Segment: Challenges
Prithvi Mruthyunjaya
In proangiogenic diseases such as neovascular age-related macular degeneration (ARMD) and diabetic retinopathy (DR), there is growing interest in delivery of targeted therapeutic agents to the posterior segment. Even if a new therapeutic agent is found to be active in preclinical studies to specific retinal structures or disease states, the challenge remains to bring the agent to the target site in safe and effective concentrations.1 Furthermore, finding delivery methods to minimize administration frequency while maintaining therapeutic drug concentrations at the target tissue may help modulate the disease while minimizing side effects from repeated delivery.
BARRIERS TO DRUG DELIVERY
Drugs must enter the eye through a specified route, maintain its active biochemical properties (bioactivity), achieve a therapeutic concentration at its target site, and sustain an effective concentration to counteract the disease process. Despite identification of the best agents to treat posterior segment diseases, there are numerous physical and physiologic barriers to achieving sustained and therapeutic drug concentrations at the retina and choroid. Barrier functions work to inhibit entry of the agent into the eye, specifically the retina and vitreous, while clearance mechanisms remove drugs that may have been successfully delivered. In addition, the disease state itself may alter both barrier and clearance mechanisms, thereby altering expected drug concentrations and duration of contact with target structures.
The ocular surface is the most anterior barrier to ocular drug penetration. Aqueous tears dilute and flush topically administered medications into the lacrimal drainage system. The tear film volume is approximately 5 to 7 μL, which is replaced almost continuously.2 However, the volume of a typical eye drop (25–50 μL) is typically cleared by bulk flow through the nasolacrimal duct in 5 minutes, leaving insufficient time for effective ocular penetration. Systemic absorption may also occur at the conjunctival and lacrimal sac via local capillaries or through sinus mucosal absorption.3
The cornea provides a structural and biochemical barrier to drug delivery. Apical corneal tight junctions can limit paracellular drug passage. However, inefficient, transcorneal permeation remains a major route of topical drug entry into the anterior chamber. Biochemical mechanisms within the cornea, including efflux pumps, peptide transporters, and multidrug resistance proteins, further limit transport from the ocular surface into the eye. The result is that <3% of the drug volume of a topically administered agent will enter into the anterior segment, which may not allow for therapeutic concentrations to effectively enter the eye.4–6
The blood-ocular barrier is the predominant deterrent to achieving therapeutic posterior segment drug concentrations via systemic, topical, or periocular administration. The anterior blood-aqueous barrier (BAB) is formed by the endothelial cells within the anterior uveal tract (iris and ciliary body), limiting plasma albumin and hydrophilic drugs from entering the aqueous humor.3,7 The BAB may significantly reduce intraocular concentrations of drugs that are designed to enter the eye through anterior pathways (conjunctival or topically).
The blood-retina barrier limits diffusion of drugs and targeted agents delivered through (BRB) a systemic or periocular route. There are two components of the Blood-Retinal Barrier (BRB): the retinal pigment epithelium (RPE) (outer barrier) and the tight junctions of the retinal vascular endothelial cells (inner barrier). The highly perfused choriocapillaris have abundant fenestrations, which provide minimal resistance to the transport of molecules into the choroid. However, the Retinal pigment epithelium (RPE) itself, with the exception of its homeostatic function of allowing selective passage of nutrients and removal of waste products between the choroid, restricts movement of particles.6,8 The retinal vascular endothelium also has a low permeability tolerance and, typically, only highly lipophilic compounds can penetrate this inner blood-ocular barrier.6 As only a fraction of the systemic blood volume flushes through the eye, very small quantities of systemically delivered drugs can be detected within the eye.2,9
When a drug reaches the posterior segment (retina or vitreous), it may be eliminated by two routes: anterior elimination involves drainage through the trabecular meshwork and by bulk flow aqueous turnover, while posterior elimination requires the drug to penetrate through the blood-retinal barrier and then actively or passively transported out of the eye. Elimination through these routes favors small, lipophilic molecules and may be altered by systemic medications.2,3
Ocular disease states may influence posterior segment drug entry and elimination. Posterior segment inflammation may have opposing effects on drug elimination based on the biochemical properties of the agent. For example, in the setting of inflammation, elimination of aminoglycoside can be increased, whereas beta-lactam antibiotics can be decreased due to permeability and active transport mechanisms.2 In retinal vascular diseases such as DR, damage to retinal vascular endothelium may enhance drug permeability and limit clearance, thereby increasing retinal drug concentrations. Similarly, in choroidal neovascular diseases such as neovascular ARMD, disruption of RPE outer barrier function may enhance drug entry and limit elimination.
