3 Ultrahigh-Resolution Optical Coherence Tomography for Imaging of Ocular Surface Tumors
Optical coherence tomography (OCT) is a powerful imaging modality that has revolutionized the field of ophthalmology by providing real-time, in vivo, cross-sectional images of ocular tissues. The eye is an optically accessible organ, and OCT technique permits high-resolution (HR) images of normal tissues and pathology. Since its development in 1991, OCT has been used for several clinical applications, 1 , 2 and even though it was initially conceived as a means of imaging the eye posterior segment, it was later used for the anterior segment (AS).
Early OCT systems detected light echoes using what is known as time-domain (TD) detection. This technology provided resolution of about 15 to 18 µm. 3 These early devices provided some information regarding the AS anatomy but not the fine details of ocular surface lesions. It was not until the development of spectral-domain OCT (SD OCT) devices, capable of HR OCT imaging—down to 5 µm—and ultrahigh-resolution (UHR OCT) devices—less than 5 µm—that the ocular surface was able to be imaged to a degree that structural details of the corneal epithelium, corneal stroma, and conjunctiva were elucidated. 4 , 5 , 6 , 7 , 8 These newer devices with improved axial resolution and imaging speed made possible the visualization of not only AS dystrophies and degenerations 9 but also of other several ocular surface conditions, disclosing reliable images with high correlation to pathology specimens. 10
Ultrahigh-resolution OCT has provided a noninvasive method to evaluate ocular surface lesions. Ocular surface squamous neoplasia (OSSN), conjunctival melanoma (CMM), and conjunctival lymphoma are conditions that require biopsy for a definitive diagnosis. However, UHR OCT can provide adjuvant information to a clinical diagnosis before excision, thus allowing early differentiation and management of suspicious lesions. 10 , 11 , 12
As OCT technology improves, it has the potential to revolutionize the management of the ocular surface. The purpose of this chapter is to illustrate the evolution and utilization of AS HR and UHR OCT imaging and its clinical applications, specifically in the diagnosis, differentiation, and management of ocular surface tumors.
3.1 Optical Coherence Tomography: The Evolution to Ultrahigh-Resolution OCT
The first demonstration of OCT imaging was reported by Huang et al, 1 showing a TD OCT ex vivo scan of the human retina and its corresponding histology. The image had an axial resolution of around 15 μm, which was almost 10 times finer than conventional clinical imaging modalities such as ultrasound. The most important advance in OCT technology was the transition from TD OCT to SD OCT because the latter is much more efficient than the serial scanning performed by the TD OCT, discussed in detail later in this chapter.
Imaging with OCT technology is based on the use of an optical device known as the Michelson interferometer. The technology compares or correlates one optical beam of light or light wave (reference) with another as the light waves return from the variably reflective tissue layers of the eye. 4 The device creates an interference pattern by gathering reflected light beams from the target tissue and from the reference. Several interference patterns are created in this fashion. 5
In early generation TD OCT machines, multiple readings are produced as a reference mirror physically moves in a linear fashion over the target, creating individual A-scans that indicate a specific measure of the depth and reflectivity of the underlying tissue. These A-scans are then combined to form a composite, cross-sectional image (B-scan). 5 Conventional clinical imaging technologies such as ultrasound, magnetic resonance, or computed tomography have poorer resolution compared with that achieved with this novel technology.
