of Prematurity
Tushar Ranchod • Antonio Capone,Jr.
INTRODUCTION
Retinopathy of prematurity (ROP) remains a leading cause of blindness worldwide.1 ROP is characterized by avascular peripheral retina, intraocular dysregulation of vascular endothelial growth factor (VEGF), and pathologic vasculogenesis.
Retinal vascular development begins prior to the fourth month of gestation, with vessels emanating from the optic nerve and growing steadily toward the ora serrata as the fetus approaches term.2 This process may be interrupted by premature birth, resulting in a spectrum of abnormalities known as ROP. At the mildest end of the spectrum, retinal vascular growth may slow down before resuming a normal growth pattern. At the most severe end of the spectrum, normal vascular growth within the plane of the retina ceases and aberrant vasculature grows in the form of intraretinal shunt vessels as well as neovascular networks extending into the vitreous gel. This neovascularization can mature into fibrovascular preretinal tractional bands, which result in tractional retinal detachment.
VASCULAR ABNORMALITIES IN ROP
Intraretinal shunting in ROP occurs along the border of vascular and avascular retina.3 If persistent, the changes in vascular flow eventually cause vascular tortuosity upstream and downstream until peripapillary tortuosity and venous dilatation occur and are identified as “plus” disease (Fig. 17.1). The presence of plus disease is therefore a proxy for persistent VEGF dysregulation and aberrant retinal vascularization.
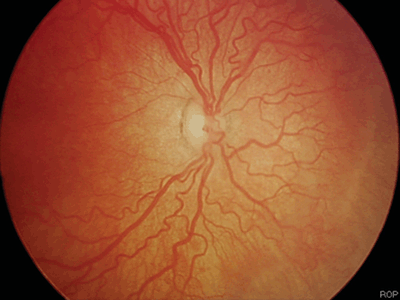
FIGURE 17.1. Plus disease. Intraretinal vascular shunting may progress to peripapillary vascular tortuosity and venous dilatation known as “Plus” disease.
The pathogenesis of extraretinal neovascularization in ROP shares common characteristics with proliferative retinal neovascularization seen in diabetes mellitus, retinal vascular occlusions, and a variety of other retinal diseases. Retinal ischemia from a variety of causes may result in elevated intravitreal VEGF levels, which in turn stimulate retinal neovascularization.4,5
CLINICAL STAGES OF ROP
The clinical stages of ROP have been described in the International Classification of Retinopathy of Prematurity (ICROP), which was updated most recently in 2005.6 Stage 1 is a discrete demarcation line between vascular and avascular retina. Stage 2 is a ridge, which rises above the plane of the retina between vascular and avascular retina (Fig. 17.2). Stage 3 is fibrovascular proliferation extending from the ridge into the preretinal vitreous gel (Fig. 17.3). Fibrovascular traction may progress to a tractional retinal detachment. Stage 4 denotes a partial retinal detachment, with Stage 4a denoting a detachment sparing the fovea and Stage 4b involving the fovea (Fig. 17.4). Stage 5 describes a total retinal detachment.
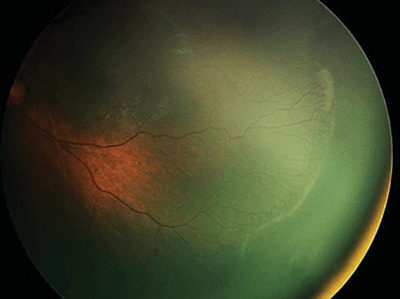
FIGURE 17.2. Stage 2 ROP. In stage 2 disease, a ridge rises above the plane of the retina between vascular and avascular retina. Intraretinal shunt vessels lie within the ridge.
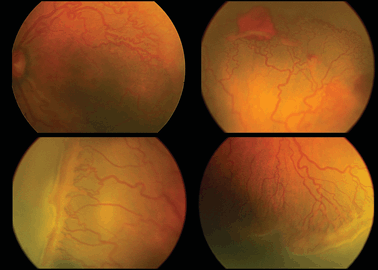
FIGURE 17.3. Stage 3 ROP. Stage 3 is comprised of fibrovascular proliferation extending from the ridge into the vitreous gel.
