Neovascularization
Robert N. Frank
INTRODUCTION
The human retinal vasculature is essentially complete at birth in the full-term infant. These vessels elongate with growth of the eye through childhood and adolescence, but new vessel sprouts do not appear. Mitogenesis of cells of the capillaries in the adult retina is very infrequent,1 and all postnatal retinal blood vessel sprouting in the human eye, called neovascularization, is abnormal.
NORMAL AND PATHOLOGICAL RETINAL VASCULAR GROWTH
Despite this major temporal difference between normal and pathological retinal blood vessel development, many of the molecular mechanisms of these two processes are, to the best of our present understanding, similar. The initial formation of primitive vessels in the developing retina from vascular precursors is called vasculogenesis, while sprouting of new vascular endothelial cells from these early vessels is termed angiogenesis.2 Although retinal vascular development was initially thought to proceed by vasculogenesis, more recent investigations2–4 indicate that, at least in some species (mouse, primates including humans), angiogenesis plays a highly important role. By these definitions, all pathologic retinal neovascularization involves angiogenesis. There has been a considerable amount of recent work dealing with the recruitment of bone marrow–derived stem cells into both retinal and choroidal neovascular lesions in experimental animals, which, by the definition above, indicates that vasculogenesis may also be involved in pathologic neovascularization.5–7 How frequently this actually occurs in retinal and choroidal neovascularization that is observed clinically in humans is, however, unknown (Box 2.1).
BOX 2.1 Retinal Neovascularization Cascade
The flow chart below describes the mechanisms of retinal angiogenesis. The capillary nonperfusion results in hypoxia, which then upregulates the expression of growth factors either directly or through HIF-1. Growth factors then increase the expression of integrins and proteinases, which results in endothelial cell migration. These factors also promote endothelial cell proliferation directly. Both cell migration and proliferation are essential steps in angiogenesis. There is a critical balance between angiogenesis promoters and endogenous inhibitors. Any destabilization in this balance may lead to angiogenesis.
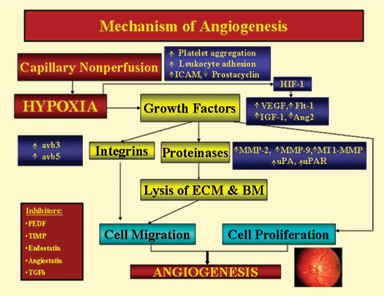
Reproduced from Das A, McGuire P. Retinal and choroidal angiogenesis: pathophysiology and strategies for inhibition. Progr Ret Eye Res. 2003; 22:721–748. Copyright (2003) with permission from Elsevier.
ROLE OF GROWTH FACTORS
IN PROMOTING NEOVASCULARIZATION: VEGF
Both normal retinal vessels and pathologic neovascularization develop through the elaboration of specific peptides, called growth factors, that are elaborated primarily by nonvascular cells and stimulate the proliferation of vascular endothelium. While there are a number of growth factors that are elaborated in the retina and that appear to contribute to the process, the most prominent of these is vascular endothelial growth factor-A (VEGF-A), a family of polypeptides derived from a single gene but with alternative splicing, resulting in several isoforms having different molecular weights and with somewhat different properties.8,9 Other closely related peptides are VEGF-B, -C, and -D and placental growth factor, all of which are mitogens for vascular endothelial cells, though with much reduced potency by comparison with VEGF-A. There are three cell membrane receptors for VEGF-A, designated VEGFR-1, VEGFR-2, and VEGFR-3. Of these, VEGFR-2, also designated Kdr (kinase domain receptor), is the one responsible for both normal and abnormal vascular proliferation.