In 1994, AS OCT was reported for the first time by Izatt et al. 3 Later, several TD OCT machines were designed and made commercially available for imaging of the AS. Likewise posterior-segment OCT machines, such as the Stratus OCT (Carl Zeiss Meditec, Dublin, CA), were also used for this purpose. 4 Devices using TD detection technology were capable of achieving satisfactory resolution to image the cornea, anterior chamber, and angle. 13 , 14 Nonetheless, this technology was limited compared with current-generation devices: first, A-scans were limited in number—from 200 to little more than 2000/s—because the reference mirror was required to physically travel as it processed serial A-scans. 15 , 16 Consequently, image resolution was compromised by relatively fewer A-scans and longer times to capture them, making this early technology state susceptible to patient compliance and vulnerable to motion artifacts. 4 The Zeiss OCT1 (Carl Zeiss Meditec, Dublin, CA) was one of the first available devices; it provided an axial resolution of 15 µm with only 100 A-scans per second. 17 Improvements continued with the Zeiss OCT2 (Carl Zeiss Meditec). 18 Later Stratus OCT (Carl Zeiss Meditec) machines were capable of an axial resolution of 10 µm and 512 A-scans in 1.3 seconds. 19
The search for quality images of the AS using TD technology continued, and two AS OCT devices were introduced to the market in 2001: the Slit-Lamp OCT (Heidelberg Engineering, Heidelberg, Germany) and the Visante OCT (Carl Zeiss Meditec). Both were capable of up to 2048 A-scans per second, 20 which granted a significant improvement in terms of image quality, with greater penetrance to a depth of approximately 6 to 7 mm while reducing signal scattering. 19 Additionally, both machines improved scan width (compared with earlier devices) to 15 to 16 mm, which could easily cover all AS structures. These devices still had limited resolution of 25 µm for the slit-lamp OCT and 18 µm for the Visante OCT, respectively. 19 , 20 , 21
The evolution from TD OCT to SD OCT technology, which uses a broadband light source, ultimately brought remarkable improvements to image resolution. 4 Replacement of the reference mirror (TD OCT) with systems that depend on a spectrometer and a high-speed camera to capture and decode (at the same time) the multiple spectra across the whole scan is the key to this dramatic progress. 19 This information is then translated into depth images using Joseph Fourier’s equations. Therefore, this technology is also known as Fourier-domain OCT. 20
The SD OCT imaging acquisition technique was then free from the mechanical limitations of a moving reference mirror and therefore capable of collecting as many as 50,000 or more A-scans per second. 20 Signal-to-noise ratio was considerably increased, and much more detailed images were reached. This innovation was then in most commercially available devices in combination with movement-tracking software products to reduce motion artifacts. 20 OCT imaging of the AS still required use of adapted lenses or the development of new software products. Remember that the initial aim of this novel technology was to image the posterior segment. A few examples of devices that were used for the imaging of the AS are the following: the Spectralis (Heidelberg Engineering), the Cirrus device (Carl Zeiss Meditec), a handheld Bioptigen model (Bioptigen Inc., Research Triangle Park, NC), and three-dimensional OCT (Topcon Medical Systems, Oakland, NJ). 22 , 23 , 24 , 25 These devices were able to obtain axial resolutions from 4 to 7µm, 20 , 22 making it possible to distinguish epithelium from stroma. 23
All things considered, SD OCTs were able to generate detailed, HR images of the AS; nevertheless, they presented marked limitations in width and scan depth. Their horizontal scan width was limited to 3 to 6 mm, making scanning of wider structures extremely difficult. 4 , 23 A new technology that was slightly different from the SD-OCT, called swept-source OCT, was developed (found in devices such as the SS-1000 CASIA [Tomey, Agoya, Japan]). It utilizes a light source with a 1310-nm wavelength and obtains images with a 16-mm horizontal scan width; nonetheless, its axial resolution was only 10 µm. 23
It was a matter of time for the development of the UHR OCT technology; images with an axial resolution of 1 to 4 µm were then possible. 4 Drexler et al, in 2001, reported UHR OCT imaging for the first time. 26 They used a TD OCT machine with resolution of up to 2 to 3 µm to image and demonstrate the Bowman layer. Later, in 2004, 27 they described in vitro corneal OCT with the ability to obtain images with an axial resolution of 1 µm.
