4 Phase-Variance Optical Coherence Tomography
Angiography of the retinal and choroidal vasculature is a valuable diagnostic tool and is essential to the evaluation of many vision-threatening diseases, including age-related macular degeneration (AMD), cystoid macular edema, diabetic retinopathy, posterior uveitis, and retinal vascular occlusion. Fluorescein angiography (FA) is the gold-standard modality for imaging of the retinal circulation, whereas indocyanine green angiography (ICGA) is used primarily for its visualization of the choroidal vasculature. 1 However, each of these modalities requires intravenous dye infusion, is time-consuming, requires significant operator skill, and carries the risk of both minor and major adverse events. 2 , 3
Dye-based angiography is also limited in its imaging capabilities. Leakage of dye from the vessels can obscure vascular detail, particularly when imaging the fenestrated vessels of the choriocapillaris. Compared with fluorescein, indocyanine green dye is more protein-bound and has higher absorption (~ 805 nm) and emission (~ 835 nm) wavelengths. 1 These properties reduce dye extravasation from intact choroidal vessels and improve signal penetration, both of which enable enhanced visualization of the choroidal vasculature. However, its ability to resolve the microvasculature, particularly the choriocapillaris, remains limited. 4 , 5
As an alternative to dye-based modalities, optical coherence tomography (OCT) provides highly detailed, noninvasive imaging of the retinal architecture, and recent developments have enabled the production of uniquely informative data. Machines capable of performing both scanning laser ophthalmoscopy and OCT permit the combined use of microperimetry and OCT to assess precisely the relationship between visual function and retinal structures. 6 The application of adaptive optics uses wavefront analysis to facilitate correction of higher-order optical aberrations and increase scan resolution. 7 The development of faster and more sensitive imaging techniques (e.g., Fourier-domain systems, such as spectral-domain (SD) and swept-source OCT), along with progress in OCT data processing, has enhanced the ability of OCT to create retinovascular angiograms. 8 , 9 , 10 , 11 , 12 , 13 , 14 , 15 , 16 , 17 , 18 , 19 , 20 , 21 , 22 , 23 , 24 , 25 , 26 , 27 , 28 , 29 , 30 Multiple approaches, each with certain advantages and limitations, have been developed for this purpose. Phase-variance OCT (pvOCT) is a noninvasive technique that creates three-dimensional representations of the retinal and choroidal vasculature by analyzing motion-related changes in OCT signal phase over time that occur between consecutive B-scans. 24 , 25 , 26 , 27 , 28 , 29 , 30
4.1 Basic Principles
Optical coherence tomography of the eye relies on the principles of optical interferometry. 31 A beam of low-coherence, near-infrared light is split into two arms: a reference signal that is projected toward a reference mirror and a sample signal of equal optical path length that is projected onto the sample tissue. As the sample signal passes through tissue, some portion of it is scattered, and the portion that is backscattered is combined with the reflected reference signal. In Fourier-domain OCT systems, the resulting spectral interference pattern is Fourier-transformed to produce a depth-resolved scattering profile that is then used to generate a structural image of the sample tissue.
Although OCT can produce images of the retinal and choroidal blood vessels, standard visualization of the B-scan (intensity of back-scattered light) is unable to highlight flow through the retinal structures; however, the reconstructed OCT B-scan contains phase information that can be analyzed to detect motion. When the sample beam is reflected by medium that is moving, the resulting phase value varies over time. 32 Phase-variance OCT is a technique that interprets phase shifts between series of consecutive B-scans to detect areas of motion. 24 , 25 , 26
An A-scan is a one-dimensional depth-scattering profile taken at a single location, and a B-scan is a two-dimensional cross-section composed of multiple, consecutive A-scans taken over a range of transverse locations. In the most common pvOCT technique, multiple successive OCT B-scans (BM-scan) are acquired consecutively at the same location, and this process is repeated over a spatial region of the retina to obtain a volumetric scan. 24 Interpretation of the statistical variance of the phase shifts occurring between the B-scans can be used to identify areas of mobility (i.e., blood flow) that contrast with the relatively static retinal tissue. 24 , 25 , 26 The ability to determine the phase contrast between successive B-scans is dependent on the image acquisition speed of the OCT system, and improvements in acquisition speed should reduce motion artifact and increase the potential imaging size. 25
An alternative method for visualizing motion is Doppler OCT. Doppler-based methods identify motion by detecting changes in the signal occurring in the axial direction of the imaging beam; pvOCT is able to characterize flow occurring in both axial and transverse planes. 25 Furthermore, because Doppler-based methods rely on detecting signal scattering that occurs on successive A-scans, increasing the image acquisition speed allows less time for scatter changes to occur and the ability to detect slow flow is reduced. 33 , 34 , 35 In contrast, pvOCT can identify motion over a wide dynamic range of flow rates and, as stated previously, benefits from improved acquisition speed. 25
4.2 Technique
4.2.1 Equipment
A primary advantage of pvOCT over other methods of OCT-based angiography is the ability to use conventional SD OCT machines to acquire the data required for phase-variance imaging. The pvOCT technique relies on analysis of phase-specific data that are normally collected, but not used, during standard SD OCT imaging; however, although no hardware modifications are necessary, specialized software-based imaging protocols must be applied to capture the entire raw data set and perform BM-scans in the appropriate fashion. Average volumetric scan times are less than 5 seconds, and irradiance levels conform to the established American National Standards Institute (ANSI) standards. 27 , 28 The generation of images comparable to those produced on custom OCT machines has been demonstrated using a commercial SD OCT platform. With existing technology, the approximate axial resolution ranges from 3 to 4.5 µm, and lateral resolution ranges from 10 to 15 µm. 30
4.2.2 Image Processing
Once raw scan data are collected, phase information must be extracted and analyzed. Acquired OCT data are initially processed using standard procedures, and bulk axial motion is calculated and corrected for each location to separate vascular flow from gross eye motion. 26 , 27 Intensity thresholding reduces signal noise, and the phase differences between sequential B-scans are extracted for phase-variance calculations. 28 This process is repeated over the entire scan area to yield a three-dimensional image. Volumetric data are segmented according to depth, and pseudocolor depth coding may be applied to highlight depth position of vascular networks. 28 , 30
4.2.3 Image Format
Phase-variance OCT images may be viewed as standard two-dimensional tomograms or as en face projections. 28 An en face projection may represent a single depth layer of the retina or choroid, or multiple depth layers may be overlaid, each with an assigned pseudocolor (Fig. 4.1). Images of specific depth layers may be produced to evaluate a particular vascular network (e.g., the Sattler versus the Haller layer of the choroid). Color-coded pvOCT B-scans may also be displayed over standard OCT tomograms to produce composite B-scan images in which color indicates areas of flow (Fig. 4.2).