It has long been recognized, in part on the basis of clinical findings in diseases in which retinal neovascularization occurs (Figs. 2.1A, B and 2.2A,B–C), that such neovascularization occurs largely adjacent to regions of retinal ischemia.10,11 One of the early findings regarding the production of VEGF is that it is strongly upregulated by hypoxia.12,13 A similarity of pathologic neovascularization in the adult retina and fetal development of the retinal vessels is, therefore, that both seem to be mediated by hypoxia, with the hypoxic stimulus arising from neurons in the immature retina stimulating glial cells to produce VEGF and the VEGF-producing glia leading the advancement of the vascular precursors through the fetal retina. Similarly, in the retinas and optic nerves of humans with diabetes and of animals with experimental galactosemia (a condition that produces a diabetic-like retinopathy), VEGF is substantially upregulated in glial cells,14–16 which in turn stimulates production of VEGFR-2 (Figs. 2.3 and 2.4) in retinal vascular endothelial cells.17 With maturation of the fetal retinal vasculature, the hypoxic stimulus is reduced and VEGF production decreases.16–18 Because pathologic neovascularization in the adult retina does not revascularize the hypoxic tissue, but rather migrates inward through the inner limiting membrane of the retina and onto this inner retinal surface, or into the vitreous cavity, the hypoxic stimulus in such cases continues unless it is aborted either by some therapeutic intervention or by another process.
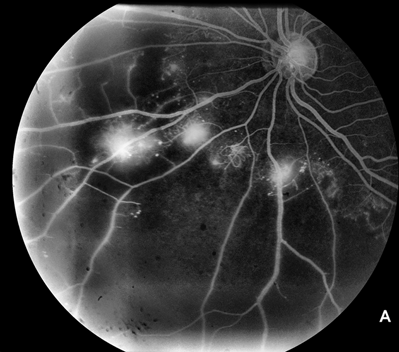
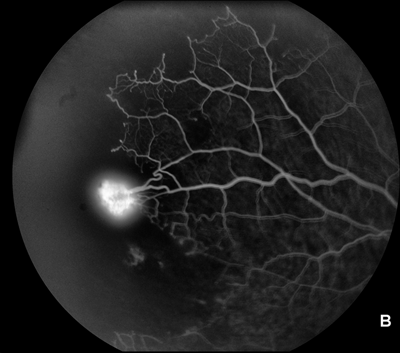
FIGURE 2.1. A: Frame from a fluorescein angiogram in a case of proliferative diabetic retinopathy showing new vessels (areas of intense dye leakage) next to nonperfused area, which appears black at the lower right of the image. B: Frame from a fluorescein angiogram in a case of proliferative sickle retinopathy. Note the intense leakage from the single neovascular tuft and the entirely nonperfused (black) peripheral retina in the portion of the photograph to the left. Note also the arteriovenous shunts, typical of sickle cell retinopathy, at the border of the nonperfused area.
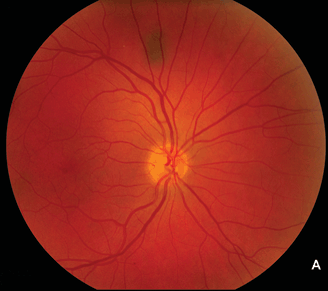
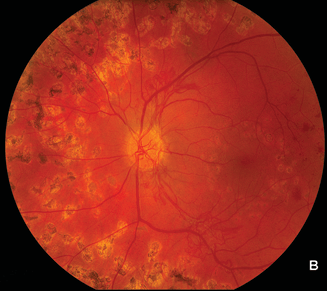
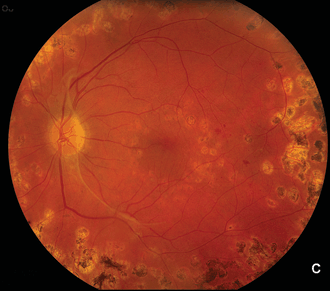
FIGURE 2.2. A: Normal right eye of a 50-year-old woman with a 12-year history of type 2 diabetes. Her left eye (B) had proliferative retinopathy with neovascularization of the optic nerve head that increased despite extensive panretinal argon laser photocoagulation. Subsequently, she was found to have an atherosclerotic lesion that greatly reduced blood flow through the left common carotid artery. She underwent successful carotid endarterectomy, following which the neovascularization dramatically regressed (C). Presumably, retinal hypoxia due to the carotid atherosclerosis was an important contributor to her persistent proliferative diabetic retinopathy.