The UHR OCT images were accomplished by using a light source with a broad bandwidth of more than 100 nm, as well as a specifically designed spectrometer that detected the fringes collected from both reference and sample arms. 4 Clinically, this allowed for the detailed imaging of the tear film, 28 , 29 tear meniscus, 29 contact lens 28 , 29 , 30 , 31 , 32 , 33 interfaces, and individual corneal layers. 31 , 33 , 34 , 35 , 36 Most of the available literature is based on the use of custom-built UHR OCT devices; a few machines are commercially available. 28 , 29 , 30 , 31 , 32 , 33 , 34 , 35 , 36
At our institution, we have available a custom-built UHR OCT machine that has changed our practice regarding the management of ocular surface lesions (Fig. 3.1). This UHR OCT device is capable of UHR imaging with an axial resolution of approximately 2 to 3 µm, 4 , 10 and it uses a three-module superluminescent diode light source (Broadlighter, T840-HP, Superlumdiodes Ltd., Moscow, Russia) with a center wavelength of 840 nm. 4 , 9 , 10 , 11 , 12 This low-coherence light is passed through a pigtailed isolator and then coupled into a fiber-based Michelson interferometer, generating up to 24,000 A-scans per second. 4 , 9 , 10 , 11 , 12

Based on our previously published experience, certain scan parameters can be recommended: a raster scan with 512 (A-scan) × 2014 (depth) × 128 (frame) pixels with a field of view 8 × 8 mm. 4 These dimensions will allow coverage of the lesion in most cases, although the scan dimension can be increased if necessary. For the generated two-dimensional images, a scan of 2048 (A-scan) × 2048 (depth) pixels provides high-definition of sufficient size to differentiate normal from abnormal tissue and corroborate clinical suspected diagnoses. 37
The use of HR and UHR OCT has become routine care in our ocular surface clinic. We have been able to capture normal and abnormal structures with extraordinary detail and as a result increased our understanding of numerous AS diseases, which has been especially helpful for ocular surface tumors (Table 3.1).
Conjunctival lesion | UHR OCT findings |
Ocular surface squamous neoplasia | Severely thickened, hyperreflective epithelium Abrupt transition between normal and abnormal epithelium |
Conjunctival melanoma | Normal to mildly thickened epithelium Epithelial cleavage indicates some involvement with atypical melanocytes Well-circumscribed subepithelial lesion |
Primary acquired melanosis | Normal thickness epithelium with strong hyperreflectivity of the basal layer No involvement of subepithelial layers |
Nevus | Normal thickness epithelium Well-circumscribed, highly hyperreflective subepithelial lesion within the substantia propria for subepithelial nevi Epithelial and substantia propria lesions seen in compound nevus Cysts within subepithelial tissue |
Conjunctival lymphoma | Normal-thickness epithelium Homogeneous hyporeflective subepithelial lesion |
3.2 Ocular Surface Tumors
3.2.1 Ocular Surface Squamous Neoplasia
Ocular surface squamous neoplasia is a term that encompasses a broad spectrum of neoplastic squamous epithelial abnormalities, including squamous dysplasia, squamous cell carcinoma in situ (also known as conjunctival intraepithelial neoplasia), and invasive squamous cell carcinoma of the cornea or conjunctiva. 38 Clinically, OSSN lesions are elevated, gelatinous, papilliform or leukoplakic and have certain characteristic features that include blood vessel abnormalities and an ameboid and frosted growth pattern when involving the cornea. 39 Whereas most OSSN lesions are clinically obvious to diagnose, some cases are not as straightforward, especially in patients with concomitant complex ocular surface conditions. 37
Excisional biopsy and histopathologic examination have been the gold standard for diagnosis. 38 As we might expect, the role of several noninvasive diagnostic imaging techniques have been studied to help detect OSSN. Confocal microscopy, 40 HR anterior segment ultrasound biomicroscopy (UBM), 41 and OCT, 10 , 11 , 12 have all shown to be beneficial as adjuvant diagnostic techniques; each, however, has its own advantages and disadvantages. For example, UBM and some confocal microscopy devices require eye contact, which increases the level of expertise needed for imaging and also the length of the scan time. Although UBM shows greater depth of penetrance, its resolution is lower than that of other technologies (50 µm). 41 Confocal microscopy can provide optical images at the level of an individual cell, but it can image only a limited area. 10 , 40 , 42 Whereas OCT has the advantage of imaging a larger area in a noncontact fashion, not all images can be fully characterized as thick, and leukoplakic lesions often display image shadowing. 43
