FIGURE 36.1 Fundus photographs of all six chronic implants. A: White arrow showing Artificial Silicon Retina (Optobionics). (Courtesy of Dr. John Pollack.) B: Argus I (Second Sight Medical Products, Inc.). (Courtesy of Second Sight Medical Products, Inc.) C: Active subretinal device (Retina Implant, GmbH). (Courtesy of Prof Eberhart Zrenner.) D: Epi-Ret 25-electrode device. (Courtesy of Prof. Peter Walter.) E: Forty-nine–electrode epiretinal device (Intelligent Medical Implants). (Courtesy of Prof. Gisbert Richard.) F: Argus II (Second Sight Medical Products, Inc.). (Courtesy of Second Sight Medical Products, Inc.) (Reprinted from Weiland JD, Cho AK, Humayun MS. Retinal prostheses: current clinical results and future needs. Ophthalmology 2011;118(11):2227–2237, with permission.)
Epiretinal
Epiretinal prostheses are placed upon the inner retina and stimulate all remaining retinal layers (10,11). While it was initially believed that these devices would stimulate retinal ganglion cells only, numerous studies have reported that the entire remaining retinal-neuronal network is stimulated. Elegant retinal histologic studies by Marc et al. have demonstrated that the photoreceptor loss that occurs in retinal degenerations results in sprouting of bipolar cell dendrites toward the inner retina (12). Retinal stimulation studies with electrical and neurotransmitter-based prostheses have shown an early inner-retinal ganglion cell response, followed by a later synaptically delayed network response. This second network response is often stronger than that of direct retinal ganglion cell stimulation (10,13).
In the earliest human clinical study, the retina was stimulated, epiretinally, by holding a bipolar stimulating electrode approximately 0.5 mm over the retinal surface after pars plana vitrectomy in blind RP and AMD patients (14). This focal electrical retinal stimulation elicited focal light responses (electrophosphenes) that increased in size and brightness with the amplitude and pulse width of stimulation. A second study utilized multielectrode arrays (3 × 3 or 5 × 5) similarly handheld on the retinal surface of blind RP patients and successfully elicited formed electrophosphene percepts in different patterns (15). Subsequent studies with epiretinal prostheses had more variable results (16–18). When the nerve fiber layer was stimulated, electrophosphene percepts were not structured according to the spatial arrangement of the stimulation electrodes.
To date, four different epiretinal prostheses have undergone chronic human clinical trials. These are the Argus I and II (Second Sight Medical Products), Epi-Ret (Epi-Ret GmbH, GieBen, Germany), and Intelligent Medical Implants (IMI Intelligent Medical Implants GmbH, Bonn, Germany).
The Argus I was a 16-electrode epiretinal device surgically implanted in six subjects at a single center (5). Patients were able to identify the differential size of objects and detect object motion. Adverse events from this study included conjunctival erosion and the dislocation of the electrode array in one patient that required repeat tack fixation. The Argus II device improved on the Argus I design by incorporating a 60-electrode thin-film flexible array with all implanted electronics placed entirely within the orbit. An external camera, worn on eyeglasses, delivered image information wirelessly to the implant through a belt-worn visual processing unit. This device acquired video information, processed it and transformed it into pulses of electrical stimulation and delivered these to the retina via the tack-fixated epiretinal electrode array. The few adverse events observed in this study included conjunctival erosion over the extraocular device, endophthalmitis, hypotony, retinal tear/detachment, and one device failure (5). All subjects were able to perceive light with electrical stimulation, and the device also improved object localization, ambulation, and letter reading. The best result in a single patient was a visual acuity measured at 20/200 with an 8× electronic magnification (7).
The IMI epiretinal implant is designed for surgical implantation entirely within the orbit. Studies using this implant have demonstrated ability to elicit visual perceptions, including the appearance of distinct electrophosphenes, simple shapes, and point-to-point relative location (19).
Epi-Ret epiretinal prosthesis is inserted entirely inside the eye, with wireless external power. Experiments using this device involved surgical implantation with retinal tacks for 28 days. The device remained stable intraocularly for this period and produced similar perceptual thresholds as previous epiretinal implants. In this time-limited study, these devices were all successfully explanted (20,21).
Subretinal
Subretinal prosthesis designs place electrodes into the subretinal space. Passive subretinal prostheses have a more simple design in comparison to epiretinal devices or other subretinal devices that employ separate camera technology. Light falls upon these implants in a topographic distribution that then provides patterned electrical retinal stimulation. One limitation of passive subretinal devices is that ambient illumination may not produce sufficient electrical charge for retinal stimulation, which would limit utility of this simple design. In order to circumvent this limitation, external projection glasses have been used to boost illumination to the implant using images from cameras mounted upon the glasses. Subretinal prosthesis designs often allow greater numbers or stimulation electrodes as most designs are based upon photoelectric pixels that both collect light and generate charge (22).
There are currently two subretinal prosthesis models that have been studied in human clinical trials. These are the Artificial Silicon Retina (Optobionics, Glen Ellyn, IL) and the Retina Implant Subretinal Device (Reutlingen, Germany), GmbH.
The first human study of the Artificial Silicon Retina involved surgical placement of artificial silicon retina microchips into the subretinal space of 10 patients with RP (23,24). This chip was 25 µm thick and 2 mm in diameter, consisting of 3,500 microphotodiodes that convert absorbed light into electrical impulses to activate overlying cells. These devices were apparently well tolerated within the subretinal space. While the device did not function in the manner intended, patients reported improvements in their visual function, but noted that areas of improved visual function did not correlate with the location of the subretinal implant. The investigators hypothesized that the implant improved retinal cell function via the neurotrophic or neuroprotective effects of chronic electrical stimulation (25).