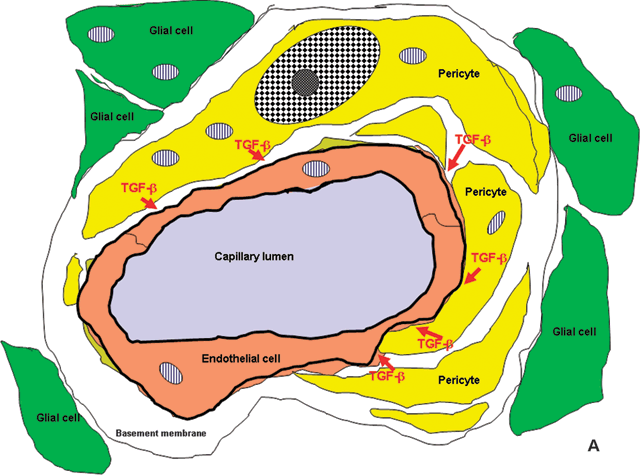
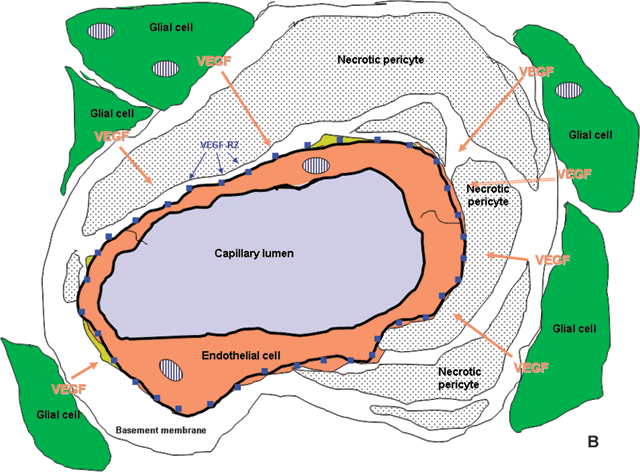
FIGURE 2.3. A: Diagram adapted from an electron micrograph of a human retinal capillary. Pericyte processes, shown in yellow, envelop the endothelial cell tube, shown in beige. The pericytes, together with the endothelial cells, produce transforming growth factor-β, which acts to prevent endothelial cell proliferation. Pericytes and endothelial cells of the capillaries are surrounded by a thick basement membrane, which is not shown in color, and the entire capillary is enveloped in glial processes (green). B: In the diabetic retina, pericytes are lost, removing the inhibitory stimulus and, perhaps, contributing to reduced blood flow with consequent hypoxia to regions of the retina. The hypoxic glial cells produce VEGF, which upregulates the receptor, VEGFR-2, on endothelial plasma membranes stimulating endothelial proliferation and neovascularization.
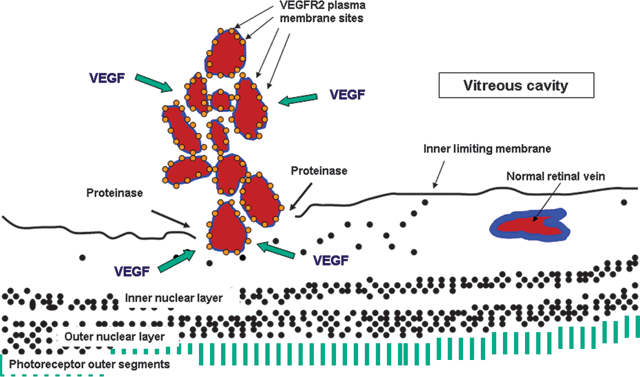
FIGURE 2.4. Drawing adapted from a light micrograph of a human eye with retinal neovascularization. The outer retinal layers are relatively intact, but the ganglion cell layer is somewhat disorganized. The hypoxic retina, in particular its glial cells, is producing greatly increased amounts of VEGF, which stimulates neovascularization. The new vessels grow inward, and the proteinases they produce allow them to digest the inner limiting membrane and break through into the vitreous.