ROUTES OF DRUG DELIVERY
Numerous routes have been proposed and used to deliver drugs to the posterior segment in attempts to control retinal vascular and neovascular diseases. These routes include (a) systemic delivery, (b) topical administration, (c) periocular administration, (d) transscleral delivery, and (e) intravitreal administration. Each method has its own advantages and disadvantages, which will be discussed.
Systemic Delivery
Systemic drug delivery to the posterior segment involves oral or intravenous drug administration. Patient compliance, systemic absorption, and first-pass drug metabolism determine the bioavailability of the drug as it is transported to the eye through blood vessels. Drug access to the posterior segment requires penetration across the blood-ocular barrier, which may typically occur with lipophilic drugs. Alternatively, drugs may diffuse into the posterior chamber through a concentration gradient from the anterior chamber and aqueous humor. These penetration mechanisms are constantly being thwarted by normal elimination mechanisms, resulting in an inefficient method of attaining therapeutic intraocular drug concentrations. In order to combat these barrier and elimination forces, high drug doses or frequent systemic administration is necessary to produce therapeutic ocular concentrations. The drawback to this method is the increased exposure of the drug to the systemic circulation.
In patients with DR, oral agents have been used to target upregulated protein kinase C (PKC) as a method to reduce vascular permeability and mediate concurrent overexpression vascular endothelial growth factor (VEGF) expression.10,11 PKC412 is an oral nonspecific multikinase inhibitor that blocks VEGF-1 and VEGF-2 receptors, Platelet-derived growth factor receptors (PDGF), and several PKC isoforms. In a double-masked, randomized, variable dose, controlled clinical trial of 141 patients with diabetic macular edema, oral doses of 100 mg/d significantly reduced macular thickening and improved visual acuity.12,13 Liver toxicity was a possible concern, limiting its continued systemic use. Ruboxistaurin mesylate (LY333531) is a specific PKC-beta isoform inhibitor that was tested in a 36-month randomized, placebo-controlled trial of 685 patients with nonproliferative DR. A significant risk reduction (40%) in sustaining moderate vision loss and preventing progression of foveal macular edema was demonstrated.14–17
Choroidal neovascularization in ARMD has been established as a VEGF-driven pathologic response to angiogenic signaling.18 Initial antiangiogenic strategies utilized selective VEGF-165 isoform inhibition by intravitreal injections of pegaptanib sodium (Macugen, OSI/Eyetech, New York, NY).19,20 Data from examination of extracted choroidal neovascular membranes demonstrated multiple isoforms of VEGF, including 121 and 110, exist within these lesions but could not be blocked with pegaptanib sodium alone.21 Bevacizumab (Avastin, Genentech, Inc., South San Francisco, California) is a full-length, humanized monoclonal antibody with two active binding sites that can bind and inhibit all active VEGF-A isoforms. Prior to the Food and Drug Administration (FDA) approval of ranibizumab (Lucentis, Genentech, Inc., South San Francisco, California) for neovascular ARMD, systemic administration of bevacizumab was studied in the Phase 1/2 Systemic Avastin for Neovascular AMD (SANA) Study. In an open-label, uncontrolled study, 18 patients with subfoveal choroidal neovascularization were given intravenous bevacizumab infusions (5 mg/kg) at study entry and an additional one to two injections in the subsequent 4 weeks. No adverse events were noted and there was mild, but controllable, elevation in systemic blood pressure. Visual acuity improved and central retinal thickness decreased through 24 weeks with few re-treatments after the initial series.22,23 These examples demonstrate the feasibility of some systemic agents to reach effective ocular concentrations, but with nonocular side effects.
