!DOCTYPE html PUBLIC “-//W3C//DTD XHTML 1.1//EN” “http://www.w3.org/TR/xhtml11/DTD/xhtml11.dtd”>
Anti-VEGF Treatment in Retinopathy of Prematurity
Retinopathy of prematurity (ROP) affects 4,000 to 16,000 premature infants in the United States per year (1,2) and is a leading cause of childhood blindness (3–9). Incomplete retinal vascular development predisposes the premature infant to a vasoproliferative disorder characterized first by slowed or even arrested blood vessel growth followed by dysregulated vascular development into the vitreous. When retinal vascular development restarts, it may proceed along the normal pathway or descend into pathologic changes with accompanying gliosis, vitreoretinal traction, and even retinal detachment (10).
To accurately and reproducibly describe the vascular changes associated with ROP, the International Classification of Retinopathy of Prematurity has defined ROP characteristics such as location (zone), extent (clock hours), severity (stage), and modifiers (plus, pre–plus, and aggressive posterior disease) (11,12).
Screening for ROP, as stipulated by the Joint Statement guidelines, is currently indicated for infants with a birth weight of 1,500 g or less or a gestational age of 28 weeks or less, as well as infants between 1,500 and 2,000 g or gestational age of more than 28 weeks with unstable clinical courses who are identified as “high risk” by their attending pediatrician or neonatologist (13,14). The goal of screening is to prevent blindness arising from ROP through the timely intervention of therapy that will induce regression of disease and result in continuing retinal vascular development.
The pathogenesis of ROP is related to interruption of the normal pattern of retinal vascular development with ensuing pathologic changes. Most studies done on normal retinal vascular development have been done in animals, but there are differences between humans and other species (see Chapters 5 and 42). In normal human retinal development, the initial vasculature is believed to occur by vasculogenesis beginning in the 16th week of gestation and continuing through at least 22 weeks’ (15) gestation. From that time, how vessels develop in the human infant is incompletely understood. However, based on animal models, it is believed to occur by a process of angiogenesis, extending the vasculature from about zone I to the nasal ora serrata by the 8 months of gestation and to the temporal ora serrata 1 to 2 months later (10). Numerous cell–cell interactions appear important as are growth factors. Important cell types include astrocytes (16), ganglion cells, endothelial precursor cells, endothelial cells, glial cells, and growth factors including vascular endothelial growth factor (VEGF), stromal-derived factor-1 and its receptor CXCR-4 (15), glia-derived trophic factor, and retinal ganglion cells (RGCs)–derived trophic factor. In mice, VEGF gradient is believed to drive the initial retinal angiogenesis (17). (Additional discussion about the development of the retina and its vasculature is given in Chapters 1, 5, and 8.)
During the second trimester of gestation, vascular development is driven by vasculogenesis. Vasogenic growth factors increase as a result of relative intrauterine hypoxia and increased metabolic activity associated with neuronal retinal differentiation and include stromal-derived factor (15) and its receptor CXCR-4 and VEGF, produced from RGC and astrocytes (18). The third trimester is believed to be associated with a transition from vasculogenesis to angiogenesis for retinal vasculature development. Angiogenesis is characterized by endothelial sprouting from an existing vessel and proliferation and (in animal models) is often stimulated by VEGF as well as recruitment of mural cells from other factors during blood vessel creation (19). RGCs migrate radially from the optic nerve to the ora serrata, expressing VEGF in relation to local tissue hypoxia gradients (17). A leading edge of endothelial tip cells directs vessel growth development (20), utilizing tractable filopodia, which in turn are patterned by the response of the numerous guidance receptors they express (VEGFR, Unc5b, and NRP1) (21–23). Trailing endothelial stalk cells then establish a fledgling vascular network. Important is the role of VEGFC and VEGFR3 in the process of endothelial tip to stalk cell conversion through regulation of notch (24). Retinal vascular maturation is modulated by a complex interaction of mural cells (which mature into pericytes and smooth muscle cells) and apoptosis. Ultimately, it is believed that the dual, simultaneous process of vascular maturation and involution results in the promotion and maturation of vessels (25) while curtailing the development of others (26).
