5 Swept-Source Optical Coherence Tomography
5.1 Background
Optical coherence tomography (OCT) was first applied in ophthalmology by Huang et al in 1991 to image the retinal layers. 1 Since then, OCT technology has been advanced from time domain (TD) to Fourier domain (FD), providing a noninvasive imaging method with high scanning speed and high resolution to visualize the structures inside the eye. The unique cross-sectional view of the eye enables not only morphologic but also quantitative assessment of the structure, which facilitates the diagnosis and monitoring of eye diseases. This chapter summarizes the applications of the latest generation of OCT, swept-source optical coherence tomography (SS OCT) for assessment of both the anterior and posterior segments of the eye.
5.2 Optical Coherence Tomography: From Time-Domain to Swept-Source
Low-coherence interferometry, the fundamental principle of OCT, detects and analyses the interference signals generated by superimposing backscattered light from the tissue of interest and that from a reference path to obtain optical intensity and depth information. The information is then translated into a color-coded or gray-scaled image to reveal the internal structures of the tissue. In TD OCT, a mobile reference arm mirror is used to ensure that the path lengths of both sample and reference arms are the same or nearly the same to allow interference between light reflected from the two arms, so that optical intensities of structures with varied depths can be sequentially documented while the reference mirror goes through the whole depth of the tissue. Because of the mobile reference mirror, the image acquisition time is rather long, which limits the image sampling density and makes TD OCT prone to motion artifacts. Additionally, the power of the light source of TD OCT is restricted by the inherited long image acquisition time and safety standards of total light exposure to the eye. Therefore, image resolution of TD OCT is also limited.
With the advent of FD technology, including spectral-domain OCT (SD OCT) and later the SS OCT, the axial image resolution and image acquisition speed of OCT have been significantly improved over the TD method. In SD OCT, the depth information is acquired without mechanical movement of the reference mirror, which significantly increases the scan speed. 2 , 3 , 4 Although the stationary reference mirror causes mismatch of path lengths, the light reflected from the structures with different depths will still interfere with the light from reference arm, generating interference signals coded with different beat frequencies. The beat frequency will be higher if the path lengths have a greater mismatch and vice versa. Then the interference signals with different beat frequencies will be dispersed by a spectrometer. After Fourier transformation of the separated interference signals, the optical intensity and depth information within the whole tissue will be determined simultaneously. This method increases the scan speed up to 100 times compared with TD OCT. However, the grating and detecting systems of SD OCT sample signals with higher frequencies in a nonlinear manner, so the higher frequency signals cannot be separated to the same extent as lower-frequency signals can, which restricts the ability of SD OCT to visualize deeper structures. Despite methods such as enhanced depth imaging and image averaging having been combined with SD OCT for better resolution of imaging deeper structures, SD OCT can produce high-resolution images of the structures only at either the proximal or the distal limit of the tissue, but not both at the same time.
Swept-source OCT uses a short cavity-swept laser as light source, which has a narrow bandwidth and is tunable to emitted light with different frequencies. When the tissue is scanned by the laser across a range of frequencies in an orderly and rapid fashion, the spectral interference patterns are generated and then detected by a photodiode in almost real time. With such a process, the interference signals from different depths are coded by time rather than by frequency. Afterward, the spectral interference pattern will be Fourier-transformed to give a depth profile of the sample’s reflectance, which is similar to what happens in SD OCT. By using a more complicated light source and simple detection system, SS OCT gains additional advantages over TD OCT, including better sensitivity with imaging depth, higher imaging resolution, longer imaging range, and even shorter imaging acquisition time. All the advantages allow us to see into the eye in a better and deeper way, providing better understanding of the eye diseases we are currently treating.
5.3 Applications of Swept-Source OCT
5.3.1 Glaucoma
Since the first commercially available model, OCT has been adopted for the detection of glaucomatous damage and progression. Now, with SS OCT, more information can be readily obtained.
