1 Perioperative Care and General Otolaryngology Section Editor Contributors This book is organized into brief chapters addressing specific clinical entities. The chapters generally follow a standard format that allows readers to readily focus on their information needs. The template includes key features; epidemiology; signs and symptoms; differential diagnosis; evaluation, including history, exam, imaging, and other testing; treatment options, including medical and surgical treatments; and follow-up care. Most chapters also include suggestions for further reading. In this first chapter, we review in detail the approach to an efficient and effective otolaryngologic history and physical examination, which should be especially useful to those new to the care of such patients. The generally accepted organization of the history and physical examination for a new patient is outlined in Table 1.1. The History of Present Illness is the subjective narrative regarding the current problem. One should include a focused summary of the complaint, including location, time of onset, quality, severity, duration, associated problems, and previous testing or treatment.
1.0 Approach to the Otolaryngology–Head and Neck Surgery Patient
History
Chief complaint |
History of present illness |
Past medical history |
Past surgical history |
Current medications |
Medication allergies |
Social history |
Family history |
Review of systems |
Physical examination |
Impression |
Plan |
Physical Exam
The physical examination in otolaryngology is typically a complete head and neck exam. This should include an evaluation of the following:
• The general appearance of the patient (i.e., well- or ill-appearing, acute distress)
• Stridor, abnormal respiratory effort
• Cranial nerve function
• The pinnae, ear canals, tympanic membranes, including mobility
• 512-Hz tuning fork testing (Weber, Rinne)
• Description of any cutaneous lesions of the head and neck
• External nasal deformities
• Anterior rhinoscopy noting edema, masses, mucus, purulence, septal deviation, perforation
• Oral cavity and pharynx, noting any masses, mucosal lesions, asymme-tries, condition of dentition
• Mirror exam of the nasopharynx, hypopharynx, and larynx
• Laryngeal exam should note vocal fold mobility, mucosal lesions, and masses as well as assess the base of the tongue, valleculae, epiglottis, vocal folds, and piriform sinuses
• Presence of hoarseness or phonatory abnormality
• Inspection and palpation of the parotid and submandibular glands
• Inspection and palpation of the neck for adenopathy or masses
• Inspection and palpation of the thyroid gland for enlargement or masses
Endoscopic Exam
If the mirror examination does not provide an adequate assessment of the nasopharynx, hypopharynx, or larynx, a flexible fiberoptic nasolaryngoscopy is performed. Usually, the nose is decongested with oxymetazoline (Afrin, Schering-Plough Healthcare Products Inc., Memphis, TN) or Neo-Synephrine (Bayer Consumer Health, Morristown, NJ) spray. Topical pontocaine spray may be added for anesthetic. Surgilube jelly (E. Fougera & Co., Melville, NY) is helpful to reduce irritation. Antifog is applied to the tip of the flexible laryngoscope. The patient is best examined sitting upright. The tip of the scope is inserted into the nostril, and under direct vision is advanced inferiorly along the floor of the nose into the nasopharynx. If there is septal spurring or other intranasal deformities, the other nostril may be used. The nasopharynx is assessed for masses or asymmetry, adenoid hypertrophy, and infection. One can comment of the presence of anteroposterior (AP) or lateral collapse in the sleep apnea patient. The scope is then guided inferiorly to examine the base of tongue, valleculae, epiglottis, piriform sinuses, arytenoids, and vocal folds. Again, mucosal lesions, masses, asymmetries, mobility are noted. Asking the patient to cough and to phonate will reveal vocal fold motion abnormalities.
Other Tests
Often, laboratory studies, audiograms, or imaging studies are reviewed. These are summarized in the note after the physical exam section. Whenever possible, radiology images (CTs, MRIs) should be reviewed to confirm that one agrees with the reports.
Impression and Plan
In the documentation of the patient’s visit, the note concludes with an impression and plan. Generally, a concise differential diagnosis is given, listing the entities that are considered most relevant. A plan is then discussed, including further tests to confirm or exclude possible diagnoses as well as medical or surgical treatments that will be instituted or considered. Timing of a return or follow-up visit, if needed, is noted.
A copy of one’s note, or a separate letter, should always be sent to referring physicians.
1.1 Preoperative Assessment
The aims of the Preoperative Assessment are risk stratification and identification of medical conditions and their severity (Table 1.2). An additional goal is to ensure that medical management is optimized preoperatively. A review of medications, allergies, NPO (nothing by mouth) status (guidelines are summarized in Table 1.3), and previous experiences with anesthesia should be obtained. Laboratory and physiologic tests and screens can be ordered and reviewed prior to surgery. The American Society of Anesthesiologists (ASA) physical status classification (Table 1.4) stratifies patient risk associated with general anesthesia based on physical status and existing diagnoses (it is not differentiated by type of operation or patient age).
System | Assessment |
---|---|
Cardiac | Does the patient have hypertension, congestive heart failure, or coronary artery disease? |
What medications are currently prescribed? | |
Does the cardiac status prohibit the patient from achieving 5 METS (up flight of stairs) without shortness of breath? | |
Review of recent (past-month) ECG for patients older than 45 or those with symptoms? | |
Review of cardiac notes and tests. | |
Respiratory | Does the patient have asthma, COPD, OSA, or reactive airway disease? |
Is the patient on medications, home oxygen, or a CPAP device? | |
Is the patient a smoker? | |
Does the patient have shortness of breath related to pulmonary conditions? | |
Review of PFTs, sleep studies, and pulmonary notes | |
Metabolic | Does patient have diabetes mellitus or thyroid disease? |
Are these conditions optimized? | |
What medications is the patient currently taking? | |
NPO status | Has the patient followed the ASA NPO guidelines?(see also Table 1.3) |
Alcohol/substance abuse | Does the patient have substance abuse issues that will interact with the pharmacology of an anesthetic? |
Will postoperative pain management be complicated by existing opioid abuse? | |
Has alcohol or drug abuse contributed to organ dysfunction? |
Abbreviations: ASA, American Society of Anesthesiologists; CPAP, continuous positive airway pressure; COPD, chronic obstructive pulmonary disease; ECG, electrocardiogram; METS, metabolic equivalents (of oxygen consumption); NPO, nothing by mouth; OSA, obstructive sleep apnea; PFT, pulmonary function test.
Item | Time Frame |
---|---|
Clear liquids | 2 hours |
Infant formula | 6 hours |
Light meal | 6 hours |
Breast milk | 4 hours |
Nonhuman milk | 6 hours |
Medications | Take with <30 mL water > 1 hour prior to surgery |
Abbreviations: NPO, nothing by mouth.
ASA Class | Definition |
---|---|
1 | Patient has no organic, physiologic, biochemical, or psychiatric disturbance. |
| The pathologic process for which the procedure is to be performed is localized and does not entail systemic disturbance. |
2 | Mild to moderate systemic disturbance caused either by the condition to betreated surgically or by other pathophysiologic processes. |
3 | Severe systemic disturbance or disease; variable degree of disability. |
4 | Severe systemic disorders that may be life threatening, not always correctable by operation. |
5 | Seriously ill patient who has little chance of survival, but is submitted to the operation in desperation. |
6 | Patients who have met brain death criteria and are undergoing organ procurement. |
1.2 Airway Assessment and Management
All forms of surgery in the head and neck region require consideration of airway management, maintenance of ventilation with an adequate form of anesthesia, and prevention of concentrated oxygen in the operative field in the presence of cautery or laser. Various forms of airway management are discussed below, considering anatomy, innervation, indications, instrumentation and equipment, and clinical context. The specific situation of the difficult airway is then discussed.