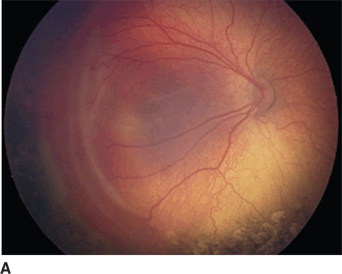
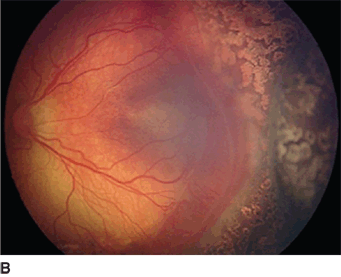
FIGURE 17.4. The images ((A) right eye top, (B) left eye bottom) show bilateral Stage 4b, or macula-involving, detachments, which extend posteriorly from the temporal ridges with neovascularization.
The stages of ROP are described by the number of clock hours involved as well as the zones in which they occur. The retina is divided into Zone 1 posteriorly, which is centered on the optic disc and extends as a circle with a radius equal to twice the distance from the disc to the fovea. Zone 2 extends from the edge of Zone 1 to the nasal ora serrata. The residual crescent of retina remaining temporally is Zone 3.
“Plus” disease is an additional critical variable in the description of ROP. As mentioned above, intraretinal shunts may form within the ridge between vascular and avascular retina, and the resulting vascular flow abnormalities eventually cause dilatation and tortuosity of the peripapillary retinal vessels.
In the latest update of the ICROP, aggressive posterior ROP (APROP) is classified as a discrete form of ROP. APROP progresses rapidly with vascular tortuosity and dilatation in all four quadrants, usually a Zone 1 or posterior Zone 2 location, and frequent progression to Stage 5 ROP if untreated.6
INCIDENCE OF ROP
Not all premature infants develop ROP, and the development of ROP is best predicted by low birthweight and early gestational age. The incidence of ROP is approximately 68% in infants born below 1,250 g, with the incidence rising to 93% of infants born below 750 g. Similarly, the incidence of ROP is approximately 14% in infants born after 32 weeks gestational age, and the incidence rises to 89% in infants born before 27 weeks.7
Most newborns with ROP develop manifestations of disease at fairly predictable intervals, with initial manifestations around 32 weeks postmenstrual age (PMA). Infants who progress to the threshold for ablative treatment usually do so by 37 weeks PMA.8–11 Approximately 10% of infants with ROP ultimately require treatment, and of the group requiring treatment, approximately 10% progress to retinal detachment despite sufficient laser treatment.12,13 The exception to this relatively predictable timeline of disease development is a subset of patients with APROP, which progresses early and rapidly to retinal detachment despite timely ablation.6
CURRENT MANAGEMENT OF ROP
Infants who reach a treatment threshold of ROP are currently managed with ablation of avascular retina (Box 17.1). Cryotherapy was initially used in the Cryotherapy for Retinopathy of Prematurity (CRYO-ROP) trial, but cryotherapy was supplanted by laser photocoagulation in later trials such as the Early Treatment of Retinopathy of Prematurity (ETROP) study. More recent trials have demonstrated superior visual and structural outcomes with laser, which has replaced cryotherapy as the standard-of-care means of peripheral retinal ablation9,13–15 (Fig. 17.5).
BOX 17.1 Pivotal Trials of Peripheral Retinal Ablation for ROP
CRYO ROP Study: CRYO-ROP
- Purpose: To examine the role of cryotherapy in preventing severe vision loss in stage 3 ROP infants.
- Method: In this randomized, prospective study, ROP babies with five or more contiguous, or eight cumulative 30 degree sectors (clock hours) of stage 3+ ROP in Zone 1 or 2 were treated with transscleral cryotherapy to the avascular retina (applied in one eye when there was threshold retinopathy in both eyes, or in half the eyes when the threshold severity existed in only one eye). An unfavorable outcome was defined as posterior retinal detachment, posterior retinal fold (usually involving the macula), or retrolental tissue that obscured the view of the posterior pole.
- Results: At 12 months, whereas 43% of untreated eyes had an unfavorable outcome, there was significantly less unfavorable outcome in the eyes that received cryotherapy (21.8%).
- Conclusions: These data support the efficacy of cryotherapy in reducing the risk of unfavorable ocular outcome from threshold ROP. It is recommended that cryotherapy be considered for both eyes whenever stage 3+ ROP involves the posterior retina (Zone 1) of both eyes. (Palmer, Doc Ophthalmol. 1990;74:245.)