The first OSSN series using UHR OCT was published by Abou Shousha et al in 2011; images of OSSN lesions of seven patients were obtained by UHR OCT and compared with their subsequent histologic appearance. 10 Substantial correlation was detected, and the authors found that a thickened hyperreflective epithelial layer and abrupt transition from normal to abnormal epithelium on UHR OCT were considered classic in OSSN. At times, a distinct plane between the lesion and underlying tissue could be noted in thinner tumors. 10 Not all features, however, were detected to the same extent in all scans (several images per lesion were taken, with 32 frames generated per scan), necessitating looking at multiple scans to evaluate for these characteristics. These abnormal UHR OCT features normalized with successful medical therapy. 10 , 12 These characteristic OSSN findings have been repeatedly described in our subsequent publications. 12 , 37 (Fig. 3.2)

Shortly thereafter, Vajzovic et al, in 2011, described a case series of UHR OCT images in various AS dystrophies and degenerations. 9 Images revealed various deposits within particular layers of the cornea, creating a specific pattern for such diseases as Salzmann nodular degeneration and also demonstrating the previously listed classic features of OSSN. In a subsequent study, UHR OCT was used to evaluate whether OSSN could be differentiated from pterygium. Kieval et al, in 2012, reported UHR OCT findings in a series of 34 patients with AS lesions suspicious for OSSN or pterygum. 11 The authors reported that UHR OCT could reliably distinguish pterygia from OSSN. Pterygia disclosed a thin, dark epithelium layer with the presence of subepithelial hyperreflective tissue, whereas OSSN showed the previously mentioned features of a thickened and hyperreflective epithelium, usually with distinct transition zones. These findings were particularly useful in cases where the clinical differentiation between the two entities was not clear. Both groups correlated with the histopathology of resected specimens. 11 In Fig. 3.3, we illustrate several pterygium cases that have been followed up in our institution and have shown consistency with these findings. Additional publications evidenced that UHR OCT could be used to diagnose OSSN in patients with concomitant ocular surface diseases. 9 For example, in Salzmann nodular degeneration, the UHR OCT of the Salzmann nodules revealed a thin, normal epithelium and a subepithelial lesion, and the area of OSSN revealed a hyperreflective, thickened epithelium with distinct transition zones. 9 Furthermore, Thomas et al described how UHR OCT was effective in detecting focal neoplastic disease in a patient with complex ocular surface disease. 37 Future studies will be necessary to more fully evaluate the role of this technology.

Surgical excision with wide surgical margins has been the traditional therapy for OSSN, often with adjunctive cryotherapy. 44 Topical chemotherapeutics agents such as mitomycin-C, 45 5-fluorouracil, 46 and interferon α-2b 47 have also been found to be effective in the management of OSSN. Consequently, as we move forward into the direction of treating OSSN medically, the importance of this noninvasive adjuvant technique gains more significance. It might help physicians to avoid unnecessary corneal or conjunctival scarring or even limbal stem cell deficiency caused from multiple biopsies or extensive surgical excisions. 48 , 49
As we recently reported, 37 we use UHR OCT for regular monitoring of the ocular surface of patients diagnosed with and treated—surgically or medically—for OSSN. UHR OCT imaging often plays a confirmatory role, affirming the lesion resolution seen on clinical examination. 10 , 37 However, it is important to mention that we have encountered patients who were noted to have disparities between clinical and OCT resolution of the tumor (i.e., patients who had a normal slit-lamp examination but evidenced residual disease by UHR OCT). Further studies are necessary to assess the frequency of these findings. In our experience, a few guidelines exist to help direct the use of UHR OCT in the treatment of OSSN: we follow up with patients who are on medical therapy every 6 to 8 weeks with serial UHR OCT scans; this is frequent enough to monitor improvement and prevent prolonged and unncessary use of medications. After lesion resolution, we follow up with such patients with serial scans every 3 to 4 months. After a year of normal examinations, follow-up visits are scheduled every 6 to 12 months. 37 We examine and supervise closely all of our OSSN patients with UHR OCT. The main goal is to ensure that every lesion has been fully resolved and also to remain vigilant for early recurrences with the assistant of this novel technology.