The hypoxic stimulus to VEGF production appears to be mediated by hypoxia inducible factor (HIF-1), a transcription factor that is itself highly regulated by tissue oxygenation and binds to the hypoxia response element of the VEGF gene, thereby stimulating upregulation of VEGF protein.19 The importance of VEGF, and HIF-1, to fetal development is evident from the fact that fetal mice with knockouts either of the VEGF gene or of the HIF-1 gene die in utero because they are unable to develop a vascular system.20,21 Although hypoxia itself is a major stimulus to HIF-1 production, this transcription factor, and VEGF, can also be upregulated by a variety of molecular stimuli, such as cytokines and other growth factors,19 and perhaps other molecules as well.22–24 In addition to hypoxia, growth factors and cytokines that are increased in the inflammatory process may, therefore, also contribute to retinal neovascularization in diabetic retinopathy and in inflammatory diseases that involve the retina and choroid.25–27
Even after long duration (>25 years) of type 1 diabetes, proliferative retinopathy appears in not more than 50% to 60% of diabetic individuals.28 This, together with evidence from epidemiologic studies that there is familial clustering both of diabetic retinopathy and of nephropathy,29–31 indicates the likelihood of a genetic predisposition to these complications of the disease. Attempts to determine what gene(s) may be involved, through genome-wide scans of multiplex families of several different ethnicities with proliferative or severe nonproliferative retinopathy and nephropathy, using genome-wide scanning and other methods, are still underway.31 Several reports have appeared describing the association of specific single nucleotide polymorphisms (SNPs) and haplotypes within the VEGF gene with proliferative and severe nonproliferative diabetic retinopathy.32–34 A problem with these and several other papers on the same topic is that they are not all in agreement about the particular SNPs and/or haplotypes that each study is reporting as associated with proliferative and severe nonproliferative disease, even with quite high probability values. Of particular interest is the report from the very large and well-characterized DCCT/EDIC study cohort, in which several VEGF gene variants in this population with type 1 diabetes were highly associated with proliferative and severe nonproliferative diabetic retinopathy, but not with diabetic macular edema. Although VEGF has been strongly linked to proliferative diabetic retinopathy for a number of years,35,36 there has also been considerable recent interest in a pathogenic role for VEGF in diabetic macular edema.37–39 This derives from the initial discovery of VEGF (then called “vascular permeability factor”) as a molecule that enhanced permeability of blood vessels.40–42 The role of VEGF in macular edema in diabetes and other retinal diseases may be more complex than it is in retinal neovascularization, however. Questions that must be resolved are, first, the observations of Al-Kateb et al.32 that SNPs and haplotypes of the VEGF gene that are significantly associated with proliferative and severe preproliferative diabetic retinopathy are not associated with macular edema and, second, that macular edema may occur in diabetes and other diseases without retinal neovascularization and, conversely, that neovascularization may occur without macular edema.43
VEGF, THE BLOOD-RETINAL BARRIER, AND PROTEINASE ACTIVITY
The breakdown of the blood-retinal barrier, and the proliferation of retinal new blood vessels, require the production of enzymes or other substances that can, first, break down the junctional complexes that form the gates between retinal vascular endothelial cells or retinal pigment epithelial cells, thereby separating retinal vascular lumina or the choriocapillaris and the neural retina, and, second, digest the basement membranes that encase the endothelial cells and pericytes or smooth muscle cells of the retinal blood vessels, or that comprise the inner limiting membrane that comprises the boundary between the neural retina and the vitreous (Fig. 2.4). VEGF stimulates an initial step in blood-retinal barrier breakdown by promoting the phosphorylation of specific sites on the cellular tight junction proteins zonula occludens-1 and occluding.44,45 Second, VEGF upregulation in the hypoxic retina stimulates production of matrix metalloproteinase enzymes (MMPs)46,47 that enhance blood-retinal barrier breakdown.48 These enzymes (MMPs) have been reported in excess in neovascular membranes from the retinas of diabetic humans,49 and experimental retinal neovascularization can be suppressed by MMP inhibitors.50
