendered ischemic by laser occlusion of the branch retinal veins20,58 (Fig. 4.3). Seven to fourteen days following the occlusion, new blood vessels grow on the surface of the iris. (This is similar to rubeosis iridis, which occurs in diabetics and is associated with retinal capillary nonperfusion). VEGF protein and mRNA levels were shown to correlate with the timing and severity of iris angiogenesis (Fig. 4.4). PCR analysis revealed that the retina was producing the 121 and 165 amino acid isoforms of VEGF, the more diffusible forms relative to the third known isoform VEGF189.20 (Primate VEGF isoforms have one amino acid more than the rodent counterparts.) In situ hybridization demonstrated VEGF mRNA expression by the ganglion cells of the retinal ganglion cell layer and by another cell type, possibly amacrine cells, in the inner nuclear layer. This expression pattern is consistent with the concept that expression is regulated by hypoxia because these cells are located in the inner retina, which is the tissue normally served by the occluded vessels.
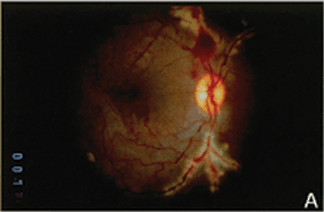
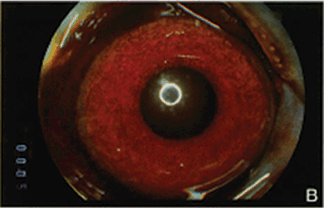
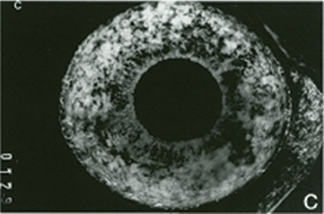
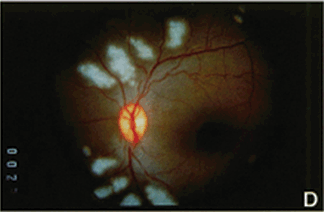
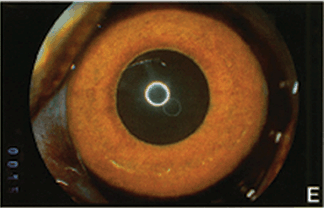
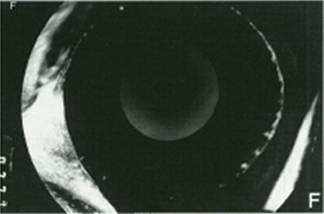
FIGURE 4.3. Experimental iris neovascularization. A: Fundus photograph immediately after laser vein occlusion. B: New vessels are seen on the surface of the iris. Iris neovascularization appears 4 to 7 days after laser vein occlusion, increases, and persists for an average of 24 days, or leads to the development of neovascular glaucoma. C: Fluorescein angiography demonstrates new vessels on the surface of the iris, with a characteristic tortuous pattern, and abundant leakage of fluorescein into the anterior chamber (grade 3). D: Fundus photograph immediately after sham laser, in which laser spots were placed adjacent to the retinal vessels, to produce retinal injury while preserving normal retinal vasculature. E: The iris appears normal 12 days after sham laser. F: Fluorescein angiography of the iris in (E) demonstrates no leakage of fluorescein from the normal iris vessels (grade 0). (Reprinted from Miller et al. Am J Pathol. 1994; 145:574–584, with permission.)
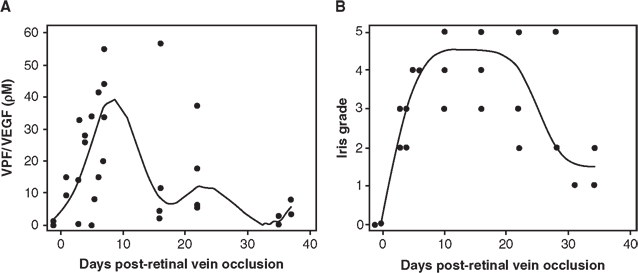
FIGURE 4.4. Aqueous VEGF levels in eyes with iris neovascularization. A,B: VEGF levels in the aqueous (A) and grade of iris neovascularization (B) of four monkey eyes are compared over time after laser vein occlusion as a scatterplot with best-fit curves. Although there is substantial variability between eyes, there seems to be an association between VEGF level and iris neovascularization. VEGF was undetectable in the aqueous before laser, the levels rise as neovascularization develops, and fall as the neovascularization regresses. (Reprinted from Miller et al. Am J Pathol. 1994;145:574–584, with permission.)
