Summary
Assessment of the optic nerve is a critical component of glaucoma evaluation. Imaging technologies have evolved to provide quantitative measurements of optic nerve head parameters and to detect changes in measurements over time that may suggest glaucoma progression. Optical coherence tomography (OCT) in particular provides reproducible, reliable, and accurate measurements of optic nerve parameters, such as thickness of the retinal nerve fiber layer, and has become integral to clinical practice to guide physicians in glaucoma diagnosis and management. We describe in this chapter a systematic approach to OCT interpretation in clinical practice with attention to its limitations and potential artifacts.
Key words
OCT – optic nerve – retinal nerve fiber layer – glaucoma – optic neuropathy – imaging – eye3 Optical Coherence Tomography of the Optic Nerve
3.1 Introduction
3.1.1 Glaucoma Imaging
Glaucoma is characterized by progressive loss of retinal ganglion cells that causes structural changes to the optic nerve and thinning of the retinal nerve fiber layer (RNFL) with subsequent corresponding visual field defects. Prompt recognition of early structural changes is critical to mitigate permanent vision loss from glaucoma. Visual field defects may not become apparent until 40% of retinal ganglion cells are lost, suggesting that perimetry alone may not capture early disease. Careful stereoscopic examination of the optic nerve remains a hallmark in detection and management of glaucoma, but subjective interpretation and two-dimensional documentation can limit the ability to identify subtle changes. Along the same lines, stereoscopic photographs of the optic nerve and monochromatic photographs of the RNFL are limited by only fair agreement in interpretation between glaucoma specialists and the lack of quantitative information.
Imaging modalities have been developed to aid ophthalmologists in optic disc assessment to monitor structural signs of glaucoma. Technologies such as the Heidelberg Retina Tomograph (HRT, Heidelberg Engineering, Heidelberg, Germany) emerged to provide quantitative information about the topography of the posterior fundus using confocal laser scanning microscopy (Fig. 3‑1). Similarly, the GDx scanning laser polarimeter estimated RNFL thickness by measuring its birefringence using polarized light (Fig. 3‑2). However, the detailed and precise imaging of the optic nerve possible with optical coherence tomography (OCT) is the most common computerized imaging of the optic nerve in current clinical practice. Commercially available OCT devices include Cirrus HD-OCT (Carl Zeiss Meditec, Dublin, CA), Spectralis (Heidelberg Engineering, Heidelberg, Germany), RTVue-100 (Optovue, Freemont, CA), 3D OCT-2000 (Topcon Medical Systems, Oakland, NJ), and RS-3000 Advance (Nikon, Tokyo, Japan). These different commercial devices utilize similar OCT acquisition principles but vary in scanning protocols and segmentation algorithms (discussed in depth in Chapter 7).




3.1.2 Optical Coherence Tomography
OCT noninvasively acquires a real-time image of the ophthalmic structures in optical cross section. Compared to previous models of time-domain OCT technology, spectral domain OCT (SD-OCT) produces higher resolution images by utilizing spectrally separated detectors and taking Fourier transform of broad spectral information (Fig. 3‑3). Using a near-infrared super-luminescent diode light, SD-OCT acquires 26,000 to 85,000 axial scans per second for an axial resolution of about 5 μm and transverse optical resolution of about 14 μm. Images are acquired either in raster scans (parallel frames), radial scans across the optic nerve, or as concentric circles to measure peripapillary RNFL thickness in a 3.46-mm scan circle centered on the optic nerve head (ONH). Software can now delineate Bruch’s membrane opening (BMO) as standard reference for defining the region of the ONH, while previous device iterations required manual centration of the scan circle. Additionally, eye motion tracking recently has been incorporated to reduce noise and artifacts in acquired images. Software programs also contain a normative database of measurements from healthy subjects to aid interpretation of patient’s measurements by comparing them to normal ranges.