A second multicenter study was conducted in 20 patients using the same device. Adverse events included device migration, device fracture during surgical implantation, hemorrhage associated with phacoemulsification, cataract extraction, and visually significant cataract development. Animal studies are currently underway to test the hypothesis that these devices provide therapeutic effects in the retina.
The Retina Implant Subretinal prosthesis consists of an externally powered subretinal array of 1,500 micro-photodiodes with a percutaneous connection behind the ear that provides power via the cable that enters the subretinal space. In this design, the microphotodiode array provides imaging functionality, switching the external power to light-activated regions. This mitigates the need for external light-amplification goggles and allows patients to incorporate eye movements into their object scan and search strategies (26). This device was surgically implanted into the subretinal space of 12 subjects through a 5-mm transchoroidal incision with adjunctive silicone oil tamponade to prevent retinal detachment (26
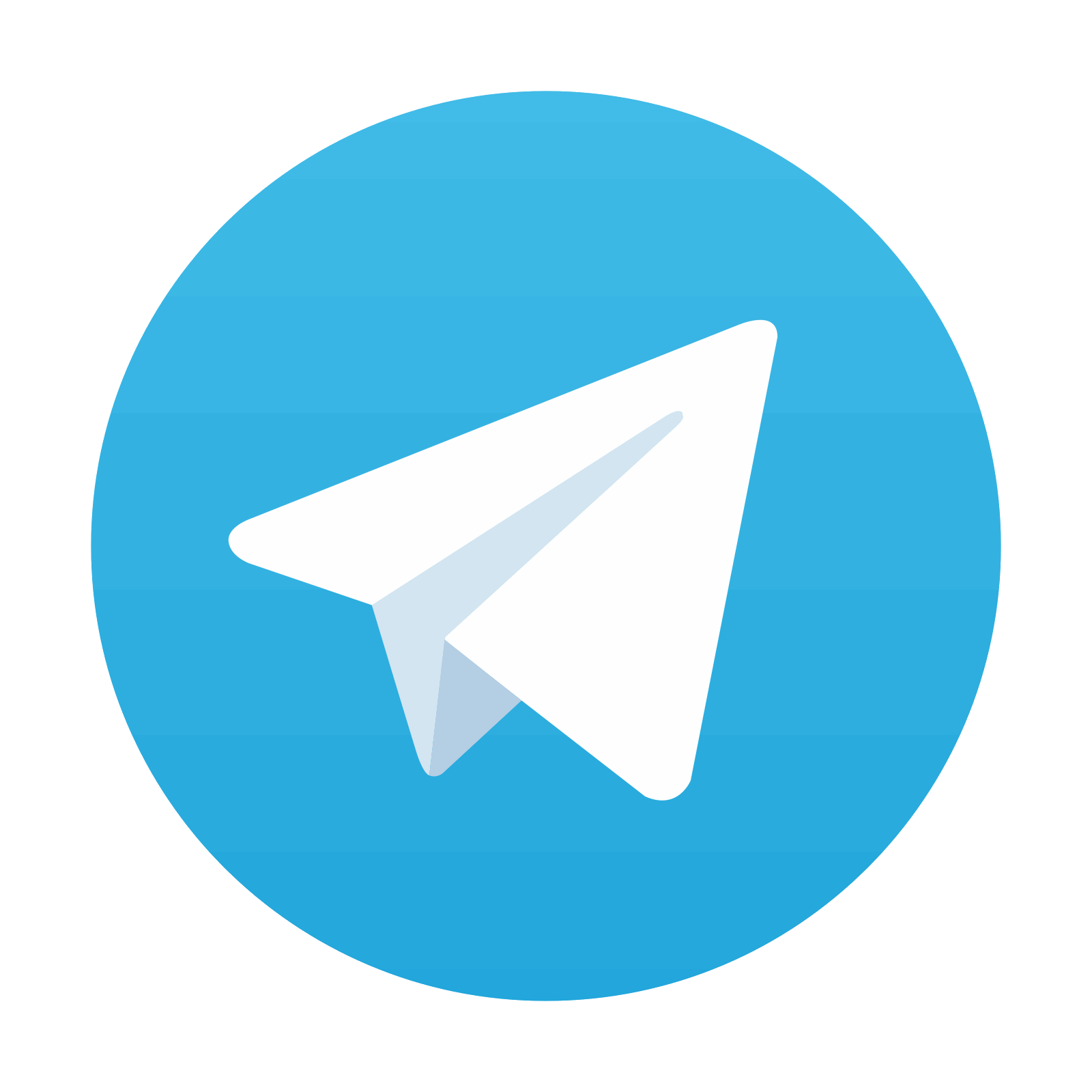
Stay updated, free articles. Join our Telegram channel

Full access? Get Clinical Tree
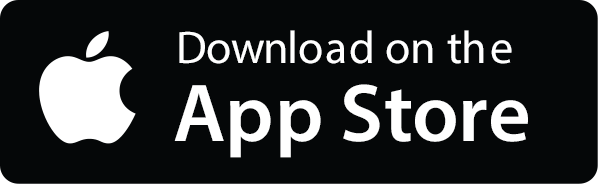
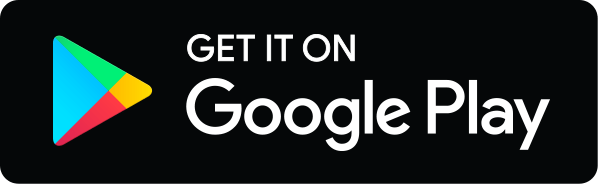