FIGURE 37.1 Morphology. A: Cellular aggregates at isolation following enzymatic dissociation. B: Cells at P1 showing the monolayer population comprised of rounded cobblestone (red arrow), polarized (black arrow), and very small phase-light cells overlying the monolayer (white arrow). C: Cells at P3 showing a dividing cell (arrow). D: Dead cells at P6 with vacuolization and membranous blebs.
The limited ability of these cells to propagate in vitro has remained a challenge. Despite reaching an early senescence, scientists have been able to generate close to 10 billion hRPCs from a single 18-week-old neuroretina (41).
It has been shown that retinal tissues from embryos between the 14th and 18th week of gestation yield the highest number of cells at isolation with a large proportion of cells expressing immature neuronal markers and also survive the longest in the absence of serum. Serum-free conditions are highly desirable for the clinical application of RPCs in human patients. These findings suggest that 14- to 18-week GAs may be the ideal time period during which hRPCs can be isolated without significantly risking the purity of the cell line, as indicated by the molecular characterization of these cells explained below.
Molecular Characteristics
Fetal retina demonstrates a temporally determined, sequential development whereby differentiation of RPCs initiates with their maturation into amacrine, ganglion, horizontal, and cone cells, followed by that into rod, bipolar, and Müller cells (46,47). Retinogenesis typically begins in the retina at 12th week of gestation and is characterized by a progressive appearance of fully matured and differentiated cells in this stereotype sequence.
hRPCs express a wide array of immature neural markers (nestin, Sox2, ki-67, nucleostemin, EGF-R, GD2 ganglioside, Lhx2, Math5, Gnl3, vimentin, Pax6, Chx10 (Vsx2), Otx2, and DCX) and genes controlling retinogenesis (Hes1, Hes5, Dach1, Pax6, Six3, and Six6) all through in vitro expansion (24,42) (Fig. 37.2). Nestin is a protein expressed by intermediate filaments of undifferentiated neural cells, while ki-67 is a nuclear protein found in actively dividing cells. Ki-67, Gnl3, Sox2, and Chx10 have all been implicated in cell proliferation and self-renewal capacity of stem cells. Chx10 is one of the earliest markers of retinal development necessary for cell replication and differentiation of cells along bipolar cells (48). PAX6 maintains the multipotent state of RPCs, while Math5 is a transcription factor necessary for the differentiation of RPCs into ganglion cells. The expression of Math5 by the hRPCs isolated during the 16- to 18-week GA implies that a small proportion of these cells have entered the ganglion lineage, while the much greater positive labeling for markers such as nestin (~95%) and ki-67 (77%) indicates the “stemness” of the majority of these cells (42). These cells coexpress Sox2, Chx10, and PAX6—a molecular profile exhibited only by neural RPCs (42). When exposed to differentiating conditions, such as serum with or without the addition of fibroblastic growth factor, EGF, all-trans retinoic acid, or triiodothyronine, these cells undergo differentiation along the photoreceptor lineage, as evidenced by an increase in the expression of recoverin (Fig. 37.2), rhodopsin, and cone opsins (41,42,49). These findings indicate that hRPCs isolated from the embryonic neuroretina do indeed have the ability to renew themselves and mature into photoreceptors when exposed to specific conditions.
FIGURE 37.2 Immunocytochemistry—(A,B,C,E,F) indicates the immature nature of these cells through positive labeling for nestin, Ki-67, SOX2, PAX6, and vimentin. D: Cells begin to express recoverin upon exposure to differentiating conditions. (Insets at right of images represent higher power views of corresponding large images on the left).
The molecular profiling of these cells along progressive passages shows the maintenance of the expression of genes such as KI67, NES, CHX10, PAX6, and SIX6 through late passage (P6). These findings confirm the preservation of an immature state of the hRPCs through in vitro culture. Through the expansion of just a few cells, hRPCs can be exponentially multiplied yielding large banks of populations that have a largely identical molecular phenotype. Although a gradual decline in the expression of ki-67 and Sox2 has been observed as hRPCs reach a passage number >5 (42), implying a loss in the self-renewing ability of these cells, cell banking is still not impossible because of the highly replicative behavior displayed by these cells in the initial phases of in vitro expansion. Ongoing work has extended to proliferative capacity of hRPCs, which can now be passaged up to 20 times with no apparent change in gene expression or molecular phenotype.