Topical Delivery
Eye drop formulations of medications designed to treat posterior segment diseases have the distinct advantages of easy, noninvasive delivery (Fig. 28.1). However, as discussed above, the tear film and cornea pose a formidable barrier to absorption through the ocular surface. Once through the corneal layers, the drug may diffuse into the aqueous humor and travel around the lens zonules into the vitreous humor where it may contact the retina. Alternatively, noncorneal entry by a topical drug may occur either directly through the pars plana into the vitreous cavity or directly across the sclera and into the vitreous cavity after traversing the choroid, RPE, and retina.6 Just entering the eye by a topically placed drug compound does not ensure adequate bioavailability to attain therapeutic efficacy at the retinal or choroidal target site. A concentration gradient is required to allow for diffusion into the posterior segment, but drug clearance mechanisms via aqueous outflow and biochemical drug inactivation may quickly reduce anterior chamber concentrations. Repeated installation of high concentration eye drops may help ensure this gradient, but may also increase the risk of local or systemic toxicity.7
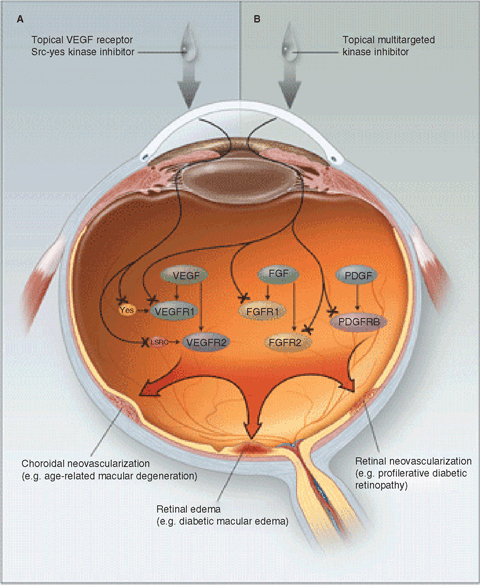
FIGURE 28.1. Topical use of kinase inhibitors. Retinal edema and neovascularization of the choroid and retina are the primary causes of vision loss in most developed countries. Multiple mechanisms mediate these changes, including pathways involving vascular endothelial growth factor (VEGF), platelet-derived growth factor (PDGF), and fibroblast growth factor (FGF) receptor kinases. These growth factors bind a variety of receptors, including VEGFR1, VEGFR2, FGFR1, FGFR2, and PDGFRβ. A recent study by Scheppke et al.24 showed that topical application of a VEGF receptor Src–Yes kinase inhibitor can essentially eliminate retinal edema in animals (Panel A). A similar study by Doukas et al.25 showed that a topical multitargeted kinase inhibitor of VEGF, PDGF, and FGF pathways can reduce choroidal neovascularization and retinal edema in animals (Panel B). Note the corneal penetration and proposed diffusion of the drug into the vitreous cavity. (Reproduced from Aiello. N Engl J Med. 2008;359:967, with permission.)