The disruption of normal retinal vascular development by ROP has been characterized by two phases (27): (i) phase I, delayed physiologic retinal vascular development and vasoattenuation in regions where oxygen is not regulated (see also Chapters 38, 42, and 43), and (ii) phase II, vasoproliferative intravitreal neovascularization. It is believed that when a preterm infant is born, there is insufficient insulin-like growth factor 1 (IGF-1) and other factors that are usually supplied through the placenta (18). This slows retinal vascular development. In addition, a number of events related to birth and to postnatal insults including oxygen fluctuations, inflammatory stimuli, and oxidative stress occur to slow physiologic retinal vascular development further and cause aberrant growth of blood vessels into the vitreous. Birth also may expose the infant to relative extrauterine hyperoxia although not at the levels initially thought based on the first descriptions of ROP and the models ensuing (28,29) (see Chapter 42). (The oxygen concentration in the tissue is complicated because fetal hemoglobin has greater affinity for oxygen than does adult hemoglobin and there can be variable effects based on shunting and dilution of blood and where the measurements are taken.) Early models provide evidence that the angiogenic factor, VEGF, is down-regulated by high oxygen (30–34) and does not promote physiologic retinal vascular development. More relevant models suggest that oxygen stresses of preterm infants today cause other events that lead to increasing levels of VEGF that first delay physiologic retinal vascular development and then cause disordered divisions of endothelial cells causing cells to grow outside of the retinal plane and proliferate as intravitreal angiogenesis in phase II rather than as intraretinal neovascularization in physiologic retinal vascular development (35,36). The ischemia from the avascular retina then combined with the increasing metabolic demand of the developing retina results in tissue hypoxia that induces neurons and astrocytes (37,38) to overexpress angiogenic factors and have increased signaling of angiogenic pathways involving, for example, VEGF and EPO (18,39,40). Stage 3 ROP occurs at about 32 to 34 weeks gestational age and is characterized by pathologic retinal and extraretinal neovascularization (30–37). Extrapolating, it has been suggested that zone I disease may stem from aberrant vasculogenesis, whereas zone II disease is angiogenesis dependent (41,42). Vitreous samples taken from eyes with stage 4 ROP requiring surgery demonstrate a nearly 50-fold increase in VEGF concentrations versus to control eyes with levels highest in those eyes also with plus disease (43). It is clear from the above summary of retinal vasculature maturation and pathogenesis resulting in ROP that there are numerous potential targets to interrupt the development and progression of ROP.
The goal of treatment in ROP is to prevent blindness while maximizing all visual functions and minimizing local and systemic side effects. Despite the proliferation of emerging pharmacotherapy, laser photocoagulation of the avascular retina remains the gold standard treatment of ROP requiring therapy (44).
Treatment indications are based on the results of two large National Eye Institute (NEI) studies: the Cryotherapy for Retinopathy of Prematurity (CRYO-ROP) trial and the Early Treatment for Retinopathy of Prematurity (ETROP) trial (11,45). The net result of these studies is that ablative therapy is now indicated for type 1 ETROP: zone I, stage 3 disease; zone I, plus disease; and zone II, stage 2 or 3 with plus disease (45,46), replacing the earlier threshold criteria from the CRYO-ROP trial (see Chapter 38).
Cryotherapy
Cryotherapy was reportedly first used for ROP in 1972 (47) but moved to the forefront of ROP treatment after the CRYO-ROP study: a large, multicenter, clinical trial in which infants with advanced ROP (termed “threshold ROP”) were either observed or treated with cryoablation of the avascular regions of their retina. Primary outcome measures were reduction of both abnormal retinal anatomy (retinal detachment or folds) and poor visual acuity (20/200 or worse) (48,49). At the time of the CRYO-ROP trial, threshold ROP was defined as stage 3 ROP in zone I or II occupying at least 5 contiguous clock hours or 8 noncontiguous clock hours of retina, with four quadrants of plus disease (48). Though initially conjectured as the level of disease, which had a 50% chance of spontaneous regression, ROP of this severity would eventually become defined as warranting ablative treatment (50). Ten-year data of the CRYO-ROP trial demonstrated that patients with threshold disease receiving treatment were 18% less likely to be legally blind and did not have significantly worse contrast sensitivity or peripheral vision loss (51,52). The effect on structural outcomes (posterior retinal fold or worse) and visual outcomes (vision better than 20/200) was maintained at 15-year follow-up (53) with adverse outcomes (posterior retinal fold or worse) reduced by 50% (54). Presently, the American Academy of Ophthalmology advocates generally that cryotherapy should be limited to those cases where laser is unavailable (55).