Other Growth Factors that May Stimulate Neovascularization
Although VEGF has been the most widely studied growth factor involved in retinal neovascularization, there has been considerable interest in other growth factors in this process. The upregulation of insulin-like growth factor-1 (IGF-1) following the imposition of “tight” blood glucose control in previously poorly controlled individuals with type 1 diabetes and early retinopathy has been claimed to be responsible for the “early worsening” of retinopathy that has been observed in that situation.51 However, Wang et al.52 have reported that serum IGF-1 levels did not correlate with proliferative diabetic retinopathy either in type 1 or in type 2 diabetics in their large, population-based series. The elegant work of Lois Smith and her co-workers strongly suggests that IGF-1 serves primarily as a “permissive” factor for neovascularization occurring in the presence of VEGF, rather than as the principal angiogenic factor itself.53
Angiopoietin-1 (Ang1) and angiopoietin-2 (Ang2), acting through the receptor Tie2, also serve as permissive factors for vascular growth. In a series of transgenic mice that can express Ang1 or Ang2 and VEGF, stimulated by different promoters that are engineered to respond to dosing with the antibiotic doxycycline, Campochiaro and colleagues54 have been able to show differential regulation of vascular sprouting from the superficial or deep retinal capillary beds, governed by the expression of Ang1 or Ang2 at different postnatal times during the development of the retinal vasculature in these mice. Although a role for Ang1 or Ang2 in the adult eye, or in pathological processes such as the proliferative retinopathies, has not been delineated, Campochiaro speculates that increased expression of one of these molecules may be necessary to permit neovascularization in the retinas of diabetic humans or experimental animals, in which VEGF has been upregulated but new vessels have not appeared.8,15,54 Additional evidence for permissive, or accessory, roles for Ang2 and the Tie2 receptor in retinal neovascularization has been presented by other authors.55,56
Members of the fibroblast growth factor family were initially thought to be important contributors to retinal neovascularization. However, subsequent work and a variety of arguments (summarized in Ref. 57) suggest that this is not likely to be the case.
Impressive recent work53,58,59 indicates that erythropoietin contributes importantly to the pathogenesis of proliferative diabetic retinopathy and, perhaps, to other proliferative retinopathies. A single SNP in the promoter of the erythropoietin gene was found, in three separate patient series, to be significantly associated with proliferative diabetic retinopathy and with end-stage diabetic nephropathy.59
INHIBITORY GROWTH FACTORS
Using the technique that they had developed for preparing flat mounts of the isolated human retinal vascular tree following digestion of the neural retina with concentrated trypsin solutions, Cogan, Kuwabara and their colleagues in the 1960s showed that an early vascular lesion in eyes from diabetic human subjects was loss of the pericytes, or “mural cells,” from the retinal capillaries.60 Selective early loss of pericytes in diabetic eyes has been considered a cardinal lesion of early diabetic retinopathy, although Kern61 has recently argued that capillary pericyte loss is not selective and that both pericytes and endothelial cells are lost equally in diabetic retinas. While this argument may be valid with consideration to vascular cell loss over the entire retina, it is certainly the case that there are some regions in which pericyte loss precedes that of endothelial cells, as Kuwabara and Cogan initially reported. While the metabolic processes that lead to this cellular loss are still unclear, it has been demonstrated that cells of the retinal capillaries in diabetic humans and laboratory animals do undergo apoptosis or programmed cell death.62 Subsequent work has shown that retinal neurons and glia in diabetic eyes also undergo apoptosis,63 a process that must be extremely slow, or else the cellular content of the retinas of diabetic individuals would soon become depopulated.
