10 Spectral-Domain Anterior-Segment Optical Coherence Tomography in Refractive Surgery
Optical coherence tomography (OCT) is a promising imaging device in refractive surgery because of its real-time, high-speed, and noncontact features. OCT allows for high-resolution, cross-sectional scans and two- and three-dimensional (3-D) reconstructions of the cornea, iris, anterior-chamber angle, and lens as well as 3-D optical biometry. 1 , 2 , 3 , 4 , 5 , 6 , 7 , 8 OCT images are acquired by measuring the intensity and time delay of wave lights diffracted from anatomical structures passing through an established reference path. 8
Spectral-domain OCT (SD OCT) systems use a charge coupled device camera to register the diffraction grating of wave lights returning from the eye structures. The time delay and intensity of the wave lights are processed using a mathematical formula (Fourier transformation). The effective acquisition speed is 26,000 axial scans per second, which is up to 100 times faster than the first-generation time-domain (TD) OCT systems. The system operates at 830 nm. 9 Implications of the increased speed and sensitivity offered by SD OCT are images with lower signal-to-noise ratio compared with TD OCT and high axial resolution. 8 , 9 , 10 , 11 , 12 A wide-angle anterior-segment adaptor (CAM-L mode) for the RTVue-100 OCT (Optovue, Inc., Fremont, CA) provides denser pixel cross-sectional imaging of the cornea and anterior segment with an axial resolution of 5 µm and transverse resolution of 15 µm. 10
A custom-developed SD OCT system, including full distortion correction (fan and optical), as well as segmentation and merging of the different volumes (cornea, iris, and lens), was built to process and generate high-resolution 3-D images. 1 , 2 , 13 , 14 , 15 , 16 , 17 The main features of this device are its 840-nm (50-nm bandwidth) super luminescent diode (SLD) illuminating source; a spectrometer with a 4096-pixel line CMOS (complementary metal-oxide semiconductor) camera; an axial pixel resolution of 3.4 μm; and a predicted axial resolution of 6.9 μm. As a result, 3-D biometrical quantification of the anterior segment can be generated.
Three-dimensional epithelial and total corneal maps can be generated by interpolating thickness profiles from multiple meridians. 18 In addition, SD OCT-based corneal tomography and visualization of all corneal layers by high-resolution cross-sectional scans are essential tools for evaluating refractive candidates, including screening and risk of ectasia assessment, progression of corneal ectasia, corneal collagen crosslinking, intrastromal corneal rings and inlays, custom ablation profiles, and presbyopic treatments.
10.1 Refractive Surgery Screening and Corneal Ectasia Risk Scoring System
One of the most important screening strategies in refractive surgery is to identify risk factors for corneal ectasia and to minimize its occurrence. Several Placido-based computerized video keratoscopy indices have been described for the diagnosis of keratoconus. 19 , 20 , 21 The diagnosis and progression of keratoconus are based on clinical features and anterior corneal curvature data as classically seen with the Amsler-Krumeich classification. 22 Over the last few years, a comprehensive corneal analysis from the anterior and posterior corneal curvature and full pachymetric data has been used for preoperative refractive screening and early detection signs of keratoconus. New corneal tomography parameters using rotating Scheimpflug devices 23 , 24 , 25 and slit-scanning elevation topography 26 have shown changes on both the posterior cornea and/or corneal thickness map as earlier indicators of corneal ectasia.
Spectral-domain OCT measures the anterior and posterior cornea curvature in addition to qualitative and quantitative analysis of the relationship of the corneal epithelium and stroma that can be expressed as topographic thickness variations. The Optovue RTVue-CAM (Optovue, Inc., Fremont, CA) pachymetry-corneal power software (Cpwr), with 1024 axial scans and 6-mm diameter scanning, measures the cornea in eight meridians. The high-resolution, cross-sectional scans are repeated five times and averaged to generate the pachymetry maps. The air-tear interface and the epithelial-Bowman layer landmark were identified automatically by a computer algorithm to generate the epithelial thickness maps, as described by Li et al. 27 The corneal pachymetry map and epithelial thickness map are divided into zones by octants and 2-, 5-, and 6-mm annular rings. The map is obtained in 0.32 seconds and comprises eight meridional scans. The average of 6-mm paracentral pachymetry measurements of superior (S), inferior (I), temporal (T), nasal (N), superotemporal (ST), superonasal (SN), inferotemporal (IT), and inferonasal (IN) zones are displayed. The central measurement corresponds to the average pachymetry of the central 2 mm. The pachymetry map also includes minimum corneal thickness and location, anterior and posterior corneal power and curvature radius, and total corneal power (Fig. 10.1). In previous studies, SD OCT pachymetry maps have shown high reproducibility in detecting eccentric and asymmetric corneal thinning in keratoconus. 27 Recently, an SD OCT pachymetry map–based keratoconus risk scoring system was developed based on these pachymetric variables. 28