ET ROP Study: Early Treatment for ROP
- Purpose: To examine the efficacy of ablative treatment in ROP infants with bilateral high-risk prethreshold disease.
- Methods: One eye was randomized to early retinal ablative treatment (mostly laser, some cryo) and the fellow eye managed conventionally (control eye). The primary outcome was visual acuity assessed by masked testers using the Teller acuity card procedure. Structural examinations were performed at 6 and 9 months.
Results: Grating acuity results showed a reduction in unfavorable visual acuity outcomes with earlier treatment, from 19.8% to 14.3%. Unfavorable structural outcomes were reduced from 15.6% to 9.0% at 9 months. Further analysis supported retinal ablative therapy for eyes with type I ROP, defined as Zone 1, any stage ROP with plus disease; Zone 1, stage 3 ROP without plus disease; or Zone 2, stage 2 or 3 with plus disease.
- Conclusion: Early treatment of high-risk prethreshold ROP significantly reduced unfavorable outcomes in both primary and secondary (structural) measures. (Good ETROP Group,, Trans Am Ophthalmol Soc. 2004;102:233.)
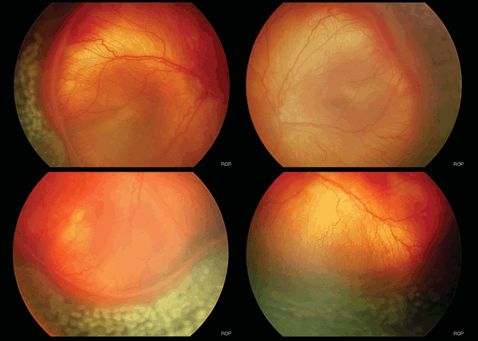
FIGURE 17.5. Laser peripheral laser ablation for threshold ROP. Current standard of care treatment for threshold ROP is thermal laser ablation of the retinal periphery from the ora serrata to the border of vascular and avascular retina.
Most cases of disease progression following laser ablation result from inadequate treatment or a delay in treatment after reaching threshold. Even in experienced hands, complete ablation in a single session may be difficult. The border between vascular and avascular retina may be particularly difficult to identify in cases of aggressive posterior disease, due to so-called flat neovascularization characterized by velvety and indistinct edges.16 Consequently, a second session of laser photocoagulation is often appropriate in eyes with APROP in order to identify and treat any residual skip areas.
Treatment from the ora serrata to the border of vascularized retina is critical, but complete ablation by laser or cryotherapy is not always possible. In some cases, complete laser ablation may be limited by the surgeon’s view (Fig. 17.6). Iris neovascularization and persistent tunica vasculosa occur more commonly in advanced cases of ROP and APROP and may contribute to poor pupillary dilation. The surgeon’s view may be limited by hyphema due to iris neovascularization or by vitreous or subhyaloid hemorrhage due to retinal neovascularization, thereby precluding complete laser ablation.
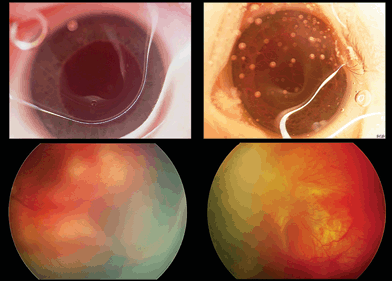
FIGURE 17.6. Poor pupillary dilation or media opacities may prevent the direct visualization needed to deliver peripheral ablation. Diffuse vitreous hemorrhage (upper left) may completely obscure the fundus. Persistent tunica vasculosa lentis (upper right) may contribute to poor pupillary dilation. Focal or sectoral vitreous hemorrhage (lower left and right) may allow incomplete peripheral ablation.
Under ideal circumstances, only about 1% to 2% of newborns with ROP should experience poor structural outcomes, since adequate laser limited poor outcomes to 9% of cases in the ETROP trial, and tertiary care centers have reported good structural and visual outcomes in approximately 90% of cases, which progress to subtotal retinal detachment.7,17–19 The actual percentage of poor outcomes may remain higher than 2% due to limitations in manpower, an inability to predict which newborns will develop severe disease, and an incomplete arsenal of treatment options to cover all clinical scenarios. The last few years have seen an increased understanding of the pathophysiology of ROP, including the role of VEGF and the potential use of antiangiogenic pharmacologic therapies in the treatment of ROP.