What are the consequences of capillary cell loss? Studies of trypsin digest preparations of human eyes in which, fortuitously, fluorescein angiograms had been performed shortly before the individual’s death have demonstrated that capillaries that have become totally acellular and are recognizable only as empty basement membrane tubes do not carry blood and therefore contribute to the retinal ischemia that is a stimulus to VEGF upregulation and neovascularization.64 However, the selective removal of pericytes appears to remove an inhibitory “brake,” permitting endothelial cells to proliferate, as Orlidge and D’Amore65 showed some years ago. They subsequently showed that the inhibitory molecule was another growth factor, transforming growth factor-β (TGF-β; Fig. 2.3A).66 Microaneurysms would, therefore, appear to be localized endothelial cell proliferations, likely in areas where pericytes have been lost (Fig. 2.3B).
While details of the mechanism(s) by which pericytes and endothelial cells eventually undergo apoptosis in the retinas of diabetics are not completely clear, a finding that appears to be relevant is that in fetal mice in which the gene for platelet-derived growth factor (PDGF) has been knocked out, capillary pericytes do not develop and the fetus dies.67 In the developing fetal eye, PDGF, therefore, appears to be important for recruiting pericytes to the developing retinal vessels and for subsequent maintenance of the vasculature.68 Whether hyperglycemia or other metabolic abnormalities in the diabetic eye interfere with PDGF production or its interaction with the cells of the retinal capillaries, leading to pericyte loss, has not been described.
Another inhibitory growth factor is pigment epithelium–derived factor (PEDF). Although many cells throughout the retina produce PEDF, its major site of biosynthesis is, as its name implies, the retinal pigment epithelium (RPE).69 In addition to its ability to inhibit blood vessel proliferation, PEDF also appears to facilitate retinal neuronal differentiation.70 The RPE is a major site of synthesis of both PEDF and VEGF, and both of these molecules are released constitutively by normal RPE cells but, remarkably, in opposite directions (Fig. 2.5). VEGF is released from the basal surface of the RPE layer,71 thereby facilitating the maintenance of the choriocapillaris and also the asymmetrical anatomy of the choriocapillary endothelium, with a thin, fenestrated surface facing the RPE and a thicker endothelial lining facing the deeper layers of the choroid. VEGF interacts with cellular junction proteins,44,45 leading to their disruption with breakdown of the blood-retinal barrier, and facilitates the development of endothelial fenestrations.72 By contrast, PEDF is released from the apical surface of RPE cells,73 which may be the reason that the outer layers of the vertebrate retina normally are avascular and also, perhaps, explains why retinal neovascularization nearly always grows inward, toward the inner limiting membrane and the vitreous. Could exceptions,74–76 in which the new vessels grow outward, toward the RPE, be the result of aberrant secretion of PEDF and/or VEGF in localized regions of the RPE?
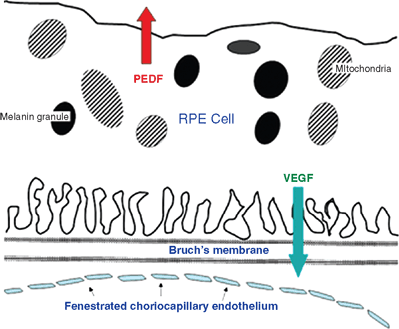
FIGURE 2.5. Drawing made from an electron micrograph, showing polarized secretion of VEGF from the basal plasma membrane of the RPE cells toward the choriocapillaris, maintaining the anatomy and function of that vascular layer and inducing the asymmetrical choriocapillary plasma membrane, which is fenestrated on the retinal side. PEDF is secreted from the apical side of the PRE, presumably acting to maintain the normal avascularity of the outer retina.