Laser Photocoagulation
Laser photocoagulation of avascular retina was demonstrated to have even better outcomes than cryotherapy, and cryotherapy has now largely been relegated as a secondary therapy for management of type 1 ETROP (55–57). A large prospective randomized clinical trial following 52 infants with threshold disease demonstrated that laser-treated eyes were nearly seven times more likely to demonstrate a visual acuity of 20/50 or better at an average of 5.8 years after treatment (58). Laser-treated patients also demonstrated an average of 2.03 diopters less myopia than their cryoablated counterparts. Photocoagulation’s advantages include ease of use and fewer systemic and local complications (58).
Proper laser photocoagulation is applied within 72 hours of diagnosis (45,59) and utilizes diode laser delivered with indirect ophthalmoscopy with burns spaced one-half burn width apart to achieve retinal whitening of the avascular peripheral retina with sparing of the ridge (60). Near-confluent laser treatment yields better outcomes than scattered patterns (60,61); thus, the retina should be inspected for “skip areas” immediately after treatment and 1 week after treatment. Use of digital fiberoptic wide-angle photography following laser photocoagulation is useful in identifying skip areas that may not be appreciated with ophthalmoscopy. Refractory neovascularization and persistent plus disease are indications for repeat treatment. Complications of laser treatment include anterior segment ischemia, cataract, and burns of the cornea, iris, or tunica vasculosa lentis (62,63).
The ETROP study demonstrated that treatment earlier in the disease course yielded improved outcomes (64). Specifically, laser ablation was recommended for any stage of ROP in zone I with plus disease, stage 3 ROP in zone I with or without plus disease, and stage 2 or 3 ROP in zone II with plus disease in at least two quadrants (45). The 2-year data demonstrated improved structural outcomes (defined as absence of retinal folds or detachment) in eyes receiving treatment for these earlier stages of the disease (65).
There is, unfortunately, no panacea for all stages of ROP, and response to laser ablative therapy varies at least with ROP location. Fifty-seven eyes with zone I ROP were treated with laser photocoagulation, and after 3 months of follow-up, 35% of eyes with anterior zone ROP and 100% of eyes with posterior zone I ROP had unfavorable anatomic outcomes (66). This trend was also noted in the CRYO-ROP, STOP-ROP, and ETROP trials, which demonstrated similarly unfavorable results after cryoablation or laser ablative therapy in 42.1% in zone I eyes versus 27.5% for zone II eyes (41). One hypothesis for this is that at the time of laser ablation, the aforementioned phase I of retinal vascular development has already been completed, and thus, zone I vessels are insensitive to VEGF and consequently unaffected by peripheral retinal ablation (41). However, the generation of zone II vessels (central and peripapillary capillaries as well as peripheral retinal vessels) remains highly reliant upon VEGF-mediated angiogenesis (41,42,67) and this may be why it appears to exhibit better anatomic and visual outcomes with laser or cryotherapy.
More recent treatment regimens have even examined more aggressive laser application. Using the ETROP guidelines and infrared diode laser photocoagulation, treatment of the entire area of avascular retina as well as areas posterior to the active ridge (with avoidance of blood vessels) resulted in a favorable anatomic outcome in 92% of cases (68), although this is not the approach broadly accepted as the treatment standard. Also, aggressive and potentially repeated laser treatment of eyes with zone I ROP with plus disease or abnormal vascular shunting (with or without neovascularization) demonstrated complete disease regression without vitreoretinal distortion in 84% of eyes with an additional 4% of eyes demonstrating complete regression with some vitreoretinal distortion (69). The authors conclude the propensity for zone I aggressive ROP patients to achieve good visual outcomes is predicated upon early detection and aggressive treatment.
Nonetheless, retinal detachment still occurs in zone I and II disease. Vitreous surgery is recommended for progressive stage 4A ROP as this represents the final stage at which meaningful vision can be salvaged and since the natural course of progressive stage 4A disease is eventual progression to stage 5 disease (49,70). Surgery for stage 5 ROP is considered in individual cases because visual prognosis is generally quite poor (61,71).