Functionality of Human RPCs
A recent study done on the calcium dynamics exhibited by hRPCs has shown that these cells respond positively to neurotransmitters such as glutamate and n-methyl d-aspartate (NMDA) (42). The presence of receptors for these molecules on the surface of these cells is an important finding because these receptors are known to be expressed by immature retinal neurons.
Transplantation of Human RPCs
Transplantation studies performed in rodent and porcine models of retinal degeneration using rodent and pig RPCs have shown robust integration and differentiation of donor cells into mature photoreceptors. Some studies have even shown an appreciable improvement in visual function of the recipient animals. Pharmacologic manipulation with molecules, such as cyclic adenosine mono-phosphate, has also improved host–graft interaction and synapse formation (50). Attempts at replicating this data using hRPCs in rd1 and rhodopsin -/- mice have shown encouraging results. Preliminary studies have shown that like rodent and porcine RPCs, hRPCs migrate into the degenerating outer and inner nuclear layers of the recipient retina following subretinal transplantation. Once these cells have migrated, they start extending neuronal-like processes into the host tissue and start expressing photoreceptor markers such as rhodopsin (Fig. 37.3). Although the initial results indicate that these cells have the ability to integrate and differentiate, synapse formation and long-term survival of these donor cells still need to be evaluated. Also, while these studies have highlighted the fact that hRPCs, like their rodent and porcine counterparts, do indeed have the potential to integrate and differentiate following transplantation, functional repair remains to be demonstrated.
FIGURE 37.3 Transplantation—(A and B) Histopathologic changes of B6 mouse retina following photocoagulation (arrows) stained with H&E (A), protein kinase C (green in B), and rhodopsin (red in B) staining B6 mouse bipolar cells and photoreceptor cells, respectively. C: Histopathologic section of the (rhodopsin -/- mouse) recipient eye 30 minutes after transplantation showing green fluorescent protein (GFP) positive hRPCs (green) within the SRS, counterstained with nuclear dye DAPI (blue). D: hRPCs (orange, stained with human-specific antimitochondrial antibody—MTC02) migrating toward the host ONL (counterstained blue with DAPI) of the B6 laser injury mouse 7 days after transplantation. E: Low magnification view of hRPCs (green) integrating within the photocoagulated retina of B6 mouse 2 weeks posttransplantation. Cells can be seen extending long processes into the ONL, while some co-label with rhodopsin. F: hRPCs (green cells with red arrow) integrating within the host ONL of rhodopsin -/- mouse (counterstained blue with To-Pro3) and expressing rhodopsin (white arrow) 2 weeks posttransplantation. GCL, ganglion cell layer; INL, inner nuclear layer; ONL, outer nuclear layer; RPE, retinal pigment epithelium; SRS, subretinal space. (Reprinted from Aftab U, Jiang C, Tucker BA, et al. Growth kinetics and transplantation of human retinal progenitor cells. Exp Eye Res 2009;89: 301–310, with permission.)