Despite these challenges, studies are underway to determine the efficacy of topical drops for ocular neovascular diseases focusing on protein kinase inhibition. These drugs may bind a variety of receptors that mediate signaling pathways used for cell proliferation, blocking apoptosis, and vascular remodeling. A unique feature of this drug class is the inhibitory effect on multiple VEGF family members including VEGFR1, VEGFR2, FGFR1, FGFR2, and PDGFR-B.18 In preclinical studies, Scheppke et al. reported the use of a topical VEGFR2–Src kinase inhibitor in knockout mice, which reduced both VEGF-induced vascular permeability and laser-induced vein occlusion-associated macular edema in rabbits.24 Using a different topical multikinase inhibitor that targeted VEGF, PDGF, and FGF, Doukas et al. noted reduced vascular leakage and reduction of choroidal neovascularization in rodent models.25 Another agent, pazopanib (GlaxoSmithKline), has demonstrated choroidal neovascularization (CNV) inhibitory effects in a murine model with activity against VEGFR1, VEGFR2, VEGFR3, PDGFR, c-Kit, and FGF.26 Phase 1 studies with topical delivery of pazopanib are currently underway.27
Periocular Delivery
Periocular delivery utilizes passage of the drug through the outer scleral eye wall to reach choroidal, retinal, and vitreous targets. Drugs have been delivered into the sub-Tenon, retrobulbar, episcleral, intrascleral, or suprachoroidal locations. Many of these locations, especially retrobulbar and sub-Tenon space, can be easily accessed in the clinic setting and have been used for many years to delivery periocular steroids that have been shown to have a therapeutic effect. These delivery techniques all have the advantage of avoiding direct intraocular penetration or wound creation to place the drug or drug delivery device. The relatively large surface area of the sclera and the expandable orbital tissues allows for long-acting drug depots to be placed in these locations, creating opportunities for more sustained release through the sclera. Further, the local administration may limit systemic toxicity as the drug is delivered close to its target site (Fig. 28.2).
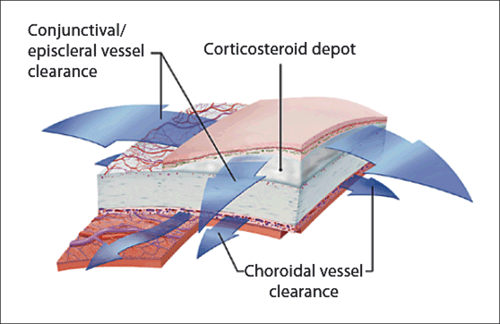
FIGURE 28.2. Subconjunctival drug depots are cleared by both periocular (conjunctival and episcleral vessels and lymphatics) and choroidal vascular channels. (Reproduced from Lee SS, Robinson MR. Ophthal Res. 2009;41:124, with permission.)
Transscleral Delivery
It is estimated that only 1% of drug placed on the episcleral surface will enter the retina and vitreous.28 The ocular tissues (sclera, choroid, and RPE) constitute a physical barrier to drug penetration by pharmacokinetic modeling and from a protein transport data.29,30 However, in vitro and in vivo studies have demonstrated that the sclera is permeable to low- and high-molecular weight compounds as well as other novel drug formulations such as single-strand oligonucleotides, large molecular immunoglobulins, and adenoviral vectors coding for antiangiogenic proteins as large as 50 to 75 kDa.31–37 Scleral permeability appears to decrease with increasing molecular weight and molecular radius, with the latter being more predictive for transscleral passage.29,31