Anti-VEGF
Bevacizumab (Avastin, Genentech/Roche) is a 150-kDa, full-length recombinant humanized monoclonal IgG1 antibody that binds all isoforms of VEGF-A (72). It was previously believed that the full-length bevacizumab antibody administered by intravitreal injection was incapable of transiting the intact retina and entering the systemic circulation (73). At that time, clinical testing was initiated in a number of clinics to test the efficacy of inhibiting VEGF bioactivity in ROP (74), a disease found associated with increased VEGF in part because it was believed that anti-VEGF agents injected into the vitreous would not reach the systemic circulation (75,76). Most of these studies done were without controls. With greater evidence, it was found that anti-VEGF agents delivered into adult vitreous caused effects in the fellow eyes of patients with diabetic retinopathy (77) and other conditions (77–80). It remains unknown what drug dose would be useful in the infant since there are many reasons why lower or higher drug doses may be effective in ROP.
Clinical series provided support of anti-VEGF in ROP. Reports demonstrated that bilateral 0.75 mg intravitreal bevacizumab injections combined with laser photocoagulation induced prompt resolution of aggressive stage 3, zone I ROP with no adverse events at 3 months (81). The 0.75 mg bevacizumab intravitreal injection also resulted in complete regression of fibrovascular proliferation and neovascularization at 1 week in another infant with aggressive posterior ROP who developed anterior segment neovascularization and anterior fibro-vascular proliferation secondary to anterior segment ischemia following laser photocoagulation (82). One of the few postmortem histopathologic analyses, from a premature infant who received bilateral injections of 0.5 mg of bevacizumab for zone I, stage 2+ ROP at gestational age of 31 weeks with repeat injections at 42 weeks (for zone II, stage 3+ ROP), found regression of neovascularization with intraretinal VEGF expression as well as apparently normal retinal morphology (83). The authors concluded that 0.5 mg of intravitreal bevacizumab represented an appropriate dose for treatment of ROP (83). The authors stated the infant’s death was unrelated to bevacizumab administration (83).
A larger retrospective case series of 11 infants injected with 0.625 mg bilaterally for stage 3, zone I or posterior zone II ROP demonstrated regression of neo-vascularization and continued (nonpathologic) vascularization of the peripheral retina, with a mean follow-up of 48.5 weeks (84). The authors noted no systemic complications (84). In a retrospective chart review, peripheral retinal vascularization was also noted to proceed normally in 15 patients with stage 3 ROP who received either combination laser photocoagulation and intravitreal bevacizumab (0.5 mg) or laser photocoagulation alone (85). Combination therapy with laser photocoagulation and bevacizumab resulted in more rapid regression of plus disease and peripheral retinal vascularization; however, peripheral retinal vascularization proceeded normally in both groups (85). No systemic or ocular complications were noted (85). Bevacizumab has also been used as an adjunct to vitreoretinal surgery for ROP patients to improve retinal visualization intraoperatively (86). In these series or case reports, no controls or definition of normal was provided. Also, infants treated were of varying postgestational ages and sizes, and therefore, the studies cannot be generalized necessarily to infants of young postgestational ages and small weights. The studies offer hope for a treatment but cannot give complete information regarding safety or efficacy compared to another treatment, such as laser.