EFFECT OF PERIPHERAL ABLATION ON VEGF LEVELS
The clinical staging of ROP has been used in clinic trials to determine the threshold for peripheral retinal ablative treatment. The CRYO-ROP study demonstrated treatment benefits based on a threshold of five contiguous or eight noncontiguous clock hours of stage 3 disease. The ETROP subsequently redefined the threshold for treatment as Zone 1 disease of any stage with plus disease; stage 3 in Zone 1 regardless of plus disease; or stage 2 or 3 disease in Zone 2 in the presence of plus disease.
Animal models of ROP have demonstrated that the avascular peripheral retina is responsible for producing abnormally high VEGF levels associated with ROP.20 Presumably, laser photocoagulation of peripheral avascular retina decreases VEGF production by ablating hypoxic tissue and thereby reducing the stimulus for VEGF production.3 Clinically, regression of ROP is usually seen following peripheral laser ablation, with resolution of plus disease and regression of fibrovascular proliferation.
Peripheral ablation is not universally effective in eliciting regression of ROP. This is particularly true for APROP, which typically afflicts profoundly premature neonates.6 In this subset of infants, progression of ROP to bilateral retinal detachment and blindness may occur despite timely and complete peripheral retinal ablation.
ROLE OF VEGF IN ROP: A TWO-STAGE PROCESS
Vascularization of the human retina begins early in the second trimester, extending radially from the optic disc and reaching the retinal periphery by approximately 36 to 40 weeks of gestation.2 VEGF plays a crucial role in this process. As the neurosensory retina matures and metabolic demands increase, a relative hypoxia occurs in the avascular peripheral retina. VEGF is released by the avascular tissue, and the resulting VEGF gradient drives retinal vascularization toward the retinal periphery.21,22
VEGF dysregulation in the premature infant occurs in two stages. In the first stage, retinal vascular maturation slows as intravitreal VEGF levels decrease in the presence of the relatively hyperoxic ex utero environment. In the second stage, retinal vasculature grows aberrantly as endogenous and intravitreal VEGF levels increase secondary to prolonged persistence of avascular retina.3
Upon delivery, the newborn enters a relatively hyperoxic environment compared to the womb. Because complete retinal vascularization does not occur until full term, premature infants are delivered into a hyperoxic environment while the peripheral retina remains incompletely vascularized.21 Hyperoxia acts to downregulate VEGF produced by the avascular peripheral retina, and the normal process of retinal vascularization is impeded. In most premature infants, retinal vascularization is delayed but eventually resumes without significant sequelae. However, in some infants, the prolonged tissue hypoxia experienced by avascular retinal periphery causes an abnormal increase in VEGF production.4,22 Instead of resuming the normal maturation process, pathologic angiogenesis occurs and intraretinal shunting and extraretinal neovascularization may develop.
The two-stage dysregulation hypothesis of VEGF in ROP is based in part based on animal models. The first stage of VEGF dysregulation was clearly demonstrated by Pierce et al.23 in a mouse model. Mice placed in a hyperoxic environment at 7 days of age displayed decreased retinal VEGF expression and also developed vascular obliteration. Intravitreal injection of VEGF prevented vascular obliteration, thereby confirming VEGF downregulation as the proximal source of vascular dysgenesis.
The progression of retinal neovascularization (the second stage of ROP), mediated by VEGF, has been demonstrated in a rat model. Intravitreal injection of anti-VEGF antibodies significantly reduced retinal neovascularization compared to fellow eyes of rat pups exposed to elevated oxygen levels after birth.24
RELATIONSHIP OF OXYGEN AND VEGF LEVELS
When ROP was first described, the disease was attributed to high levels of neonatal oxygen exposure. A hypoxic stimulus for neovascularization was proposed, and oxygen was considered a possible treatment modality for ROP. However, the Cooperative Study of Retrolental Fibroplasia in 1956 demonstrated that administration of 50% oxygen to premature infants for 4 weeks actually increased rates of ROP and vision loss compared to lower oxygen delivery.25
Evidence for a hypoxic stimulus gained traction again as research demonstrated a relationship between hypoxia and angiogenic stimuli. The Supplemental Therapeutic Oxygen for Prethreshold ROP (STOP-ROP) study examined whether supplemental oxygen might decrease the rate of prethreshold ROP.8 Premature infants with oxygen saturations <94% on room air were randomized to a conventional oxygen saturation target (89%–94%) or supplemental oxygen with an increased target (96%–99%). A total of 649 infants were enrolled in the study. The oxygen supplementation group demonstrated a slightly lower rate of progression from prethreshold to threshold ROP (48% vs. 41%), but this difference was not statistically significant. A subgroup analysis of patients without plus disease showed a more pronounced decrease in disease progression (32% vs. 46%), suggesting that oxygen supplementation might reduce disease severity if given early enough. However, the STOP-ROP study also highlighted possible adverse effects of aggressive oxygen supplementation. The oxygen supplementation group demonstrated an increased incidence of pneumonia and exacerbation of chronic lung disease, stayed in the hospital longer, and remained on diuretics for longer compared to the conventional target group.