Clearance mechanisms must also be considered in determining the low retinal and vitreous concentrations obtained after periocular drug delivery. Such mechanisms have been implicated, for example, in the lack of clinical superiority in the use of periocular depot of anecortave acetate, an angiostatic steroid formulation, compared to verteporfin photodynamic therapy in neovascular ARMD patients.28,38
The choroid and choriocapillaris represent high-flow vascular beds that may contribute to bulk drug elimination. However, cryotherapy elimination of the choroid in rabbits or in vitro did not enhance vitreous concentration after a periocular steroid injection, suggesting the importance of conjunctival and lymphatic drainage.39–41 Kim et al. utilized magnetic resonance imaging to follow the movement of gadolinium–diethylenetriamine pentaacetic acid (Gd–DTPA) from an episcleral implant placed in rabbit eyes and found Gd–DTPA accumulation in the buccal lymph nodes. In periocular delivery studies, following euthanasia, intravitreal drug concentrations increase, suggesting preferential transscleral permeability in the absence of lymphatic, conjunctival, and choroidal clearance mechanism.29,42,43 Robinson et al. demonstrated the importance of the conjunctival blood vessels and lymphatics in the rabbit eye, by demonstrating detectable triamcinolone acetonide (TA) vitreous levels after excising a conjunctival window at the site of periocular delivery, but not in eyes with intact conjunctiva.29,39
Several approaches have been reported to enhance intraocular drug availability following periocular delivery, which focus on minimizing or eliminating clearance mechanisms (Fig. 28.3). Drug-releasing episcleral exoplants provide higher and more sustained scleral drug concentration gradients, which allow greater transscleral diffusion despite normal conjunctival and lymphatic clearance mechanisms. Device construction with polymeric coatings may help shield the drug core from washout from the periocular tissues and lymphatics. A flexible, refillable silicone device that was sutured to rabbit eyes demonstrated increased retina and vitreous sodium fluorescein compared to a periocular injection. The unique device sheltered the drug from the conjunctiva and only allowed direct contact with the sclera.44 In a similar concept, a nonbioerodible polyvinyl alcohol (PVA)–betamethasone episcleral exoplant allowed preferential delivery into the rabbit retina-choroid rather than the vitreous.45 Using a novel synthetic polymer, poly(methylidene malonate) (PMM2.12), 8 × 1 mm TA discs were coated and sutured to the scleral surface to allow therapeutic intravitreal levels in rabbit eyes for up to 5 to 8 weeks.46
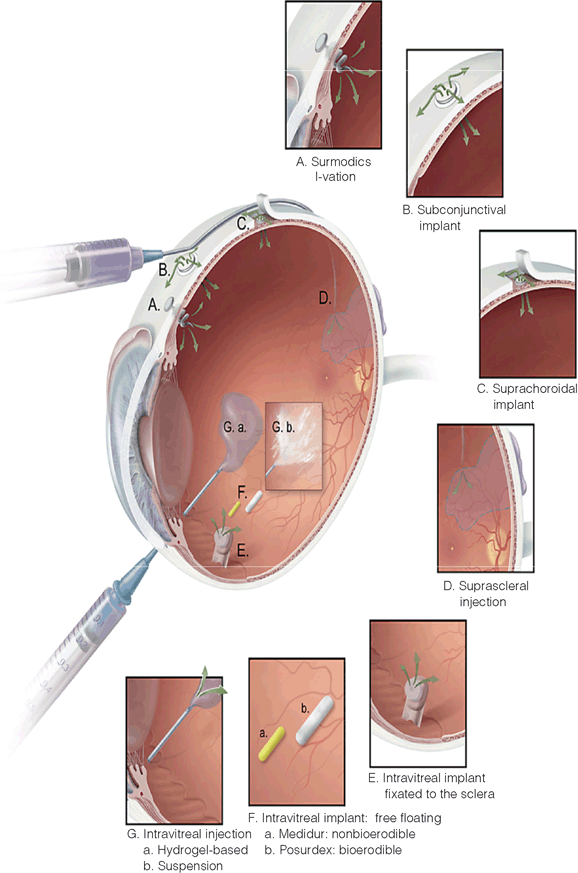
FIGURE 28.3. Various routes and systems of drug delivery for posterior segment disorders. (Reproduced from Lee SS, Robinson MR. Ophthal Res. 2009;41:124, with permission.)