3.2 OCT Output
3.2.1 Overview
Most commercial OCT platforms generate a printout to summarize quantitative and qualitative measurements of RNFL and ONH parameters. Depending on the software algorithm and technical capabilities of the device, these measurements include RNFL thickness, cup-to-disc ratio, neuroretinal rim area, and neuroretinal rim volume.
In addition, quality metrics are given to allow the interpreter to assess the validity of the data output. Patient identification and date of study are embedded in the report. Many printouts also include a quality score or indication of signal strength, as lower quality images are more prone to artifactual measurements. Additionally, algorithmic delineation of the circumpapillary RNFL is demonstrated over acquired B-scan images in order for the user to assess accuracy of the RNFL tracing.
Retinal Nerve Fiber Layer Measurements (Fig. 3‑4 and Fig. 3‑5)
Measurements of circumpapillary RNFL thickness feature prominently in the output and are given as a global average, by quadrant, and by clock-hour for each eye. Summary measurements are presented in table form, including degree of RNFL symmetry, as asymmetry between eyes can be associated with glaucoma. Thickness maps illustrate RNFL measurements around the detected ONH, with warmer colors corresponding to thicker values in a classically butterfly-shaped distribution around the optic nerve. Deviations from normal thickness are also illustrated in a deviation map to highlight potential areas of RNFL loss.


RNFL thickness is also graphed in a “TSNIT” plot, in which the circumferential RNFL thickness measurements are “unrolled” into linear form and presented from the temporal to inferior quadrants (the “Temporal-Superior-Nasal-Inferior-Temporal” plot). In normal eyes, this plot should demonstrate a characteristic double hump, with thickest RNFL measurements peaking at the inferior and superior regions.
Patient measurements are plotted over a normative measurement database, and interpretation of the probability of abnormal values is aided by color coding with red, yellow, green, and white shading. Red color highlights values in the lowest 1% of normative values and is considered outside normal limits. Yellow flags values from the 1st to 5th percentile and suggests borderline abnormality. Green indicates the middle 90% of normal, from 5th to 95th percentiles, and white flags values greater than the 95th percentile of normal subjects. Plots over normative values compare patient measurements with those from subjects of similar age, as age-related changes lead to RNFL thinning over time even in the absence of disease. Despite similar presentation and color coding of the TSNIT plot between devices, images cannot be directly compared from one OCT device to another because different manufacturers generate these plots from their own normative databases.
Optic Nerve Head Parameters
ONH analyses are generated by defining the boundary of the optic disc using the BMO. The area of the neuroretinal rim is measured as the minimum distance from the BMO to the internal limiting membrane (ILM), a parameter described at the BMO-MRW (Bruch’s membrane opening minimum rim width). The Spectralis device acquires radial scans across the ONH to determine BMO-MRW and thus define the neuroretinal rim (Fig. 3‑6). For the Cirrus HD-OCT, ONH data is extracted from the 200 × 200 Optic Disc Cube scan using minimum area rather than distance to define the BMO-MRW, and normative measurement limits are corrected for optic disc tilt and size. Resulting metrics include cup-to-disc ratio, neuroretinal rim area, and neuroretinal rim volume. These ONH measurements complement RNFL data to detect glaucoma or progression, but themselves have less diagnostic accuracy. However, ONH parameters can suggest nonglaucomatous optic neuropathy if there is thin RNFL in the setting of normal neuroretinal rim and cup-to-disc ratio.

3.2.2 OCT Interpretation
As with perimetry, clinicians should interpret OCT output systematically in order to avoid overlooking information that could influence decision-making and to identify artifacts or anatomic variants that affect measurement values. 1 Although there is no standardized system for interpretation, several important components of the output summary should be regularly assessed for accuracy and reliability (Fig. 3‑7 and Table 3‑1).