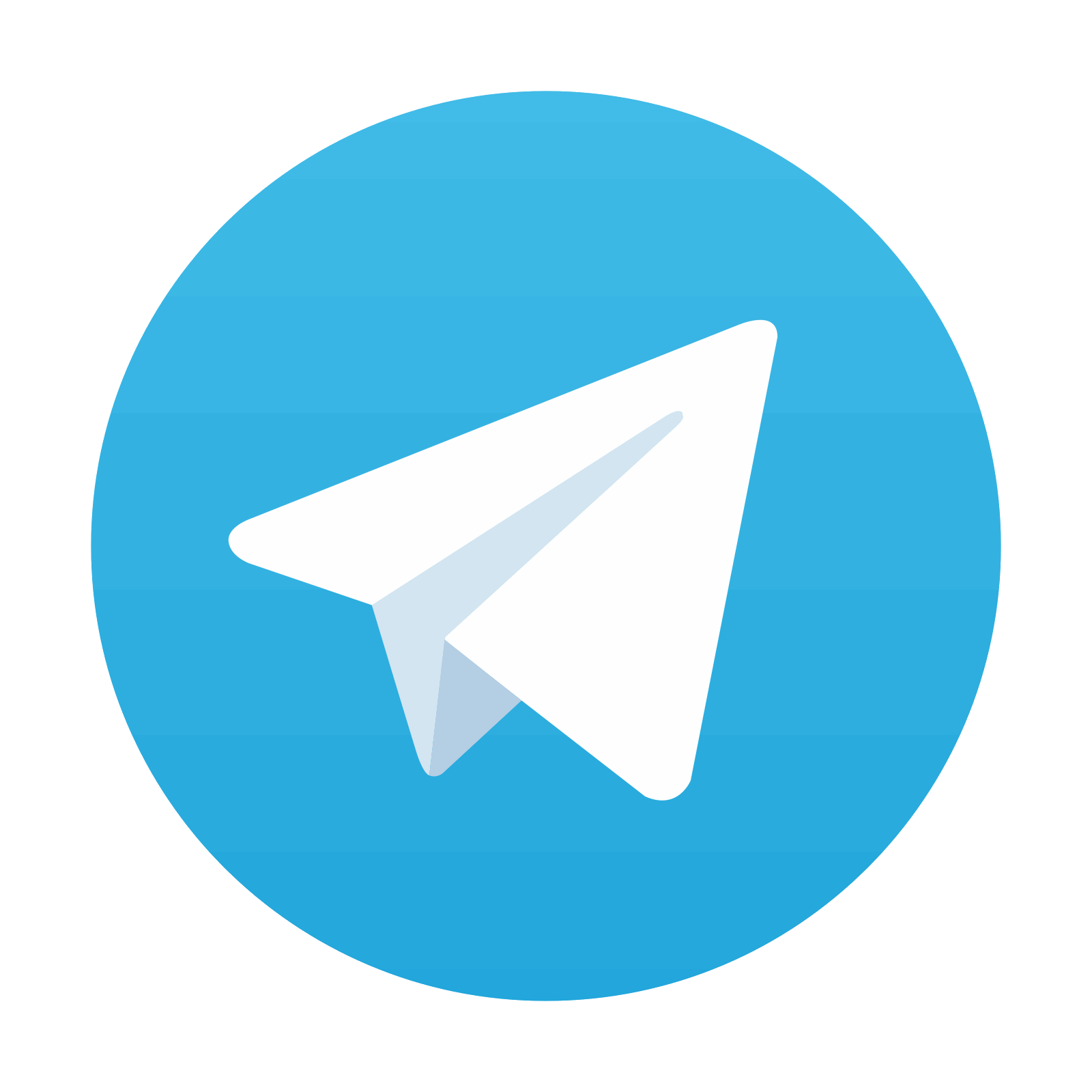
Stay updated, free articles. Join our Telegram channel

Full access? Get Clinical Tree
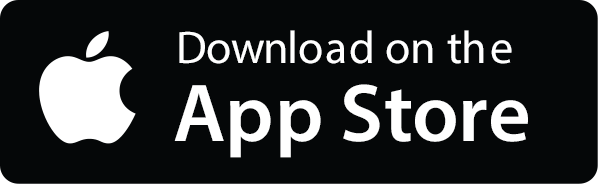
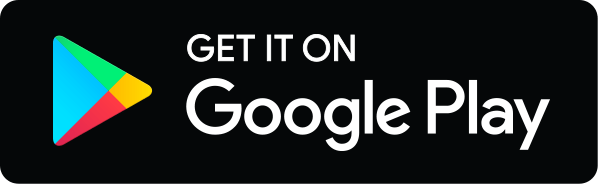