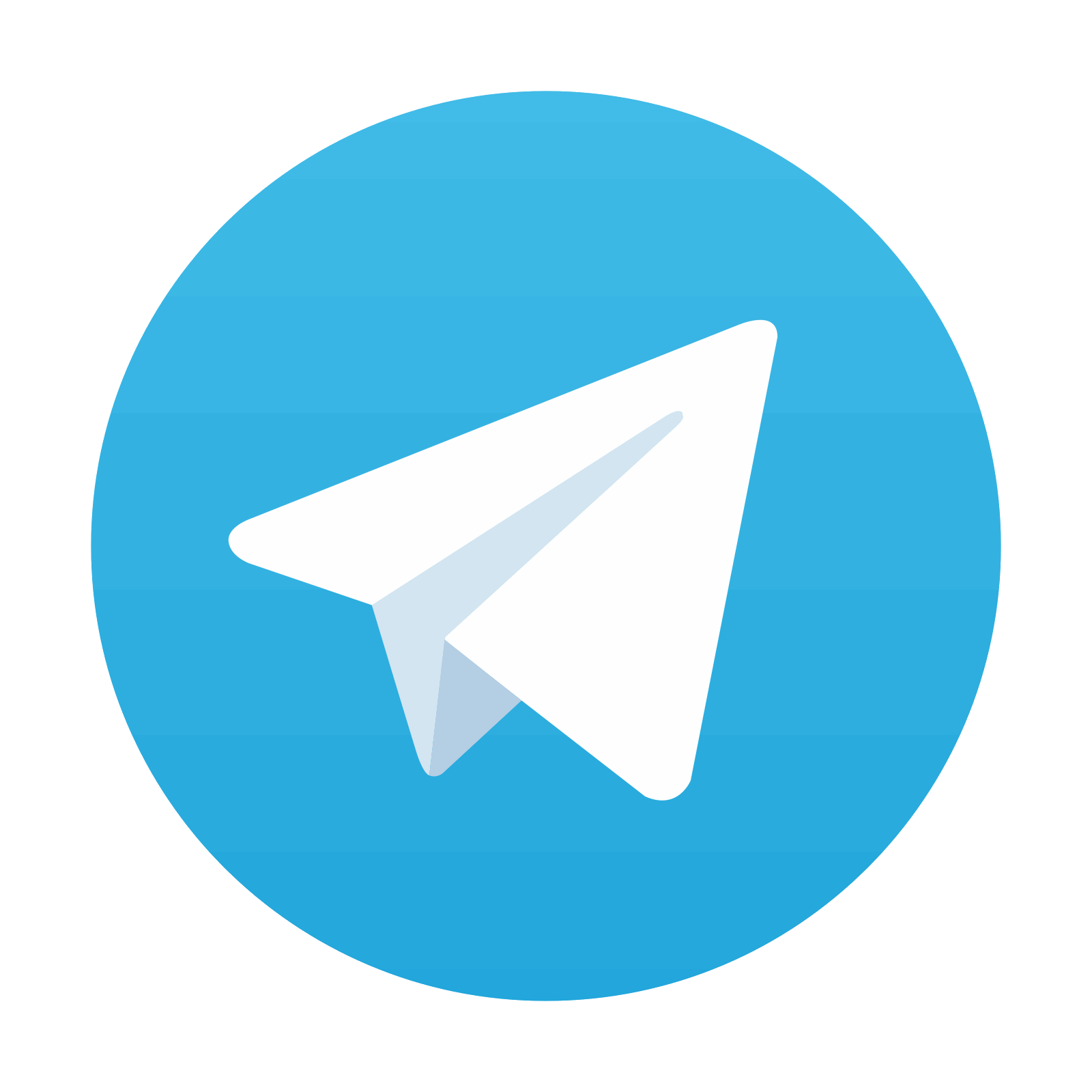
Stay updated, free articles. Join our Telegram channel

Full access? Get Clinical Tree
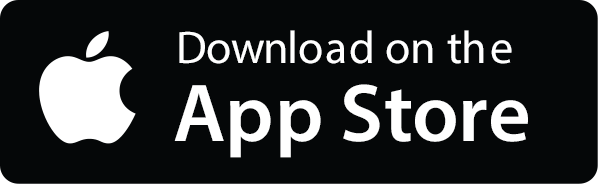
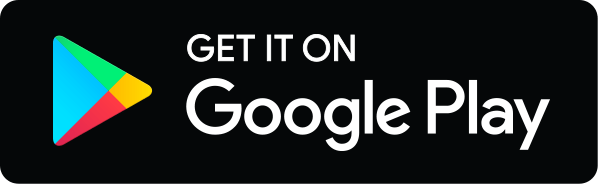