A necessary role for VEGF in this process was demonstrated by studies in which neutralizing monoclonal antibodies against VEGF were administered intravitreally at the time of vein occlusion and every other day thereafter for 14 days.59 The vitreous of eyes with PDR has been shown to have levels of VEGF significantly higher than vitreous of eyes without proliferative retinopathy60–62 and neovascular membranes were shown to have VEGF mRNA.62
VEGF has been similarly implicated in the neovascularization that characterizes the ROP. The retinas of neonatal mice incubated in high oxygen and then returned to room air had elevated levels of VEGF mRNA that were localized to Muller cells in the inner nuclear layer.63 A causal link between VEGF expression and the observed neovascularization was demonstrated when the vessel growth was shown to be suppressed by the injection of soluble VEGF receptor chimeric proteins that block VEGF activity.21 Since the neovascularization had been shown to be due to hypoxia-induced VEGF, it was suspected that oxygen levels might be titrated to be high enough to prevent hypoxic damage to the retina but not so high as to suppress the VEGF expression required for normal retinal vascularization. This balance was shown to be effective in the kitten model of ROP64 and is now the focus of clinical trials for the treatment of human ROP. Further insight into the involvement of VEGF in the pathogenesis of ROP came from studies using a cat model of the disease.
VEGF in the Adult
There is no doubt that VEGF plays a critical and central role in vascular development and in pathologic angiogenesis such occurs in proliferative retinopathies and choroidal neovascularization. In contrast, the role of VEGF in the adult is relatively uninvestigated. Virtually all adult tissues express VEGF21 and examination of the expression pattern of VEGF in the adult using a VEGF-LacZ mouse has revealed that VEGF expression is localized to specific cell types in each tissue/organ.65 In the retina, VEGF is expressed by a subset of pericytes and astrocytes52 as well as by the RPE.1,66 In the lung, VEGF is expressed by type 2 pneumocytes,21 and in the kidney, it is expressed by podocytes and some ductal cells.67 It is increasingly clear that VEGF in the adult contributes to the stabilization of the tissue microvasculature. Evidence that the VEGF is signaling the EC of adult vessels is provided by the fact that VEGFR2 is constitutively phosphorylated in a number of tissues including the lung,68 retina,1 and choroid.1
Systematic VEGF inhibition in mice achieved using a small molecule VEGF tyrosine kinase inhibitor or soluble VEGF receptors led to the regression of capillaries in normal tissues as well as in tumors. Among the normal tissues examined, significant vessel loss was measured in pancreatic islets, thyroid, adrenal cortex, pituitary, choroid plexus, small-intestinal villi, and epididymal and adipose tissue after one week of VEGF neutralization.69,70 The extent of vessel loss varied depending on the tissue, with the most significant vessel regression seen in the thyroid (66%). Of interest, all the capillary beds were fenestrated and expressed high levels of VEGF and VEGFR2 and the capillaries that remained in these beds had fewer fenestrations and lower VEGF. Vessels in the thyroid grew back within two weeks after withdrawal of the inhibitors.