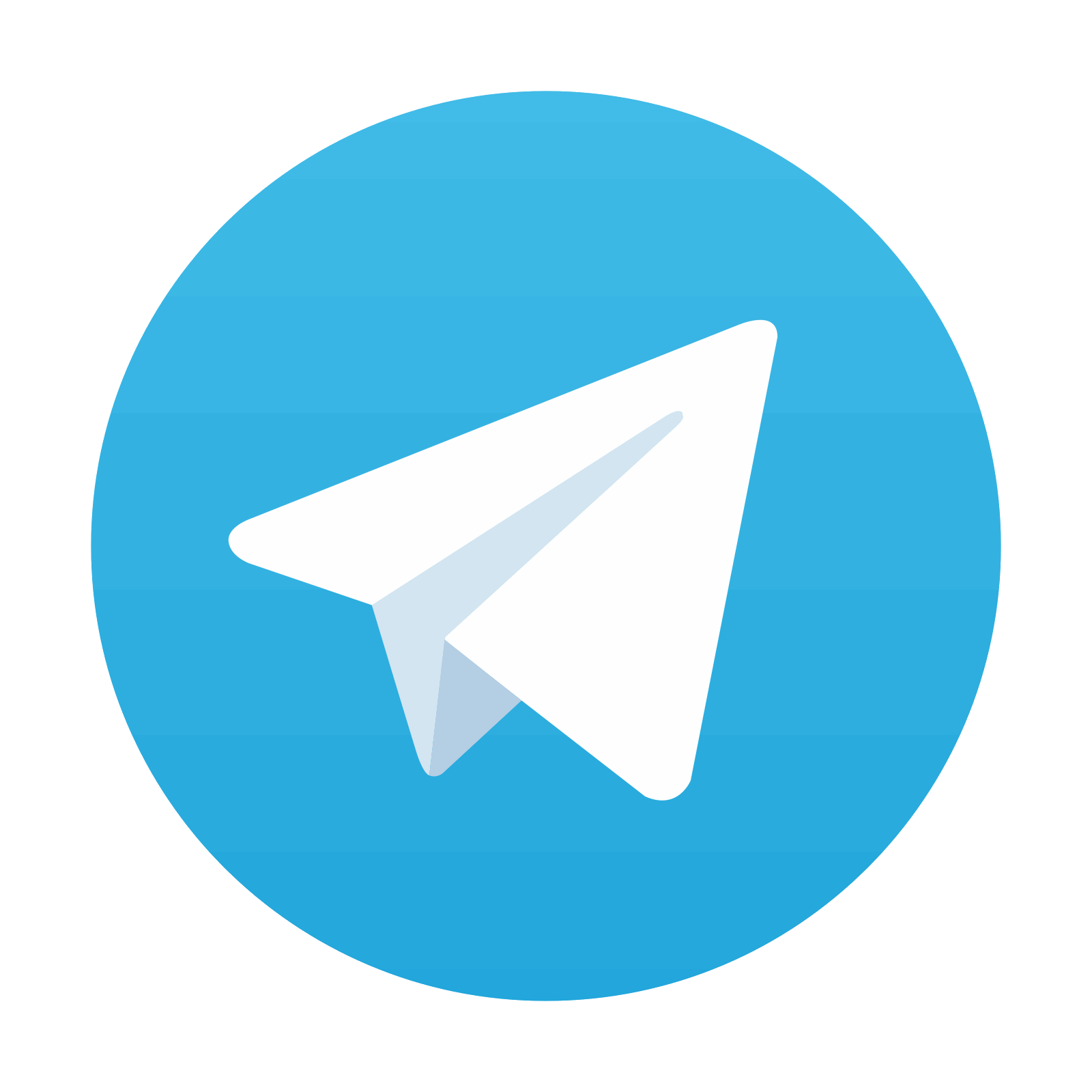
Stay updated, free articles. Join our Telegram channel

Full access? Get Clinical Tree
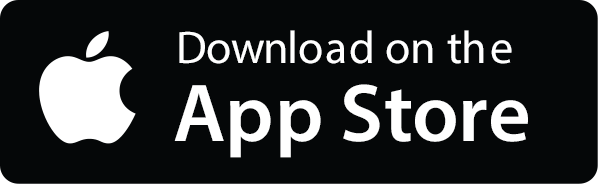
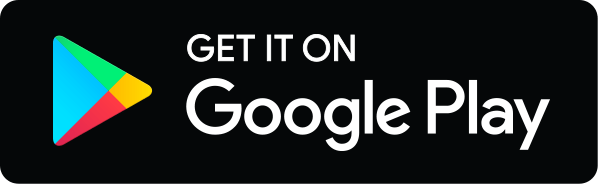