Airway Anatomy
There are two physiologic entry points to the airway: the nose and the mouth. The epiglottis, located at the base of the tongue, separates the oropharynx from the hypopharynx. The larynx is made up of a cartilaginous structure supported by muscles and ligaments.
Innervation
The sensory innervation above the epiglottis is provided by the trigeminal nerve (cranial nerve V [CN V]) and the glossopharyngeal nerve (CN IX); below the epiglottis, by the superior laryngeal and recurrent laryngeal branches of the vagus nerve (CN X). For more information on the cranial nerves, see Appendix B.
• Nasal mucosa: by the sphenopalatine ganglion branch of the middle division of the trigeminal nerve
• Posterior pharynx (including uvula and tonsils): by the continued branches from the sphenopalatine ganglion
• Oropharynx and supraglottic area: by the glossopharyngeal nerve; branches of this nerve include the lingual, pharyngeal, and tonsillar nerves
• Trachea: by the recurrent laryngeal nerve
• Larynx: sensory and motor innervation from the vagus nerve
Sensory: above the vocal folds, innervation is supplied by the internal branch of the superior laryngeal nerve; below the vocal folds, by the recurrent laryngeal nerve.
Motor: all muscles are supplied by the recurrent laryngeal nerve except for the cricothyroid muscle, which is supplied by the external branch of the superior laryngeal nerve.
Airway Equipment
Oral and Nasal Airways
In anesthetized patients, loss of airway tone allows the tongue and epiglottis to contact the posterior pharyngeal tissue leading to obstruction. Artificial airway devices can be placed in the nose or mouth to provide an air passage. Nasal airways carry a risk of epistaxis and should be avoided in anticoagulated patients. These devices should also be avoided in patients with basilar skull fractures to avoid intracranial penetration of the airway device. If an airway device is indicated in a lightly anesthetized patient, the nasal route is generally tolerated better.
Face Masks
The face mask is designed to contour and conform to a variety of facial features with the intention of creating an airtight seal capable of delivering gasses from the anesthesia equipment.
Laryngoscopes
The most commonly used intubating laryngoscopes by the anesthesiology team have curved (Macintosh) or straight (Miller) blades and an open-blade design. Newer fiberoptic video rigid laryngoscopes are useful, such as the GlideScope (Diagnostic Ultrasound Corporation, Bothell, WA). There are a variety of operative laryngoscopes that may be useful for intubation, such as the Holinger or Dedo laryngoscope.
Airway Assessment
A complete airway examination looks at several aspects of the airway. However, the first priority is to identify patients who may be difficult to ventilate or intubate. This is critically important: induction of general anesthesia with the subsequent inability to adequately ventilate the patient is an acute life-threatening emergency. Thus identification of a patient with a potentially difficult airway before induction will enable the operative and anesthetic team to institute an appropriate plan, backup plans, and assemble and test airway equipment ahead of time.
• Mallampati classification: used to predict the ease of intubation by looking at the anatomy of the oral cavity while the patient is sitting upright in the neutral position (Fig. 1.1)
• Thyromental distance: measured from the upper edge of the thyroid cartilage to the chin with head in full extension. A short thyromental distance (<6 cm) equates with an anterior (superior) laryngeal position that is at a more acute angle, which makes the larynx difficult to visualize via direct laryngoscopy. It is a relatively unreliable test unless combined with other tests.
• Mouth opening: less than two finger-widths of mouth opening (trismus) suggest difficulty with intubation.
• Cervical spine movements: mobility of the atlantooccipital and atlantoaxial joints may be assessed by asking the patient to extend the head while the neck is in flexion. Extension of the head with atlantoaxial joint immobility results in greater cervical spine convexity, which pushes the larynx anteriorly and impairs laryngoscopic view.
• Temporomandibular joint (TMJ) mobility: assessed by asking the patient to protrude the jaw while sitting up in the neutral position. Decreased mobility suggests greater difficulty with intubation.
Grade A – lower incisors in front of upper incisors (good mobility)
Grade B – lower incisors up to upper incisors
Grade C – lower incisors cannot be protruded to touch upper incisors (poor mobility)
Fig. 1.1 The Mallampati classification of oral opening. Class 1: visualization of soft palate, hard palate, uvula, and tonsillar pillars. Class 2: visualization of soft palate, hard palate, and portion of uvula. Class 3: visualization of soft palate, hard palate, and base of uvula. Class 4: visualization of hard palate only. (From Goldenberg D, ed. Acute surgical management of the airway. Oper Tech Otolaryngol Head Neck Surg 2007;18(2):73. Reprinted with permission.)
Fig. 1.2 Cormack & Lehane grades. Grade I: visualization of entire laryngeal aperture. Grade II: visualization of posterior part of the laryngeal aperture. Grade III: visualization of epiglottis only. Grade IV: not even the epiglottis is visible. (From Goldenberg D, ed. Acute surgical management of the airway. Oper Tech Otolaryngol Head Neck Surg 2007;18(2):73. Reprinted with permission.)
• Cormack and Lehane grade: used to classify the view on direct laryngoscopy. Previous documentation of laryngoscopy should include the grade of glottic view (Fig. 1.2).
Preoperative Medication
Medications are often administered preoperatively to alleviate anxiety, to provide analgesia, or as aspiration prophylaxis (Table 1.5).
Airway Management
Endotracheal Intubation
Indications for Endotracheal Intubation
• Protection from aspiration
• Altered level of consciousness (Glasgow Coma Score [GCS] score <8)
• Respiratory distress
• Severe pulmonary or multiorgan failure
• Facilitation of positive pressure ventilation
• Operative position other than supine
• Operative site involving the upper airway
Anxiolysis | Analgesia | Aspiration Prophylaxis |
---|---|---|
Diazepam | Narcotics: fentanyl, | Bicitra |
Midazolam | morphine, meperidine | Metoclopramide |
Lorazepam | Nonsteroidal antiinflamma-tory drugs | Famotidine |
| COX II inhibitors |
|
| Acetaminophen |
|
| Gabapentin |
|
• Disease involving the upper airway
• One-lung ventilation
Confirmation of Endotracheal Intubation
• Direct visualization of endotracheal tube passing through the vocal folds
• Carbon dioxide present in exhaled gasses in at least four consecutive breaths (positive end-tidal CO2)
• Bilateral breath sounds
• Absence of air entry during epigastric auscultation
• Condensation of water vapor in endotracheal tube (ETT) during exhalation
• Maintenance of arterial oxygenation
• Chest x-ray with tip of ETT between the carina and thoracic inlet
Endotracheal Tube Size Recommendations
• Endotracheal tube size (mm): Age/4 + 4 for patients >2 years old.
• Length of insertion: 12 + Age/2. Add 2 to 3 cm for nasal intubation.
• Pediatrics: Uncuffed ETTs are generally used in patients <8 years old.