Studies such as STOP-ROP accounted for the hypoxic drive for retinal neovascularization mediated by VEGF, which is now understood to comprise the second stage of ROP. The first stage of the disease, however, results from the relatively hyperoxic ex utero environment, which impedes retinal vascular growth in the premature neonate. As appreciation grew for the first stage, several studies examined whether lower oxygen saturations might reduce the incidence of ROP in premature infants.
A study by Chow et al.26 in 2003 examined the incidence of ROP before and after the institution of tighter guidelines for oxygen administration in premature infants with birthweight <1,250 g. The new guidelines aimed to reduce hyperoxic and hypoxic fluctuations with a target oxygen saturation of 85% to 93%. The incidence of stage 3 and 5 ROP dropped from 12.5% to 2.5% after adoption of the new protocol, supporting the role of oxygen fluctuations in stimulating ROP.
Studies by Wright et al. in 2006 and Tokuhiro et al. in 2009 examined the incidence of ROP after adoption of reduced oxygen protocols, and both found reductions in threshold ROP with the newer reduced oxygen saturation targets.27,28 These studies taken together supported the theory that lower oxygen saturation targets more closely mimic the in utero environment and, thereby, limit the hyperoxic stimulus in the first stage of ROP.
The two-stage theory of ROP implies that lower oxygen targets in the immediate postnatal period might allow retinal vascularization to proceed, while higher oxygen targets might inhibit VEGF-induced neovascularization as premature infants reach term. Sears et al.29 examined this hypothesis by comparing the incidence of ROP before and after the adoption of a protocol for reduced oxygen (target oxygen saturation of 85%–92%) prior to 34 weeks PMA and elevated targets (92%–97%) after 34 weeks PMA.29 The incidence of ROP decreased from 35% to 13% and the incidence of threshold disease decreased from 7% to 1% with the new protocol.
FACTORS OTHER THAN VEGF
While the role of VEGF in ROP is supported by a large body of literature, several other factors crucial to disease development have been elucidated recently. Consequently, the two stages of disease progression are increasingly understood in terms of multiple growth factors, both independent and interdependent.
Erythropoietin (Epo), a growth factor secreted by the fetal liver and adult kidney, mediates retinal angiogenesis using a pathway apparently independent from that of VEGF. Like VEGF, Epo is oxygen sensitive, with downregulation in the presence of hyperoxia and upregulation in the presence of hyperoxia.30 The delivery of Epo to premature infants for the treatment of anemia resulted in increased incidence of treatment-requiring ROP, just as one might expect with the exogenous delivery of VEGF during the second phase of ROP development.31 Modulation of Epo may prove beneficial in the treatment or prevention of ROP if these initial findings are borne out in further studies.
Insulin-like growth factor-1 (IGF-1), a growth factor transmitted maternally to the developing fetus, appears to be necessary for normal angiogenesis. The presence of VEGF is necessary for angiogenesis as well, but VEGF alone is insufficient based on studies in which IGF-1-knockout mice demonstrated deficient retinal vascular development but were rescued by exogenous IGF-1 delivery.32,33 Serum levels of IGF-1 increase as the fetus approaches term, and premature infants, therefore, experience a relative deficiency of IGF-1 in the immediate postpartum period. Since IGF-1 acts as a critical cofactor for VEGF activity, vascular development is inhibited during this period. As the neonate matures and begins producing endogenous IGF-1, VEGF activity ensues and may stimulate retinal neovascularization.34 Modulation of IGF-1 and its related pathways during the immediate postpartum period may play a role in preventing ROP, particularly as the relationship between IGF-1 and VEGF is further clarified.