A role for VEGF in renal microvascular integrity is similarly indicated by recent observations of human preeclampsia. Overexpression of soluble Flt (VEGFR1) by the placenta has been shown to be correlated with development of the symptoms of preeclampsia, including proteinuria due to loss of glomerular integrity.71 Moreover, many of the features of preeclampsia including hypertension and proteinuria were replicated by systemic expression of sFlt-1 in pregnant rats. These studies not only generated insight into the pathogenesis of preeclampsia but also provided evidence that the most common side effects of systemic anti-VEGF therapy in human, hypertension and proteinuria, were the direct result of VEGF neutralization and supply strong evidence for a role for VEGF in the integrity and function of the adult large and small vessels.72
Similar studies in which VEGF was systemically neutralized in nonpregnant mice revealed a role for VEGF not only in the maintenance of the choroid plexus of the brain, a result that was expected because of its status as a fenestrated vascular bed, but also in the maintenance of the ependymal lining of the ventricles.73 Results of these studies expanded the list of nonvascular VEGF targets and provided a possible explanation for the observation of reversible posterior leukencephalopathy as a rare side effect in patients on anti-VEGF therapy for cancer treatment.74
Nonvascular Targets of VEGF
In addition to its multiple roles on vascular cells, it has become apparent that VEGF targets many nonvascular cells (for review see Ref. 75). VEGFR1 and VEGF2 expressions have been demonstrated on a number of cells types including pneumocytes,76 megakaryocytes,77 dendritic cells,78 monocytes, lens epithelial cells,79 retinal pigment epithelial cells,1 and a variety of neural cells. The preponderance of data supporting a role for VEGF outside of the vascular system is the neural system where there is significant evidence to indicate both neurotrophic and neuroprotective effects on neural cells both in vitro and in vivo.80,81 Neural targets of VEGF include astrocytes and microglia, as well as a variety of neurons (sensory, cortical, hippocampal, etc.), with neurogenic, neuroprotective, and/or neurotrophic effects. Moreover, VEGF has been demonstrated to stimulate the proliferation of neural progenitors in parts of the brain capable of neurogenesis such as the hippocampus, the olfactory, and the subventricular zones. Most recently, a conditional transgenic system was used to suppress VEGF signaling at specific site in the brain where interneurons traverse on their way to be incorporated into the existing olfactory circuits. Blockage of VEGF that is expressed by a specific type of resident olfactory neurons significantly reduced dendrite development in incoming subventricular zone–born neurons. These results point to a role for VEGF in the maturation of adult-born neurons in the brain.82
Of significance for this publication is the presence of VEGFR2 on retinal astrocytes, Müller cells, photoreceptors, and RPE.1,2 These observations coupled with the production of VEGF by the Muller cells and the RPE themselves point to the presence of both paracrine (Müller-photoreceptor; RPE-choriocapillaris) and autocrine (Müller cells, RPE) in the adult retina (see Boxes 4-1 and 4-2).
BOX 4.2 Role for RPE-derived VEGF in the Maintenance of the Adult Choriocapillaris
RPE produces VEGF early during retinal development, and its production continues into adulthood.1 On the other hand, the endothelium of the choriocapillaris expresses relatively high levels of VEGFR2 and biochemical analysis reveals that VEGFR2 is constitutively phosphorylated.1 Since this VEGF signaling is occurring in the absence of active angiogenesis, VEGF must be serving a role other than stimulating vessel growth. RPE in vivo produced VEGF120 and VEGF164, but no detectable VEGF188.1 Since VEGF188 is not freely diffusible, and since VEGF produced by RPE would need to diffuse through Bruch membrane to reach the target choriocapillary EC, the absence of VEGF188 production is logical. Taking advantage of the restricted VEGF isoform production by RPE, mice that had been engineered to express only VEGF188 were used to examine the effect of reduced VEGF signaling between the RPE and the choriocapillaris. Although the RPE-choriocapillaris of mice that produce only VEGF188 were normal though 4 months of age, there was significant pathology in the choriocapillaris of 6-month-old VEGF188 mice, including the replacement of the choriocapillaris with large, nonfenestrated vessels and abnormalities in the RPE such as vacuoles, separation from the photoreceptor layers, and breakdown on intercellular junctions (Fig. 4.5).4 The defects worsen with age and lead to areas of RPE loss and reduced visual function. These findings indicate that RPE-derived VEGF is essential for the maintenance of the choriocapillaris and the RPE themselves and suggest that chronic use of anti-VEGF therapies should be applied with caution and with attention to possible unwanted side effects.