Types of Endotracheal Tube
• Ring-Adair-Elwyn Endotracheal Tubes. Ring-Adair-Elwyn (RAE) endotracheal tubes are preformed to fit in the nose or mouth and are commonly used in oral or pharyngeal surgery, particularly adenoidectomy or tonsillectomy. The shape prevents obstruction of the surgical field and fits surgical retractors such as the Crow–Davis retractor.
• Armored Endotracheal Tubes. These tubes are commonly used in head and neck surgery. They prevent kinking of the tube when the head is manipulated. This tube works well through a tracheotomy as it can be curved inferiorly out of the surgical field without kinking and sutured in place temporarily.
• Laser-Resistant Endotracheal Tubes. Laser-resistant endotracheal tubes are used in laser surgery, particularly treatment of laryngeal lesions. By preventing an interaction of inhaled oxygen and the laser, these tubes help prevent airway fires.
• Nerve-Monitoring Endotracheal Tubes. For thyroid surgery, a tube with contact electromyographic electrodes positioned at the level of the vocal folds permits intraoperative monitoring of recurrent laryngeal nerve integrity.
Endotracheal Intubation Procedure
• Preoperative evaluation will help determine the route (oral vs. nasal) and method (awake vs. anesthetized) for tracheal intubation.
• Equipment: laryngoscope with working light, appropriate-sized endotracheal tubes, oxygen supply, functioning suction catheter, functioning intravenous (IV) line, and anesthetic medications.
• Cricoid pressure: an assistant’s thumb and forefinger depress the cricoid cartilage downward, compressing the esophagus against the underlying vertebral body. This prevents spillage of gastric contents into the pharynx during the period of time from induction of unconsciousness to placement of the endotracheal tube in the trachea.
• Induction of anesthesia can be achieved by using IV or inhaled agents.
Endotracheal Intubation Complications
During intubation, possible complications include aspiration, dental damage, laceration of lips/gingiva/palate, laryngeal injury, esophageal intubation, endobronchial intubation, bronchospasm, and activation of sympathetic nervous system (elevated heart rate [HR] and blood pressure [BP]).
After intubation, possible complications include aspiration, laryngospasm, transient vocal fold incompetence, pharyngitis, or tracheitis.
Orotracheal Intubation (Fig 1.3)
If there is no history of cervical spine instability, the patient’s head is extended into a “sniffing” position. This position aligns the oral, pharyngeal, and laryngeal axes such that the passage from the lips to the glottic opening is a straight line. The height of the operating room (OR) table should be manipulated so that the patient’s head is at the level of the clinician’s xiphoid cartilage.
The laryngoscope is introduced into the right side of the mouth. Advancing the blade posteriorly and toward the midline, the tongue is displaced to the left. Check that the lower lip is not pinched between the blade and the incisors. Placement of blade depends on which style has been selected:
Fig. 1.3 Intubation. (A) Necessary instruments. 1, Mcintosh laryngoscope; 2, stylette; 3, tube with cuff; 4, Guedel tube. (B) Introduction of the tube. (From Becker W, Naumann HH, Pfaltz CR. Ear, Nose, and Throat Diseases: A Pocket Reference. 2nd ed. Stuttgart/New York: Thieme; 1994:450.)
• Miller (straight) blade: the tip of the blade is passed below the laryngeal surface of the epiglottis, which is then lifted to expose the vocal folds.
Regardless of the blade selected, the laryngoscope is lifted upward and forward in the direction of the long axis of the handle. The upper incisors should not be used as a fulcrum for leverage as this may damage teeth.
The vocal folds should be visualized prior to advancement of the endotracheal tube. Passing the endotracheal tube from the right, little resistance should be encountered. The balloon cuff of the endotracheal tube should pass 1 to 2 cm past the vocal folds. Once the proper position of the endotracheal tube is confirmed, it should be secured in place.
Nasotracheal Intubation
Prior to instrumentation, a vasoconstrictor (i.e., oxymetazoline) should be applied to nasal mucosa. After induction of anesthesia and mask ventilation is established the endotracheal tube can be placed.
The naris and endotracheal tube should be generously lubricated. The tube can be softened by placing it in warm water prior to induction. The tube is placed in the nose parallel to the palate, aiming inferiorly to avoid skull base injury, until a loss of resistance is encountered consistent with entrance into the pharynx.
Placement under direct visualization can be performed using a laryngo-scope and McGill forceps to direct the endotracheal tube past the glottic opening. Alternately, a fiberoptic bronchoscope can be placed through the tube and directed past the vocal folds.
Rapid-Sequence Intubation
Indications include aspiration risk (full stomach, history of gastroesophageal reflux disease [GERD], pregnancy, trauma) in a patient who does not appear to be a difficult intubation on physical examination.
Nonparticulate antacids, H2 blockers, and metoclopramide can be administered to decrease the acidity and volume of gastric contents. As with a standard intubation described above, instrumentation should be prepared and available. Preoxygenation with 100% O2 by mask for 3 to 5 minutes or 4 maximal breaths over 30 seconds is sufficient. Once the paralytic and induction agents are administered, no further ventilation is given.
Induction is accomplished with any induction agent, and the procedure is followed immediately with the administration of a paralytic agent. As the medications are being delivered, cricoid pressure should be applied. Succinylcholine (1–1.5 mg/kg) because of its speed of onset is the drug of choice for rapid sequence induction. As soon as jaw relaxation is present, intubation should be performed. Cricoid pressure should continue until tracheal placement of the endotracheal tube is verified.
Conscious Intubation
Indications include history of difficult intubation, acute infectious/inflammatory process that may involve the airway, mandibular fractures or other facial deformities, morbid obesity, and certain neoplasms involving the upper aerodigestive tract.
The indications and plan should be discussed with the patient. As with a standard intubation all necessary equipment should be available and checked prior to induction. A backup plan should be formulated should intubation be difficult, such as the creation of a surgical airway.
Preparing the airway by decreasing secretions with an antisialagogue (glycopyrrolate 0.2 mg 30 minutes before intubation) will help with visualization. If the nasal route is desired, administer 4 drops of 0.25% Neo-Synephrine to each naris to reduce bleeding. After standard monitors are placed, sedation with fentanyl, midazolam, or dexmedetomidine should be considered.
Topical anesthesia can be achieved using topical agents or nerve blocks. Topical agents include lidocaine jelly, nebulized lidocaine, nasal cocaine, or Cetacaine (Cetylite Industries, Pennshauken, NJ) spray. Regional anesthesia, either alone or in combination with topical agents, is useful in awake intubations. Each major nerve should be approached as described above.
Fiberoptic-Assisted Tracheal Intubation
Indications for a fiberoptic-assisted tracheal intubation include upper airway obstruction, mediastinal mass, subglottic edema, congenital upper airway abnormalities, immobile cervical vertebrae, and confirmation of proper double lumen ETT placement.
Nasal Technique
After vasoconstricting and anesthetizing the airway (see above), the ETT is advanced through the naris into the nasopharynx. The bronchoscope is then inserted into the ETT until the epiglottis and glottic opening are visualized. The scope is then passed through the opening until the carina is in view. While maintaining the carina in sight, the ETT is passed over the scope.