Additionally, the cross-sectional high-resolution scans can show localized areas of epithelial compensation overlying areas of stromal thinning in keratoconus (Fig. 10.2). SD OCT high-resolution cross-sectional scans demonstrated significant regional variability in corneal epithelial thickness profiles, as well as greater patterns of thickness deviation in eyes with keratoconus and postoperative corneal ectasia, compared with normal eyes. 28 , 29 , 30 Fig. 10.3 shows two patients with inferior steepening seen on Placido-based corneal topography; SD OCT epithelial thickness map shows localized zone of epithelial thinning surrounded by thickened epithelium over the region of the cone in keratoconus and thickened epithelium, corresponding to the area of inferior steepening seen on topography, in corneal warpage. Analysis of the epithelial thickness profile can be used as an adjunctive tool in refractive screening and possible early detection of keratoconus.


10.2 Epithelial Thickness Remodeling in Refractive Surgery
Recently, detailed analysis of the corneal epithelial thickness profile in laser-assisted in-situ keratomileusis (LASIK) using SD OCT has been described. 31 , 32 The corneal epithelium has a fast turnover, and compensatory changes of the corneal epithelium help to generate a smoother corneal surface in the setting of irregularities of the underlying stroma. 29 , 30 , 31 , 32 , 33 , 34 , 35
We evaluated epithelial thickness remodeling and changes in the flap architecture after myopic LASIK using high-resolution SD OCT to correlate the anatomical findings to the preoperative spherical equivalent manifest refraction and refractive outcomes. 31 Fig. 10.4 shows the air-tear film interface and the epithelial-Bowman layer landmark automatic segmentation and reconstruction, as described by Li et al 36 ; the distance between the anterior surface of the cornea and the flap-stroma interface were manually detected to generate flap-thickness maps (see LASIK Flap Mapping by Spectral-Domain OCT section).

Forty myopic eyes were included in this prospective, randomized, contralateral eye study. The preoperative manifest refraction ranged from –1.00 to –7.25 diopters (mean –3.25 ± 1.9). Flap creation was randomized between eyes by using the IntraLASE FS60 (IL, Abbott Medical Optics, Irvine, CA) in one eye and WaveLight FS200 (FS, Alcon Laboratories Inc., Fort Worth, TX) in the contralateral eye, and all eyes were treated with the ALLEGRETTO Eye-Q excimer laser (Alcon Laboratories Inc.). SD OCT (Optovue RTVue-100, Fremont, CA) was used to evaluate the epithelial and flap thickness profiles and corneal power preoperatively and at 1 week, 1 month, 3 month, and 9 months postoperatively. Statistically significant epithelial thickening was observed in both the IL and FS groups as early as 1 month postoperatively (P = 0.033 and P = 0.042), but this thickening stabilized between 3 (P = 0.042 and P = 0.035) and 9 months postoperatively (P = 0.043 and P = 0.041) (Fig. 10.5). The magnitude of epithelial and flap thickness remodeling correlated to the preoperative spherical equivalent refraction. We found a statistically significant correlation between the magnitude of preoperative myopic refraction and the central epithelial thickness at 1, 3, and 9 months (Pearson correlation coefficients 0.485, 0.587, and 0.576) (P = 0.0021, 0.0010, and 0.0011), respectively. Additionally, the corneal power change reconstructed from the SD OCT maps showed steepening at 3 and 9 months, in correlation with both the thicker epithelium and a mild myopic shift in manifest and wavefront refraction.