Recently, the bevacizumab eliminates the angiogenic threat of retinopathy of prematurity (BEAT-ROP) trial reported its primary outcomes (76). Eligible infants had either zone I or posterior zone II with stage 3+ROP disease (76). Patients were randomly assigned to receive bilateral intravitreal bevacizumab (0.625 mg) or laser photocoagulation (76). The primary outcome was treatment failure, defined as the recurrence of retinal neovascularization needing retreatment before 54 weeks of gestational age (76). The authors observed that the rate of recurrence in zone I disease was significantly higher with laser therapy (42% recurrence rate) than with intravitreal bevacizumab (6% recurrence rate) (76). In contrast, a difference in the recurrence rate noted between zone II posterior disease treated with laser therapy (12% recurrence) and zone II posterior disease treated with intravitreal bevacizumab (5%) did not reach statistical significance (76
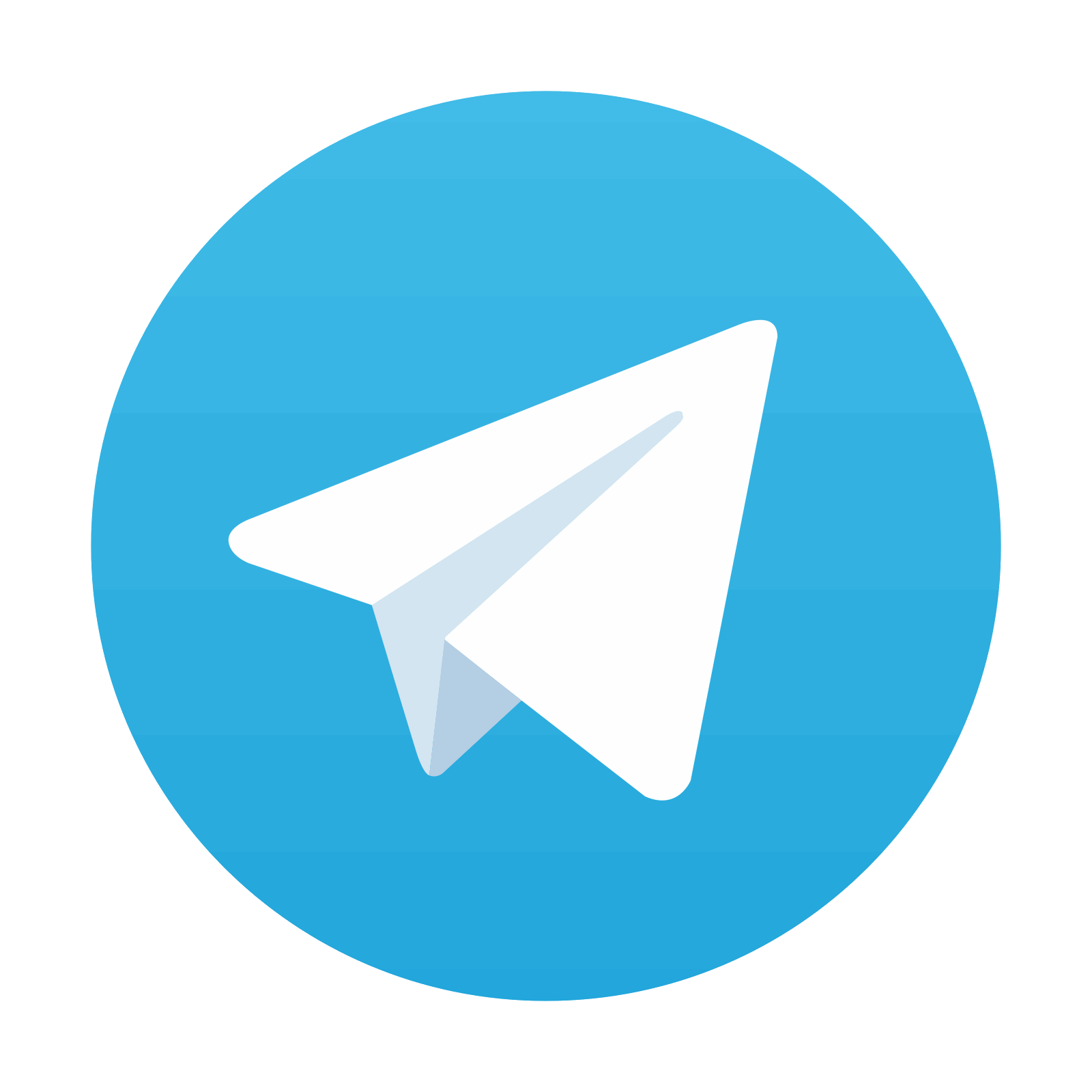
Stay updated, free articles. Join our Telegram channel

Full access? Get Clinical Tree
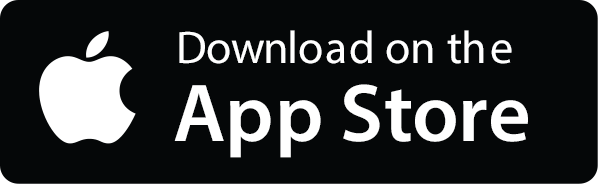
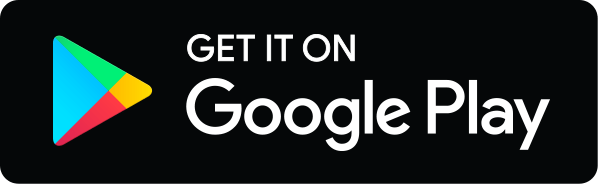