Oral Technique
A bite block should be inserted to protect the fiberoptic scope after anesthetizing the airway (see above). The tracheal tube is inserted into the oropharynx (8–10 cm) and the bronchoscope is inserted through the ETT. The epiglottis and glottic opening should be in view. The ETT is advanced over the scope as the carina is kept in view.
Transtracheal Ventilation
Transtracheal ventilation serves as a temporizing measure if mask ventilation and oxygenation become inadequate or impossible. A catheter (12- or 14-gauge) is inserted into the trachea through the cricothyroid membrane and connected to a jet-type ventilator capable of delivering gas at a pressure of 50 psi. The gas is then delivered using the hand-held jet ventilator. Ventilation is best assessed by observing chest rise and fall. It is advised that an inspiration:expiration (I:E) ratio of 1:4 is utilized.
Laryngeal Mask Airway (LMA)
Indications for the LMA
• Can be used in place of a face mask or ETT
• Can be used in place of an ETT for controlled ventilation as long as the peak pressure does not exceed 30 cm H2 O
• Can aid in the management of difficult airways by providing ventilation or as a guide for fiberoptic intubation
Contraindications for the LMA
• Does not protect against gastric regurgitation and aspiration because it does not provide an airtight seal of the airway
Insertion of the LMA
• The backside of the LMA should be lubricated with a water-soluble lubricant.
• Unconsciousness is rendered with an induction agent of choice. Typically, propofol at doses of 2 to 3 mg/kg produces reliable jaw and pharyngeal muscle relaxation.
• With the LMA held like a pencil, it is placed into the midline of the mouth and advanced with pressure against the hard palate as it slides into the hypopharynx. When the upper esophageal sphincter is encountered resistance is felt. The LMA is then inflated.
• When placed properly, the black vertical line on the back of the LMA faces backward toward the head of the patient.
• The LMA is then removed upon emergence when the patient can follow commands.
Complications of the LMA
• Possible regurgitation/aspiration
• Negative pressure pulmonary edema can result when placed improperly in a spontaneously breathing patient.
• LMA malfunction in patients with pharyngeal or esophageal disease.
• Placement requires neck extension, which is often contraindicated with cervical spine disease.
The GlideScope
The GlideScope is a video laryngoscope that can be a useful alternative to the fiberoptic scope for placement of an endotracheal tube if a difficult airway is expected. The blade is curved like the Macintosh blade with a 60-degree curvature to match the anatomic alignment. The GlideScope has a digital camera incorporated in the blade, which displays a view of the vocal folds on a monitor. Under visualization on the monitor, an endotracheal tube is passed between the vocal folds.
Surgical Laryngoscopes
Closed cylinder-style rigid laryngoscopes with bright fiberoptic light guides such as the Dedo or Holinger are used by the otolaryngologist and have advantages that permit visualization of the glottis and intubation.
The Difficult Airway
Among otolaryngology–head and neck surgery patients, a high percentage presents with a difficult airway (Table 1.6). By definition, a patient with a difficult airway potentially poses difficulty with ventilation or endotracheal tube placement. The goal of history, physical exam, and chart review is to identify patients with difficult airway before they arrive in the OR. Evaluation by the otolaryngologist and review of diagnostic studies can provide invaluable information to the anesthesiologist when a difficult airway is suspected. Conditions associated with difficult airway are discussed below.
Condition | Examples |
---|---|
Tumors | Cystic hygroma |
Hemangiomas | |
Hematoma | |
Infections | Submandibular abscess |
Peritonsillar abscess | |
Epiglottitis | |
Congenital anomalies | Pierre Robin syndrome |
Treacher Collins syndrome | |
Laryngeal atresia | |
Goldenhar syndrome | |
Craniofacial dystocias | |
Trauma | Laryngeal fracture |
Mandibular/maxillary fracture | |
Inhalational burn | |
Cervical spine injury | |
Inadequate neck extension | Rheumatoid arthritis |
Ankylosing spondylitis | |
Halo traction | |
Anatomic variations | Micrognathia |
Prognathism | |
Large tongue | |
Arched palate | |
Short neck | |
Prominent upper incisors | |
Obesity |
|
Airway foreign body |
|
The induction of anesthesia in otolaryngology patients should not be initiated until a plan is formulated between the surgical and anesthesia teams. As outlined in the Difficult Airway Algorithm of the American Society of Anesthesiologists (Fig. 1.4), the plan should address basic management choices: surgical versus nonsurgical approach, awake versus asleep, and spontaneous versus nonspontaneous ventilation. The discussion should address backup plans should the initial attempt to secure the airway fails or inability to ventilate ensues. A surgical airway is indicated when ventilation becomes inadequate despite utilization of a nonsurgical airway ventilation device [jet ventilation, LMA, Combitube (Tyco-Kendall, Mansfield, MA)] in a patient under general anesthesia.
If a difficult airway is suspected, instrumentation and plans for possible surgical airway should be available. Cricothyroidotomy is the preferred method in adults. Briefly, the neck is extended, and the cricothyroid membrane is palpated and incised with a scalpel. The airway is entered and an endotracheal or tracheotomy tube is placed to ventilate the patient. Details on cricothyroidotomy are below.
Clinical situations involving a patient with a difficult airway can be divided into two categories. The first is the acute or urgent problem and the second, an elective situation with a suspected or known difficult airway. Both of these scenarios are to be handled differently. A logical, strict approach can prevent a disastrous outcome in what is often a stressful situation. The otolaryngologist–head and neck surgeon should have particular expertise in ensuring an adequate airway and should be skilled in the use of laryngos-copy, bronchoscopy, and surgical approaches to the airway.
Specific techniques to be discussed here include cricothyroidotomy and awake tracheotomy.
Cricothyroidotomy
For a patient with impending or complete obstruction, rapid establishment of an airway is required—the OR is the safest place for this airway control though this is not always possible. If the situation requires establishment of an emergency surgical airway, a cricothyroidotomy is generally the preferred procedure (Fig. 1.5). This is because it is simpler and faster than a tracheotomy and has a lower complication rate, especially in less-experienced hands. If it is impossible to mask ventilate, intubate, or control the airway by any other technique such as a laryngeal mask or jet ventilation, or if these are not readily available, cricothyroidotomy should be performed by the most trained and skilled physician in attendance.
Contraindications to cricothyroidotomy include subglottic stenosis or mass, laryngeal trauma, with an inability to identify landmarks; in general, it should be avoided in the pediatric population. A cricothyroidotomy should be revised to a tracheotomy in the OR within 24 hours to prevent subsequent development of subglottic stenosis.
Fig. 1.4 The American Society of Anesthesiologists Difficult Airway Algorithm. (From Practice guidelines for management of the difficult airway: an updated report by the American Society of Anesthesiologists Task Force on Management of the Difficult Airway. Anesthesiology 2003;98:1273. Reprinted with permission.)
Fig. 1.5 Cricothyroidotomy. The airway is entered through an incision of the cricothyroid membrane. In this illustration, a trocar through a tracheotomy tube is used; a no. 15 blade will also suffice. (From Probst R, Grevers G, Iro H. Basic Otorhinolaryngology: A Step-by-Step Learning Guide. Stuttgart/New York: Thieme; 2006:379.)