The use of SD OCT in refractive surgery is important to recognize the initial instability seen after LASIK and to characterize the spatial relationship of epithelial remodeling with refractive outcomes. We think the pattern of epithelial remodeling with different ablation profiles should be considered in the future planning of customized excimer laser ablations, including topography-guided, wavefront-guided, and multifocal presbyopic excimer laser treatments.
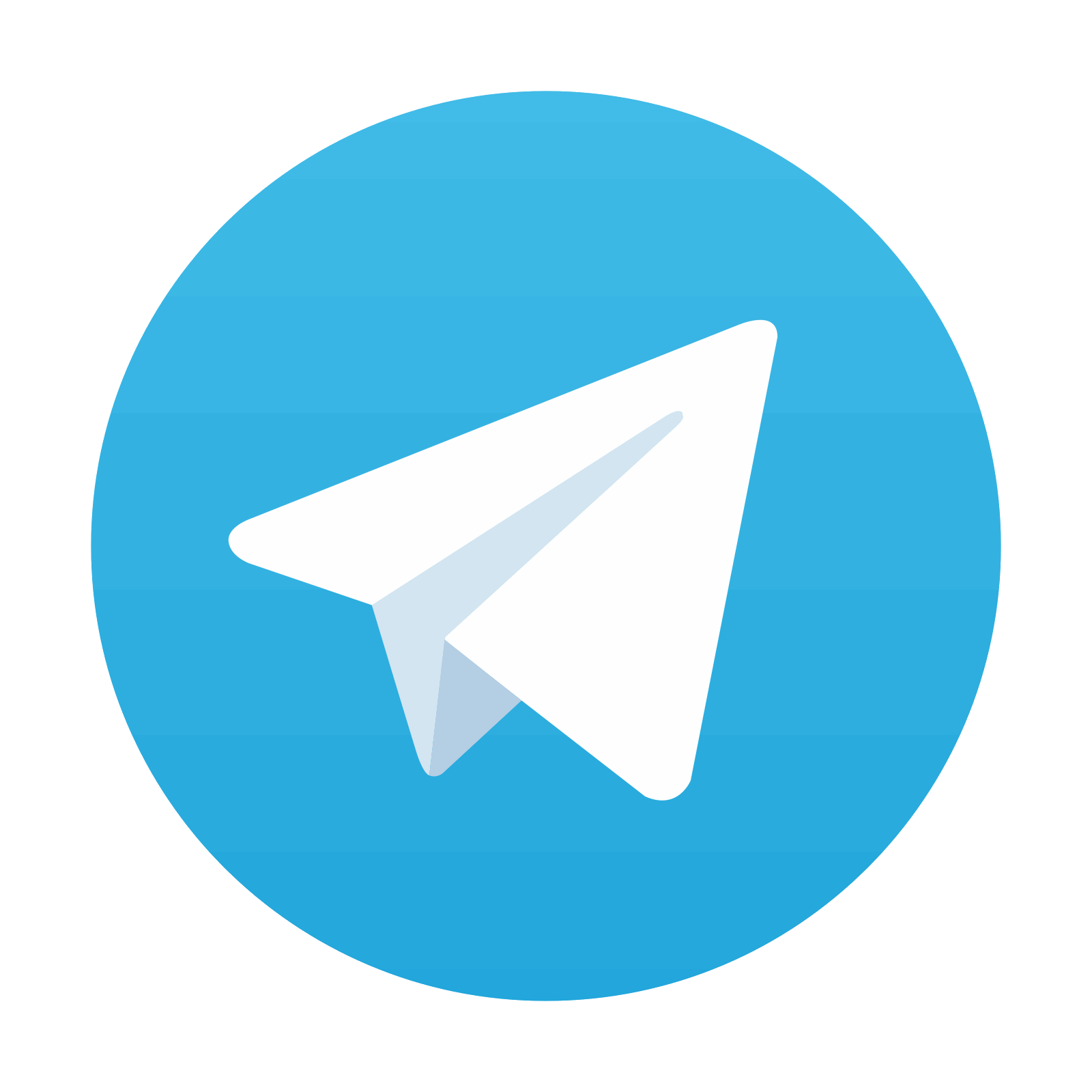
Stay updated, free articles. Join our Telegram channel

Full access? Get Clinical Tree
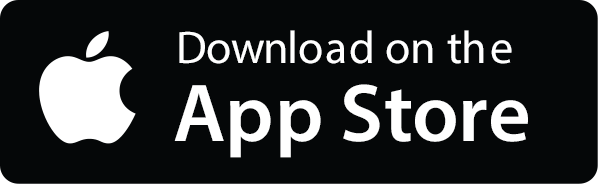
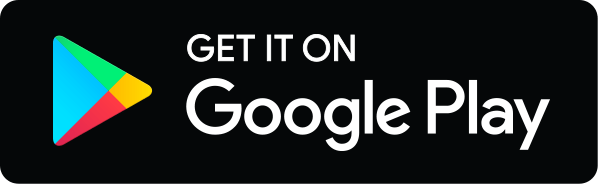