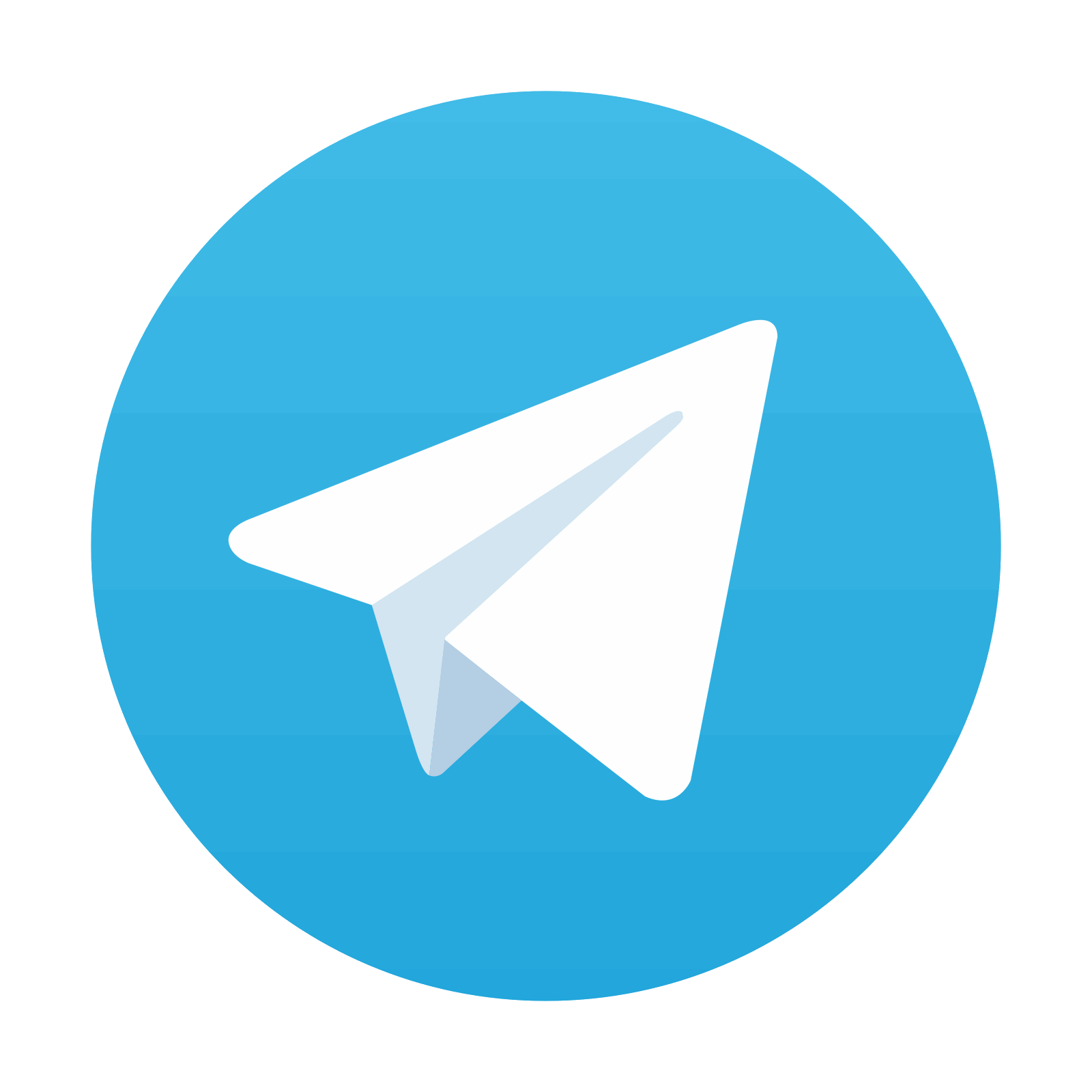
Stay updated, free articles. Join our Telegram channel

Full access? Get Clinical Tree
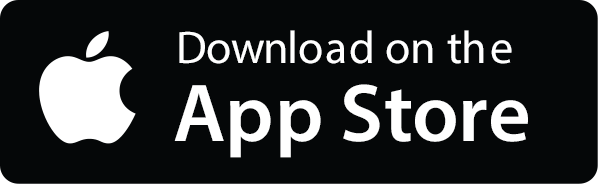
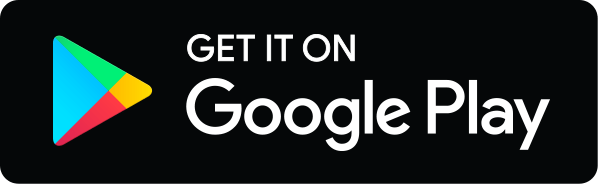