For needle cricothyroidotomy, a catheter is placed over a needle that penetrates the cricothyroid membrane, allowing ventilation by a pressurized stream of oxygen. Needle cricothyroidotomy is the preferred method of establishing an emergency airway in children younger than 10 to 12 years. (See the Cricothyroidotomy section, A7, in Appendix A).
Stable but Compromised Airway
A patient with supraglottitis, angioedema, or a laryngeal mass may have a stable but compromised airway. These patients maintain their oxygen saturation and do not require an emergency airway; however, the stability of the situation requires clinical judgment. In some cases, the patient can be managed with medical management and close monitoring. In other cases, in may be prudent to secure the airway.
Tracheotomy is a surgical procedure in which the tracheocutaneous airway is created through the anterior neck and the trachea. It may be reversible (Table 1.7). (For details on open surgical tracheotomy, see A6 in Appendix A.)
Awake Tracheotomy
In the unstable, poorly ventilated patient, an airway must be secured. If intubation is deemed impractical or dangerous, an awake tracheotomy can be performed. It is important to note that this is best done in a controlled environment, in the OR, or in the intensive care unit (ICU) with local anesthetic and a patient who can maintain spontaneous adequate ventilation. Clear communication between the otolaryngologist–head and neck surgeon, anesthetist, nurses, and technicians is vital. It is important to note that a life-threatening emergency may arise and this will necessitate close collaboration. All equipment should be open and ready.
Upper airway obstruction with any of the signs or symptoms at right | Air hunger, stridor, retractions, obstructive sleep apnea, bilateral vocal fold paralysis, previous neck surgery or throat trauma, previous neck irradiation, prolonged or expected prolonged intubation |
Pulmonary toilet | Inability of patient to manage secretions, including the following: aspirations and excessive bronchopulmonary secretions |
Ventilatory | Where prolonged ventilation or ventilatory |
support | problems are anticipated |
For the awake tracheotomy, the patient should be placed in the semi-Fowler position enabling the anesthesiologist ready access to the airway. Every effort should be made to keep the patient comfortable and the general atmosphere should be as calm as possible. The patient is typically only minimally sedated and should not be paralyzed, maintaining spontaneous adequate ventilation.
Note that in the elective (nonurgent) tracheotomy the patient is intubated prior to the start of surgery.
• Awake Tracheotomy Technique. Surgical landmarks are palpated, and a horizontal or vertical skin incision is made between the cricoid and suprasternal notch. Subcutaneous tissue is bluntly spread. Next, lateral retraction of the strap musculature is performed. The thyroid isthmus is encountered and either retracted caudad, cephalad, or divided using a monopolar cautery. Once the trachea is in view, a tracheal hook may be used to provide superior retraction on the cricoid ring; next the trachea is entered, usually below the second ring, and a window or inferiorly based (Bjork) flap is created.
An appropriate tracheotomy tube is then placed while the anesthesiolo-gist withdraws the endotracheal tube under direct visualization. After the tracheotomy tube is in place, the cuff is inflated, the patient is ventilated and CO2 return and appropriate rise in oxygenation are confirmed. Then, the tracheotomy tube is sutured at four corners with suture, and a tracheotomy tie should be placed to secure the tracheotomy tube around the neck.
Complications of tracheotomy include bleeding, infection, crusting or mucus plugging, pneumothorax, and accidental dislodgment of the tracheotomy tube. Late postoperative complications include tracheoesophageal fistula, tracheal stenosis, tracheocutaneous fistula, and airway stenosis. A rare but deadly complication is a trachea–innominate artery fistula.
Percutaneous Dilation Tracheotomy
A percutaneous dilation tracheotomy is not to be used in an emergent airway situation: this procedure is only to be performed on patients who are already intubated. Percutaneous bedside dilation tracheotomy has become a safe alternative to elective open tracheotomy in many ICUs. There are several different techniques all widely based on the Seldinger technique (needle and catheter over guide wire). Indications for a percutaneous dilation tracheotomy (PDT) are identical to those used for a routine open operative tracheotomy. The most common indication is prolonged intubation, mechanical ventilation. However, particular attention should be paid to potential contraindications. Some absolute contraindications for the performance of a PDT are the need for emergency tracheotomy (i.e., an emergent airway), or in a population of infants and children. Relative contraindications include poor neck landmarks, morbid obesity, previous neck surgery, limited neck extension, and severe coagulopathy that is not correctable. Other relative anesthetic contraindications include high positive end expiration pressure (PEEP >18 cm), high airway pressure (>45 cm), high FiO2 requirement (>80%), retrognathic mandible with limited view of the larynx, and an inability to reintubate.
An appropriate percutaneous tracheotomy kit (i.e., Ciaglia or Griggs kit) and a video bronchoscope are needed to perform this procedure. At least two persons are needed: one to perform the tracheotomy, the other to manage the endotracheal tube and video bronchoscope.
• Percutaneous Dilation Tracheotomy Technique. A shoulder roll is placed beneath the patient’s shoulders and a bronchoscopist should perform simultaneous bronchoscopy through the endotracheal tube during the procedure. An incision is made either horizontally or vertically between the cricoid and the suprasternal notch and an introducer needle is placed into the trachea at about the second ring; this is confirmed with bubbling of air and with simultaneous video bronchoscopy. In addition, transillumination of light from the bronchoscope can be used to help demarcate the best site for the introducer needle. The needle is withdrawn and the overlying plastic cannula is left in place. A J-tipped guide wire is then placed under direct visualization through the catheter into the trachea. Again, every step should be bronchoscopically visualized on the video monitor during the procedure. Dilation is then performed using the provided dilator over the guide wire. This is removed and once dilation of the anterior tracheal wall has been performed, a percutaneous tracheotomy appliance is placed over the guide wire and positioned in the airway. Following this, the bronchoscope should be withdrawn from the endotracheal tube and introduced via the tracheotomy tube to confirm placement in the airway. The placement is confirmed by visualization of the carina. The tracheotomy tube is connected to the anesthesia circuit to confirm bilateral breath sounds and end-tidal CO2. It is then secured using four sutures on all corners of the flange and a tracheotomy tie is also used to secure the appliance around the neck. Only then can the orotracheal tube be fully removed.
Criteria for Extubation
• Respiratory rate (RR)<30 per minute
• Tidal volume (TV) >5 mL/kg
• Vital capacity (VC) >10 mL/kg
• Negative inspiratory force (NIF) > −20 cm H2 O
• Tidal volume/respiratory rate (TV/RR) >10
• PaO2 >70 mm Hg (40% fraction of inspired oxygen [FiO2 ])
• PaCO2 <50 mm Hg
• Level of consciousness (LOC) stable or improving
• Ventilation, dead space/tidal volume ratio (Vd/Vt) <0.6
Further Reading
Caplan RA, Benumof JL, Berry FA, et al. Practice guidelines for management of the difficult airway. A report by the American Society of Anesthesiologists Task Force on Management of the Difficult Airway. Anesthesiology 1993;78(3):597–602
Fowler RA, Adhikari NKJ, Scales DC, Lee WL, Rubenfeld GD. Update in critical care 2008. Am J Respir Crit Care Med 2009;179(9):743–758
Goldenberg D, ed. Acute surgical management of the airway. Oper Tech Otolaryngol Head Neck Surg 2007;18(2):71–160
Hsiao J, Pacheco-Fowler V. Videos in clinical medicine: cricothyroidotomy. N Engl J Med 2008;358(22):e25
1.3 Diagnostic Imaging of the Head and Neck
Many of the structures of the head and neck are deep and inaccessible to direct visualization, palpation, or inspection. Therefore, valuable information may be obtained by the use of various radiographic techniques. Technologic advances have replaced simple x-ray procedures with computed tomography (CT), magnetic resonance imaging (MRI), ultrasound, and positron emission tomography (PET). Other imaging modalities, such as angiography, are used for specific conditions such as vascular lesions.