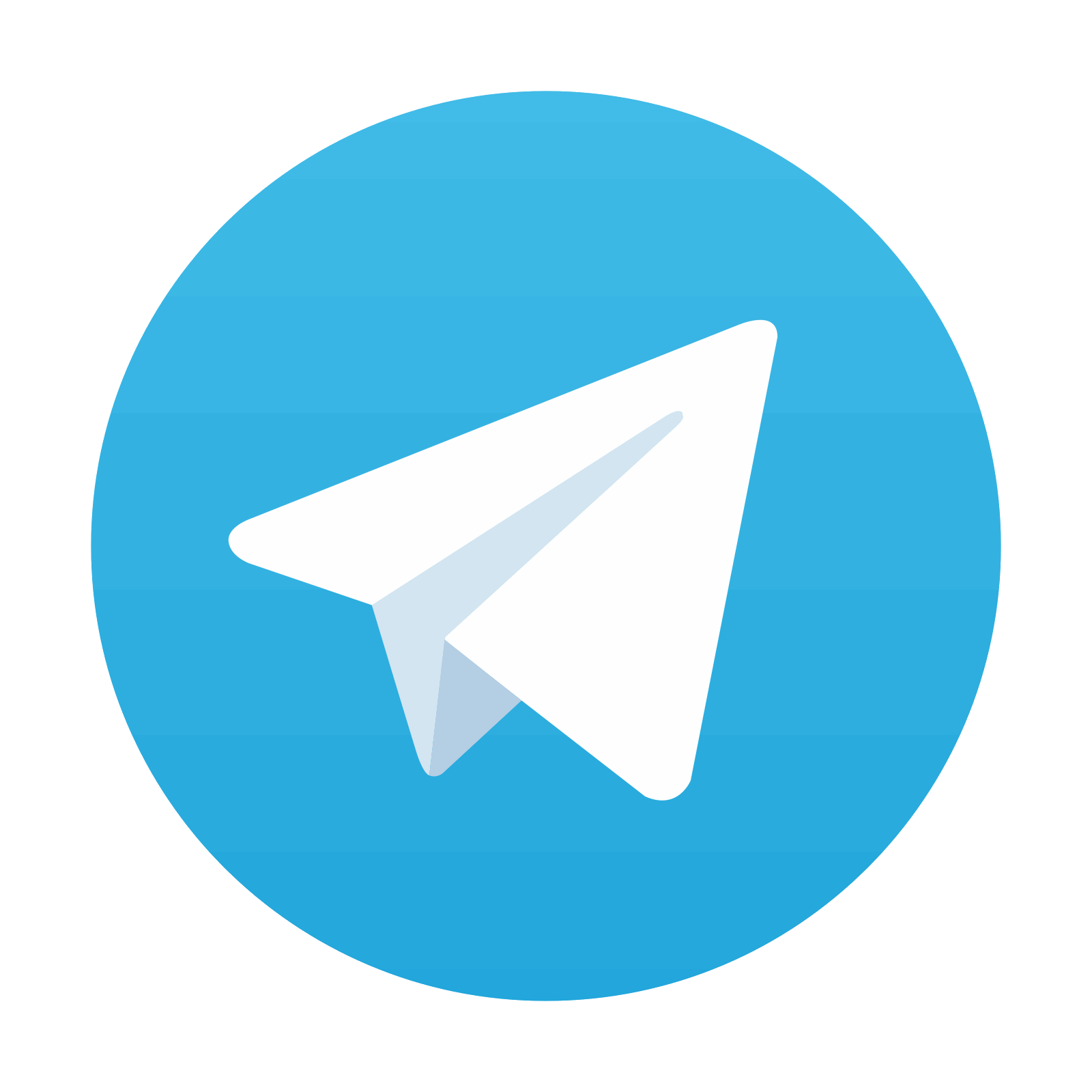
Stay updated, free articles. Join our Telegram channel

Full access? Get Clinical Tree
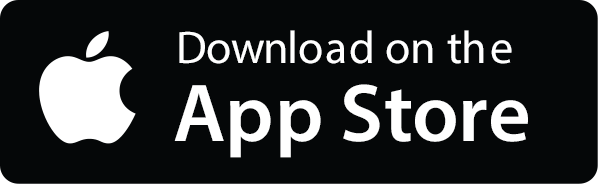
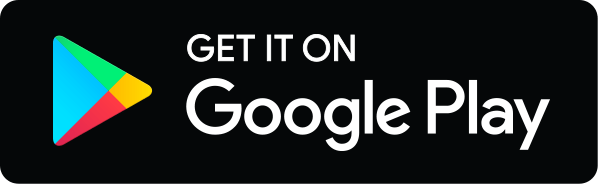