Confirm Patient’s Name and Age
Ensure that the output file is from the correct patient and confirm that age was put correctly, as measurements are made in reference to age-matched nomograms.
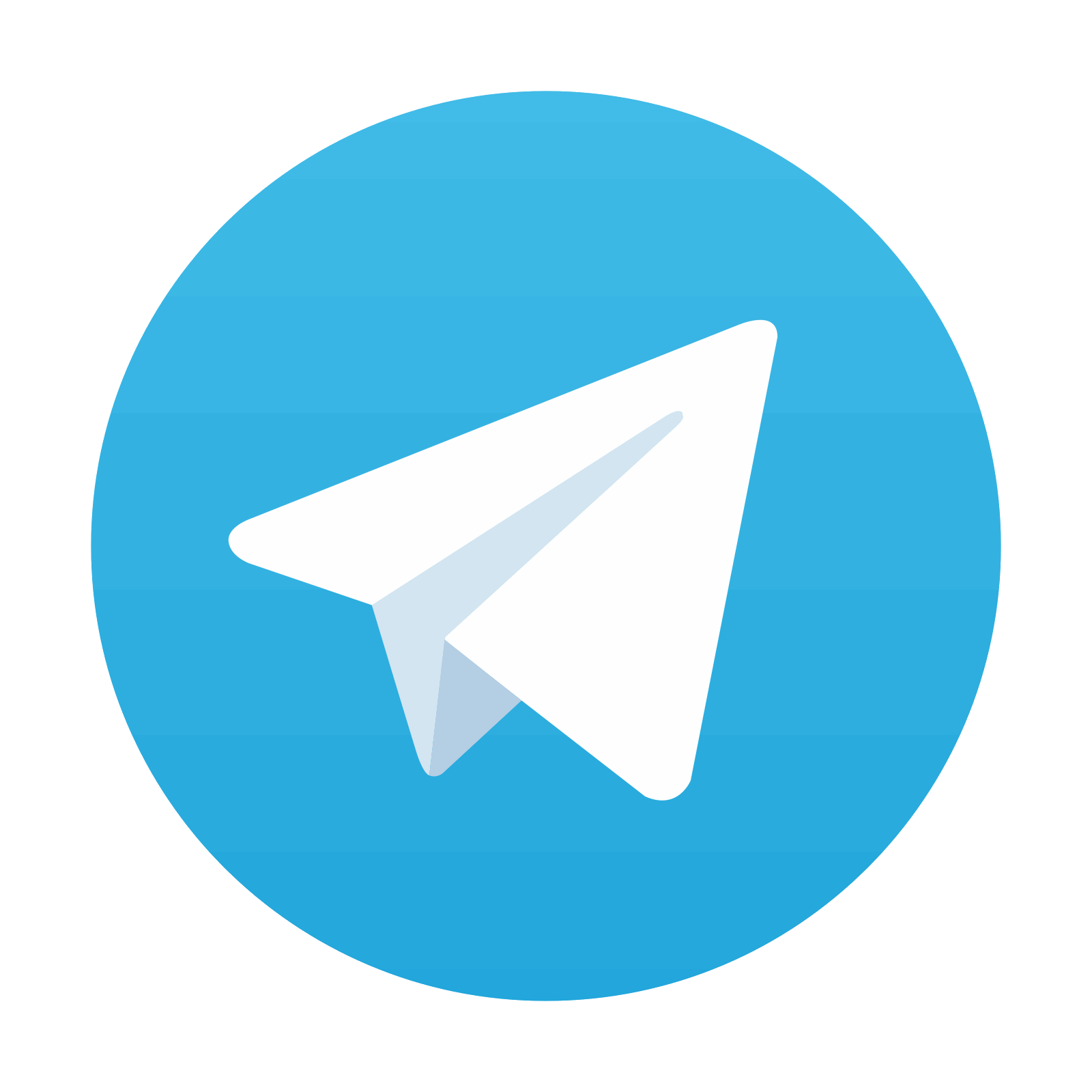
Stay updated, free articles. Join our Telegram channel

Full access? Get Clinical Tree
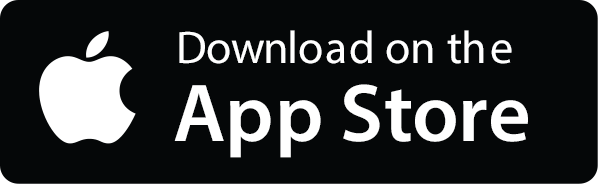
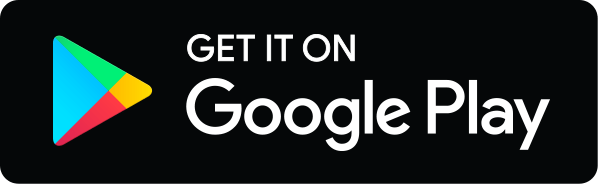