Computed Tomography
A contrast-enhanced CT scan is typically the first imaging technique used to evaluate many ear, nose, throat, and head and neck pathologies. The CT scan is an excellent method for staging of tumors and identifying lymphadenopathy. A high-resolution CT scan may be used in cases of trauma of the head, neck, laryngeal structures, facial bones, and temporal bone. Temporal bone CT is used to assess middle ear and mastoid disease; paranasal sinus CT is the gold standard test for assessing for the presence and extent of rhinosinusitis. A CT scan is superior to MRI in evaluating bony cortex erosion from tumor. A CT scan is also widely used for posttreatment surveillance of head and neck cancer patients.
Working Principle of CT
In CT, the x-ray tube continuously rotates around the cranio-caudal axis of the patient. A beam of x-rays passes through the body and hits a ring of detectors. The incoming radiation is continuously registered and the signal is digitized and fed into a data matrix taking into account the varying beam angulations. The data matrix can then be transformed into an output image (Fig. 1.6).
Recent advances have improved the quality of CT imaging. Multidetector scanners have several rows of photoreceptors, allowing the simultaneous acquisition of several slices. Helical techniques allow patients to move continuously through the scanner instead of stopping for each slice. These advances have significantly decreased scan times and radiation exposure while improving spatial resolution. Newer in-office flat plate cone beam scanners can rapidly acquire 1-mm slice thickness images of the sinus and temporal bone with very low radiation exposure.
Fig. 1.6 Working principle of computed tomography. The x-ray tube rotates continuously around the longitudinal axis of the patient. A rotating curved detector field opposite to the tube registers the attenuated fan beam after it has passed through the patient. Taking into account the tube position at each time point of measurement, the resulting attenuation values are fed into a data matrix and further computed to create an image. (From Eastman GW, Wald C, Crossin J. Getting Started in Clinical Radiology: From Image to Diagnosis. Stuttgart/New York: Thieme; 2006:9. Reprinted with permission.)
Body Component | Hounsfield Units (HU) |
---|---|
Bone | 1000–2000 |
Thrombus | 60–100 |
Liver | 50–70 |
Spleen | 40–50 |
Kidney | 25–45 |
White brain matter | 20–35 |
Gray brain matter | 35–45 |
Water | −5 to 5 |
Fat | 100 to −25 |
Lung | −1000 to −400 |
Source: Eastman GW, Wald C, Crossin J. Getting Started in Clinical Radiology: From Image to Diagnosis. Stuttgart/New York: Thieme; 2006:9. Reprinted with permission.
Contrast Media
Contrast media is used in CT to visualize vessels and the vascularization of different organ systems (Table 1.8). Contrast material contains iodine or barium, which attenuates the scan radiation.
Computer-Assisted Surgical Navigation
CT scanning data can be utilized for computer-assisted surgical navigation. There are several systems in use. The axial CT image data, acquired at 1 mm slice thickness or less, is loaded onto the image-guidance system in the operating room. The machine uses a system, such as an infrared camera, to detect the patient’s facial features or landmarks, and can also be registered to detect various surgical instruments. The location of an instrument tip is then displayed on the previous CT images in three planes. Most often, this is used in sinus surgery.
Magnetic Resonance Imaging
MRI provides the physician with high definition imaging of soft tissue without exposing the patient to ionizing radiation. MRI is useful for mucosal tumors, neoplastic invasion of bone marrow, and at times perineural invasion of large nerves. MRI is valuable in assessing intra cranial extension of tumors of the head and neck. Gadolinium-enhanced MRI of the brain with attention to the internal auditory canal is the gold standard test for diagnosis of vestibular schwannoma or meningioma, easily identifiable on postcontrast T1-weighted images. The disadvantages of MRI include limited definition of bony detail, and cost. Magnetic resonance angiography (MRA) is a useful modality for imaging vascular anatomy or vascular pathology without the intravascular infusion of iodine contrast medium, which is used in traditional angiography with x-ray fluoroscopy.
Time to repetition | TR |
Time to echo | TE |
Time to inversion | Ti |
Time to magnetize (regrowth); also known as spin lattice relaxation time | T1 |
Time to demagnetize (DK); also known as spin relaxation time | T2 |
Working Principle of MRI
MRI is a technique that produces cross-sectional images in any plane without the use of ionizing radiation. MR images are obtained by the interaction of hydrogen nuclei (protons), high magnetic fields, and radiofrequency pulses. This is done by placing the patient in a strong magnetic field, which initially aligns the hydrogen nuclei in similar directions. The intensity of the MRI signal that is converted to imaging data depends on the density of the hydrogen nuclei in the examined tissue (i.e., mucosa, fat, bone) and on two magnetic relaxation times (Table 1.9).
Contraindications to MRI
• Implanted neural stimulators, cochlear implants, and cardiac pacemakers (MRI may cause temporary or permanent malfunction)
• Ferromagnetic aneurysm clips, foreign bodies with a large component of iron or cobalt that may move or heat up in the MRI scanner
• Metallic fragments within the eye
• Placement of a vascular stent, coil, or filter in the past 6 weeks
• Ferromagnetic shrapnel
• Relative contraindications include claustrophobic patients, critically ill patients, morbidly obese patients who cannot physically fit in the MRI scanner, metal implants in the region of interest
Ultrasound
Ultrasound or ultrasonography is an inexpensive and safe method of gaining real-time images of structures of the head and neck. Neck masses can be assessed for size, morphologic character (i.e., solid, cystic, or combined solid and cystic also known as complex), and for association with adjacent structures. High-resolution ultrasound is used for head and neck anomalies such as thyroglossal duct cysts, branchial cleft cyst, cystic hygromas, salivary gland masses, abscesses, carotid body and vascular tumors, and thyroid masses.
Working Principle of Ultrasound
An alternating electric current is sent through piezoelectric crystals; it vibrates with the frequency of the current, producing sound waves of that frequency. In medical ultrasound, typical frequencies vary between 1 and 15 MHz. Ultra-sound gel acoustically couples the ultrasound transducer to the body, where the ultrasound waves can then spread. Inside the body the sound is absorbed, scattered, or reflected. Fluid-filled (cystic) structures appear dark and show acoustic enhancement behind them. Bone and air appear bright because they absorb and reflect the sound, showing an “acoustic shadow” behind them (Fig. 1.7). Linear transducers with a width of 7.5 to 9 cm and frequencies of 10 to 13 MHz are typically used for evaluating the neck and thyroid.