ANTI-VEGF THERAPY FOR ROP
The advent of anti-VEGF drugs has raised the possibility of treating selected cases of ROP off-label with these medications. The rationale for this approach is drawn from intravitreal VEGF concentration data in human infants with ROP.
In a recently published study, we examined undiluted vitreous samples at the time of surgical repair from the midvitreous of children who developed stage 4 ROP despite standard of care laser.5 At the time of surgery, the eyes were graded and divided into two groups—vascularly active and vascularly inactive—and compared to a control group consisting of children undergoing cataract surgery for uncomplicated congenital cataract. We measured all isoforms of VEGF by enzyme-linked immunosorbent assay (ELISA). Our findings confirmed that upregulation of VEGF occurs in ROP and that abnormally high levels are present in eyes developing retinal detachments with persistent vascular activity. Such eyes are potential candidate for anti-VEGF medications.
As VEGF is required in the developing retina for normal angiogenesis, the goal of treatment is to quench the excessive levels of VEGF in the vitreous rather than to penetrate retinal tissue. Furthermore, as VEGF activity is endogenously downregulated around the time of the due date, it is likely that premature infants will only require one or perhaps two injections of anti-VEGF medications to prevent progression of disease. For this reason, bevacizumab (Avastin, Genentech, South San Francisco, CA) is a more desirable drug than ranibizumab (Lucentis, Genentech, South San Francisco, CA) for treatment of ROP. While the two medications work through an identical mode of action, bevacizumab is a larger molecule than ranibizumab and may, therefore, penetrate the retina to a lesser degree. Since intraretinal VEGF is desired for continued retinal vascular maturation, less retinal penetration is preferable in this case.
STUDIES OF ANTI-VEGF THERAPY FOR ROP
Several small case series in the literature have documented the use of intravitreal bevacizumab in eyes with APROP refractory to peripheral ablation as well as in eyes with threshold or advanced ROP either as primary or adjunctive therapy35,36 (Table 17.1).
Table 17.1.
Reported cases of intravitreal bevacizumab for retinopathy of prematurity (ROP)
Author, Year | Patients | Eyes | Stage of Disease | Dose | Injectionsa | Outcomes After Injection |
---|---|---|---|---|---|---|
Chung37 | 1 | 2 | APROP | 0.75 mg | 1 | Combined with laser OU Regression OU |
Honda39 | 1 | 1 | St3 in Z1, s/p laser | 0.4 mg | 1 | Acute progression of TRD |
Kusaka35 | 14 | 23 | All eyes s/p laser 3 eyes St 3 18 eyes St 4a 2 eyes St 4b | 0.5 mg | 1 | Combined with vitrectomy in 8 eyes Subsequent vitrectomy in 12 eyes Decreased vascularity 14/15 eyes on IVFA New/progressive TRD in 3 patients |
Lalwani40 | 3 | 5 | Case 1: VH OU, no laser Case 2: Z1, plus s/p laser OU Case 3: ERD OU, no laser | 1.25 mg ×1 0.63 mg ×7 | 1–2 | All eyes stable except RRD in one eye Subsequent laser in all eyes |
Martinez-Castellanos41 | 27 | 53 | Group 1: St4 s/p laser Group 2: Threshold, poor view Group 3: Threshold, no laser | 1.25 mg | 1 | Regression all eyes Resolution of ERD without surgery in 1 eye |
Mintz-Hittner38 | 11 | 22 | St 3 in Z1/ posterior Z2 | 0.625 mg | 1 | Regression in all eyes |
Nazari36 | 6 | 12 | APROP, St3, s/p laser | 0.625 mg | 1 | Regression in all eyes |
Nonobe58 | 6 | 6 | 4 eyes St 4b 2 eyes St 5 | 0.75 mg | 1 | All St 4b eyes and 1 St 5 eye attached after one subsequent procedure (SB or lens/vit). One St 5 eye attached after three procedures for RRD. |
a Number of injections per eye.