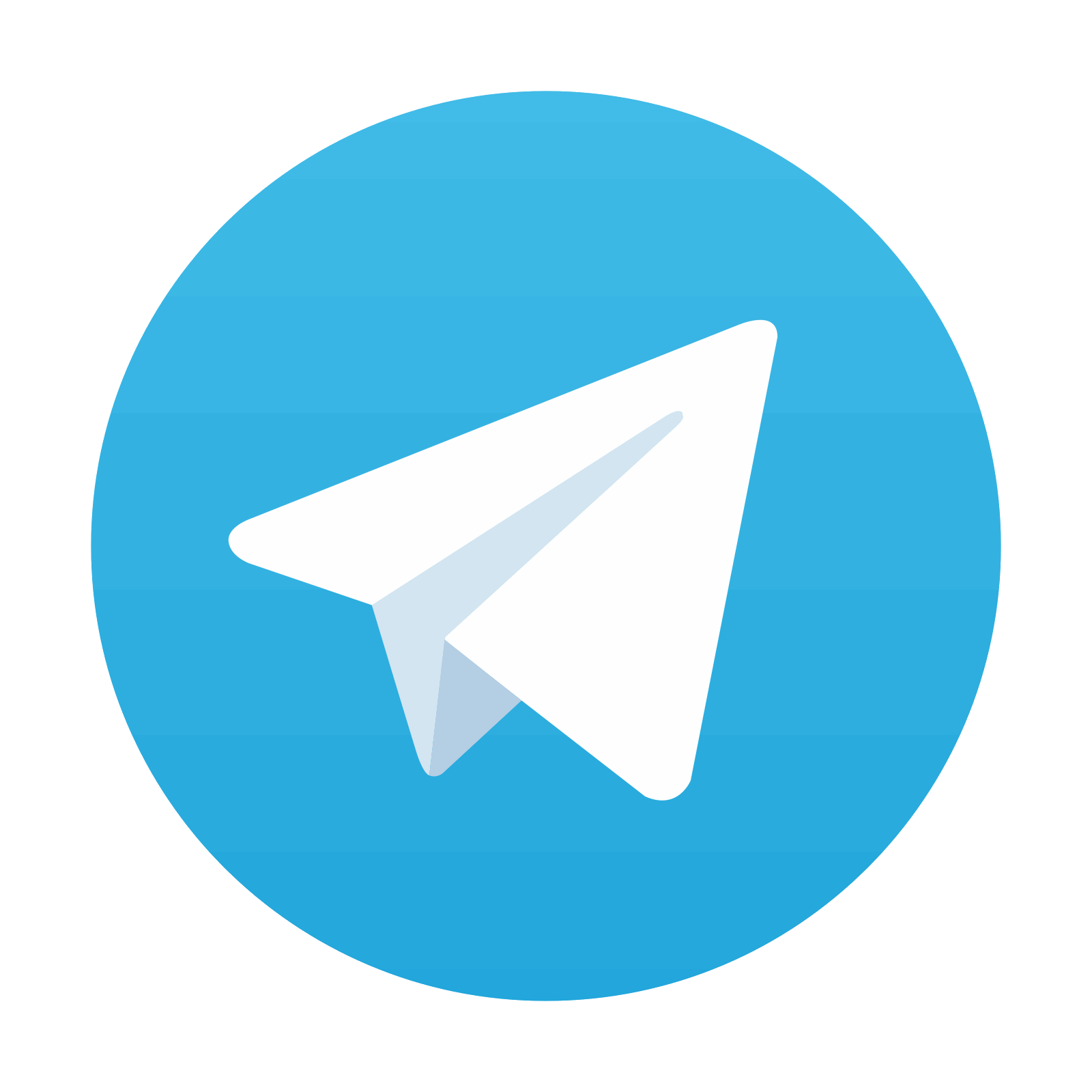
Stay updated, free articles. Join our Telegram channel

Full access? Get Clinical Tree
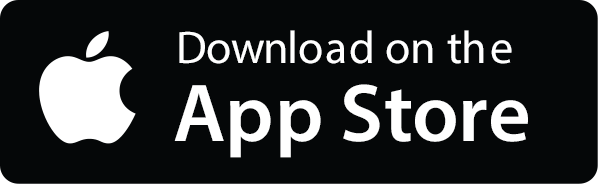
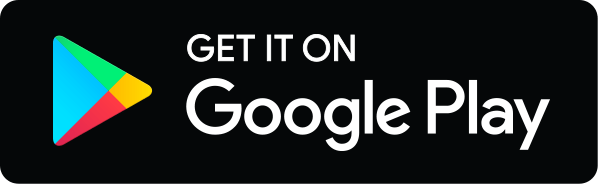