Fig. 1.7 Working principle of ultrasonography. (From Eastman GW, Wald C, Crossin J. Getting Started in Clinical Radiology: From Image to Diagnosis. Stuttgart/New York: Thieme; 2006:11. Reprinted with permission.)
Barium Esophagram
An esophagram (also known as a barium swallow) is designed to evaluate the pharyngeal and esophageal mucosa; it is distinct from a modified barium swallow (MBS), which evaluates laryngotracheal aspiration and is usually performed in conjunction with a speech pathologist. These techniques are performed utilizing fluoroscopy. Fluoroscopy with intraluminal contrast is invaluable for studying the functional dynamics of the pharynx and esophagus.
An MBS evaluates the coordination of the swallow reflex. It is most often used to determine the etiology and severity of airway aspiration. A speech pathologist is usually in attendance and administers barium suspensions of varying thickness (thin liquid, thick liquid, nectar, paste, and solid) while the radiologist observes fluoroscopically in the lateral projection. One can also assess for esophageal motility/dysmotility, Zenker diverticulum, stricture, mass, hiatal hernia, or obvious free reflux.
Contrast Media
Barium suspension is the most commonly used fluoroscopic contrast agent. If a perforation of the hypopharynx or esophagus is suspected there is a risk for barium extravasation into the soft tissues of the neck and/or chest. Therefore, in these cases, water-soluble contrast agents are used (such as Gastrografin, Bracco Diagnostics, Inc., Princeton, NJ). It is important to note that these agents may cause a chemical pneumonitis or severe pulmonary edema if aspirated into the airway.
Nuclear Medicine Imaging
Positron Emission Tomography with Computed Tomography
PET-CT is essentially a positron emission tomography scan performed and superimposed upon a simultaneous computed tomography scan to allow precise correlation between increased function (enhanced cellular activity) and anatomic evaluation provided by the CT. The PET scanning portion is a functional imaging technique that measures metabolic activity through the use of tagged nuclear isotopes such as a glucose precursor. 18F-fluoro-deoxyglucose (FDG) is the most commonly used radiotracer and has a half-life of ~110 minutes.
Working Principle of PET-CT
After being emitted from the atom, the positron travels in the tissue for a short distance until it encounters an electron and forms a positronium, which immediately annihilates (converts its mass to energy) forming two photons. These annihilation photons travel away from each other at 180 degrees and are picked up by the detectors placed around the patient. Simultaneous detection of these photons relates them to the same annihilation event and allows spatial localization. Annihilation detection is accomplished by dedicated PET scanners, which yield spatial resolution and sensitivity. The spatial resolution of the final reconstructed images is limited by the number of collected events.
18F is cyclotron produced and has a half-life of 110 minutes. Tumor concentration of FDG generally peaks at 30 minutes, remains constant for 60 minutes, and then declines.
Note that FDG can also accumulate nonspecifically in other cells that have active glycolysis such as areas of active inflammation and infection. This may lead to a false-positive PET-CT scan. Other activities that may cause false-positive findings include muscular activity, foreign bodies, and granulomas. False-negatives in PET-CT scans may occur when the tumor threshold is too small (<0.5 cm in diameter). In PET scanning, quantification of FDG uptake intensity is generally expressed on an arbitrary scale as standard uptake values (SUVs).
Thyroid Scintigraphy
Thyroid scintigraphy renders, at one point in time, information about the global and regional functional status of the thyroid. It is observer-independent and reproducible with low inherent radiation exposure. Scintigraphic imaging of the thyroid helps determine whether solitary or multiple nodules are functional when compared with the surrounding thyroid tissue. Findings for a nodule may be normal functional (warm), hyperfunctional (hot), or hypofunctional (cold). Scintigraphy can also help determine whether cervical masses contain thyroid tissue, and it can demonstrate whether metastases from well-differentiated thyroid cancer concentrate iodine for the purpose of radioiodine therapy. For thyroid scintigraphy the following radionuclides are in use: technetium-99m (99mTc), ioflupane (123 I), and iodine-131 (131I).
Working Principle of Thyroid Scintigraphy
The technique of thyroid scintigraphy is based on the principle that functional active thyroid cells incorporate iodine.
Parathyroid Scintigraphy
Several radiotracers are available for parathyroid scintigraphy. At present, the radiotracer of choice is 99mTc-sestamibi (aka sestamibi, methoxyisobutylisonitrile, or MIBI). 99mTc-sestamibi is a lipophilic cation that is taken up in the mitochondria of the cells. Of note, this radiotracer can be used with a wide variety of imaging techniques including planar MIBI, single proton emission computed tomography (SPECT), and fused SPECT-CT.
Working Principle of Parathyroid Scintigraphy
Sestamibi accumulates in the thyroid and parathyroid tissues within minutes after IV administration, but it has a different washout rate from these two tissues. It is released faster from the thyroid than from the parathyroid. The presence of large numbers of mitochondria-rich cells in parathyroid adenomas is thought to be responsible for their slower release of 99mTc-sestamibi from hyperfunctioning parathyroid tissue versus the adjacent thyroid tissue. Thus, on 2- to 3-hour washout images, after thyroid uptake has dissipated, the presence of a retained area of activity allows one to identify and localize a parathyroid adenoma. Overall, 99mTc-sestamibi parathyroid scintigraphy has good sensitivity for the detection and localization of a single adenoma in patients with primary hyperparathyroidism. Correlation with ultrasound findings can also be helpful.
Single Proton Emission Computed Tomography
SPECT scanning for parathyroid disease allows increased accuracy of routine sestamibi scanning by ~2 to 3%. SPECT scanning can be performed within the first several hours after a patient is injected with the sestamibi. During the scan, multiple images are taken of the patient’s head and neck. These images are assimilated to provide a three-dimensional picture. SPECT scanning is typically used when ordinary planar sestamibi scans are inconclusive.
Further Reading
Eastman GW, Wald C, Crossin J. Getting Started in Clinical Radiology: From Image to Diagnosis. Stuttgart/New York: Thieme; 2006
Mafee MF, Valvassori GE, Becker M. Imaging of the Head and Neck. 2nd ed. Stuttgart/New York: Thieme; 2005
Moedder U, Cohnen M, Andersen K, Engelbrecht V, Fritz B. Head and Neck: Direct Diagnosis in Radiology. Stuttgart/New York: Thieme; 2008
1.4 Anesthesia
1.4.1 Principles of Anesthesia
Modes of Anesthesia
• General anesthesia: characterized by total loss of consciousness with blunted (or absent) protective upper airway reflexes and the use of an endotracheal tube or supraglottic device (e.g., laryngeal mask airway).
• Sedation: aims to maintain protective upper airway reflexes in patients with iatrogenic altered levels of consciousness. Supplemental oxygen is often required (e.g., nasal cannula), with or without the assistance of an oral/nasopharyngeal airway. It can range from minimal (i.e., patient is responsive to verbal command) to deep (i.e., no response to procedural stimulation).
• Regional anesthesia: involves the use of local anesthetics in conjunction with sedation or general anesthesia to improve analgesia, expedite recovery, and reduce overall IV/inhalational anesthetic requirements. It can be used as a sole anesthetic for minor or superficial procedures.