Glaucoma, a chronic degenerative optic neuropathy, is characterized by progressive loss of retinal ganglion cells and remodeling of the optic nerve head (ONH). Therefore, quantitative assessment of the peripapillary retinal nerve fiber layer (RNFL) and displacement of lamina cribrosa (LC) is of great interest to glaucoma specialists. SD OCT is capable of measuring RNFL thickness around the ONH region with low test-retest variability; however, the wider imaging range provided by SS OCT can cover a larger retinal area to avoid missing subtle RNFL defects, which could be located away from the optic disc margin and even close to the macular region. This capability not only indicates involvement of the macular region in glaucoma development, but it also offers an opportunity to understand the natural history of glaucomatous damage to the RNFL from an early disease stage.
Visualization of the LC is feasible with SS OCT. 5 , 6 Lopilly Park et al reported that the lamina thickness measured with SS OCT in primary open-angle glaucoma and normal-tension glaucoma eyes was significantly decreased compared with normal controls. 7 Yoshikawa et al studied the change of displacement of LC using SS OCT in glaucoma patients after surgical reduction of intraocular pressure (IOP) and concluded that changes in LC may correlate with the IOP level and disease severity. 8 In contrast to SD OCT, SS OCT permits simultaneous imaging of the retina, choroid, and LC with high sensitivity in one scan, so that landmarks, like the Bruch’s membrane opening, and delineation of lamina will be determined reliably to generate quantitative data over time for further understanding of the process of ONH remodeling and its significance in glaucoma progression.
In addition, SS OCT has been used to image the choroid and sclera to study their roles in glaucoma. Usui et al measured choroidal thickness in highly myopic eyes with and without normal tension glaucoma using SS OCT and reported that choroidal thickness was significantly thinner in highly myopic eyes with normal-tension glaucoma compared with age- and refractive error–matched controls. 9 After measuring the subfoveal sclera using an SS OCT system, Lopilly Park et al found that subfoveal scleral thickness in normal-tension glaucoma eyes was thinner than in primary open-angle glaucoma eyes. 7
Application of SS OCT in glaucoma is not limited to the posterior part of the eye. Anterior-segment imaging has also been improved by enabling three-dimensional imaging and increasing sampling density. Identification of scleral spur is crucial for quantification of angle status (Fig. 5.1). A study by McKee et al assessed the visibility of scleral spur in SS OCT images of the anterior-chamber angle. Using high-density raster scan (64 A-scan/mm), visibility ranged from 95 to 100% for the superior, inferior, nasal, and temporal quadrants. 10 Although currently the scleral spur needs to be determined manually, measurement reproducibility was found to be high by Liu et al. 11 Compared with gonioscopy, SS OCT provides the feasibility to examine the angle in different lighting conditions and the possibility of quantification of the extent of angle closure. Additionally, the contribution of the iris to angle closure has been studied using SS OCT. Mak et al measured the iris and anterior chamber volume in open-angle and angle-closure eyes before and after dilation and found that the iris volume decreased after pupil dilation and the degree of reduction was less in eyes with a smaller anterior-chamber volume. 12 Therefore, both iris volume and anterior chamber volume contribute to angle closure. Lai et al also used SS OCT to visualize and measure the area and degree of peripheral anterior synechia. 13 The authors concluded from the low measurement variability that SS OCT would be useful to detect peripheral anterior synechia progression in relation to IOP increase and glaucoma progression (Fig. 5.2).