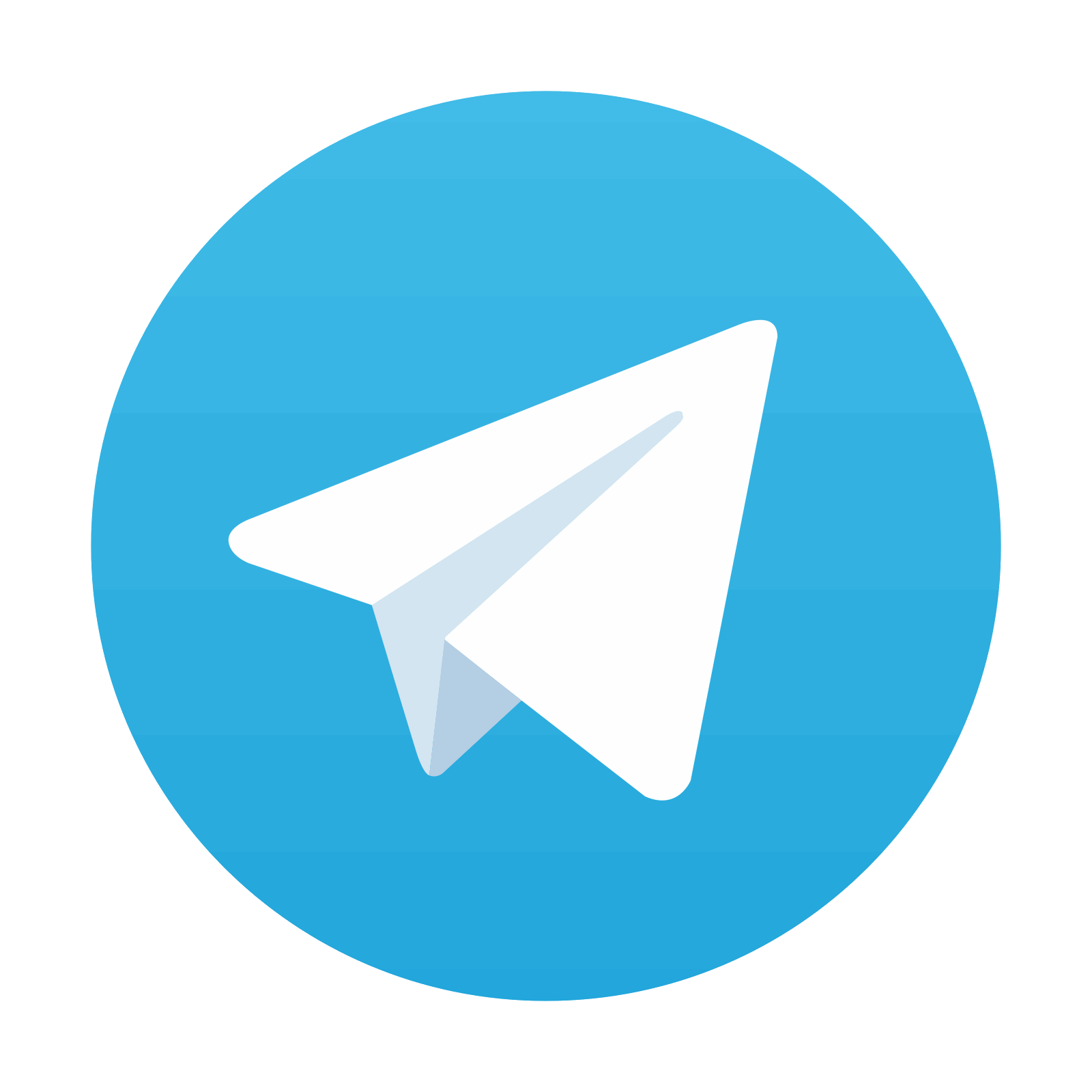
Stay updated, free articles. Join our Telegram channel

Full access? Get Clinical Tree
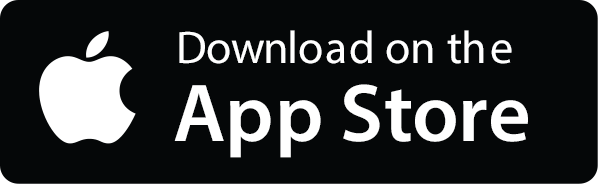
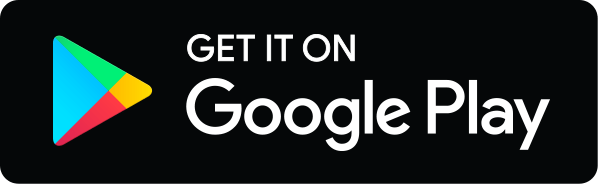