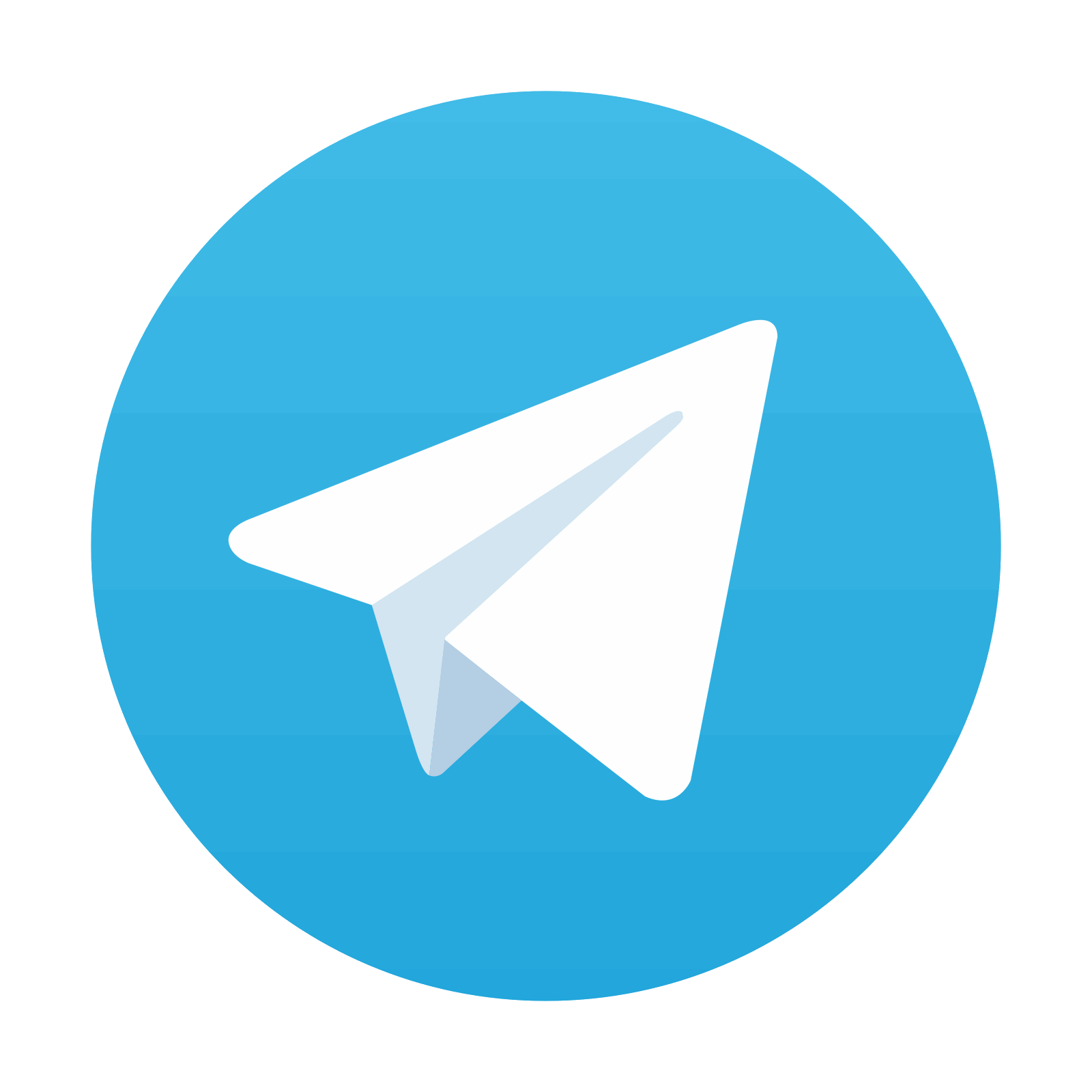
Stay updated, free articles. Join our Telegram channel

Full access? Get Clinical Tree
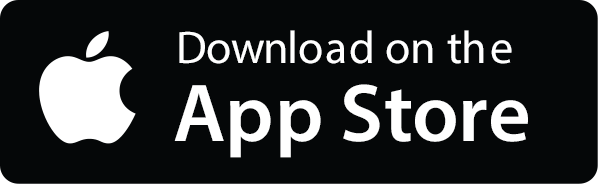
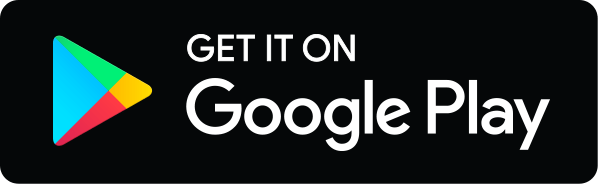