Factors of Anesthesia
An ideal anesthetic strikes a balance between the following four essential factors, which in turn are influenced by independent patient risk factors, unique surgical requirements, and circumstances under which recovery is to occur.
• Amnesia/anxiolysis: management of preoperative anxiety and control of intraoperative awareness is a cornerstone of anesthetic care. Various oral, IV, and/or inhalational agents may be used, depending on the desired effect.
• Analgesia: multimodal therapy is the preferred method of achieving intra- and postoperative pain control with a combination of narcotics, nonsteroidal antiinflammatory drugs (NSAIDs), local anesthetics, and/or adjutants [e.g., gabapentin, Ketamine (Pfizer Pharmaceuticals, New York, NY), clonidine].
• Muscle relaxation: depolarizing and/or nondepolarizing muscle relaxants facilitate optimal airway conditions for laryngoscopy and surgical manipulation.
• Antiemetics: postoperative nausea and vomiting (PONV) is a strongly undesirable complication in patients following head and neck surgery. High-risk procedures include otology surgery, adenotonsillectomy, thyroidectomy, as well as head and neck cancer surgery. PONV influences patient satisfaction, length of stay, recovery, and surgical outcome. Multimodal and prophylactic therapy improves outcomes. Emesis may predispose development of wound hematoma, which can become an airway emergency in the presence of a neck wound.
Phases of Anesthesia
• Preinduction: begins in the preoperative area and continues to the point where a patient is positioned on the operating room table with standard monitors (electrocardiogram [ECG], noninvasive blood pressure, pulse oximetry, capnography) and has been adequately preoxygenated.
• Induction: general anesthesia in patients with established IV access is initiated with rapid-acting parenteral drugs to facilitate airway control. Otherwise, inhalational anesthetics are administered via a face-mask; once an adequate depth of anesthesia is achieved, IV access is established and is followed by the placement of an endotracheal tube or supraglottic device (unless a very brief procedure is planned). For sedation cases, IV agents are titrated to effect while maintaining spontaneous ventilation. For difficult airway situations, awake fiberoptic nasotracheal intubation or awake tracheotomy may be indicated to secure the airway, prior to induction of general anesthesia.
• Maintenance: global amnesia is maintained during surgery with inhalational agents and/or parenteral drug infusions. Surgical analgesia is maintained either with a well-established regional block, generous local anesthetic infiltration, intermittent opioid boluses, and/or IV narcotic infusion. Duration of required muscle paralysis depends on several factors, and may be just long enough to facilitate endotracheal tube placement. In some instances (e.g., cases involving extensive neuromonitoring), anesthesia may be maintained through the predominant or exclusive use of parenteral infusions (total intravenous anesthesia [TIVA]). TIVA is sometimes useful to reduce vasodilation and bleeding, i.e., in endoscopic sinus surgery.
• Emergence: extubation upon meeting criteria
Table 1.10 provides an overview of the key aspects of each phase of anesthesia.
Stages of Anesthesia
• Stage 1: the period between initial delivery of induction agents and loss of consciousness. Patients experience analgesia without amnesia and can often carry on a conversation during this time.
• Stage 2: more commonly known as the “excitement stage,” follows loss of consciousness and is characterized by delirious, uninhibited, and often spastic activity. Cardiovascular and respiratory patterns are erratic. There is also an increased risk for aspiration. The goal of any anesthetic induction is thus to minimize time spent in this stage.
• Stage 4: massive anesthetic overdose that causes severe depression of brainstem activity leading to respiratory and/or cardiovascular collapse. This stage should never be reached as it may be lethal even with appropriate cardiovascular and/or respiratory support.
Further Reading
Barash PG, Cullen BF, Stoelting RK, et al. Clinical Anesthesia. 6th ed. Philadelphia, PA: Lippincott Williams & Wilkins; 2009
Morgan GE, Mikhail MS, Murray MJ. Clinical Anesthesiology. 4th ed. New York:McGraw-Hill Medical; 2005
1.4.2 Regional Anesthesia Techniques
Benefits of Regional Anesthesia
When combined with a general anesthetic, regional nerve blocks reduce narcotic requirements and their subsequent side effects such as nausea, vomiting, somnolence, and respiratory depression. Time to discharge is reduced.
Contraindications to Regional Anesthesia
Contraindications to regional anesthesia include lack of patient consent and interference with surgical field/technique. Relative contraindications include coagulopathy, infection at the skin site, and presence of neurologic disease.
Complications Common to All Nerve Blocks
Complications common to all nerve blocks include local anesthetic complications (intravascular injection, overdose, and allergic reaction), nerve damage (needle trauma, intraneural injection), and hematoma.
Blocks of the Scalp and Face
Supraorbital and Supratrochlear Nerve
Indications: closure of lacerations, forehead, and ear procedures
The forehead and anterior scalp can be rendered insensate by blocking the supraorbital and supratrochlear branches of the ophthalmic division of the trigeminal nerve where they exit from their respective foramina along the brow line.
A skin wheal is placed over the glabella. A 25-gauge needle, bent to aid in superficial placement, is inserted through the anesthetizing wheal and advanced laterally along the brow. A total of 8 mL of local anesthetic is applied from the glabella to the lateral edge of each brow.
Greater and Lesser Occipital Nerves
Indications: closure of lacerations
By blocking the greater and lesser occipital nerves, the posterior scalp can be anesthetized. By placing a track of local anesthetic from the mastoid process to the inion (i.e., external occipital protuberance) along the highest nuchal line from each side, both the greater and lesser occipital nerves will receive a dose of local anesthetic and the posterior scalp will become anesthetized.
A large skin wheal is placed over the mastoid process on each side using a 27-gauge needle. Then through this wheal, a wheal is placed from the mastoid process to the inion using a 25-gauge Quincke needle that is bent to facilitate a superficial injection.
Infraorbital Nerve
Indications: closure of lacerations, facial surgery
The maxillary division of the trigeminal nerve innervates the midface, from the inferior portion of the orbit to the mandible. This area includes the area overlying the zygoma, the maxilla, and most of the nose, as well as the philtrum and the hard and soft palate.
The infraorbital foramen is palpable 2 to 3 mm below the rim of the orbit, just medial to the equator of the orbit. A small-gauge needle is used to inject local anesthesia just outside the foramen. Avoid injection into the foramen as the nerve is located in a confined space.
A small amount (2–4 mL) of local anesthetic is sufficient and it should be injected based on which area is to be anesthetized, i.e., emphasize above the foramen for lower lid work, medial to the foramen for lateral nasal work, and inferomedial to the foramen for work on the philtrum.
Blocks of the Neck
Superficial Cervical Plexus
The cervical plexus is composed of four nerve roots, C1 –C4, and terminates in four branches: lesser occipital, great auricular, transverse cervical, and supraclavicular nerves. The terminal branches emerge superficially at the posterior border of the sternocleidomastoid (SCM) muscle along the mid-portion of the muscle.
This is a purely cutaneous nerve block; there is no motor block with the superficial cervical plexus block. Neuromonitoring and stimulation of the recurrent laryngeal nerve is not compromised when using this block.
The patient is positioned in the seated position. A line connecting the insertion of the SCM at the midpoint of the clavicle to the mastoid process along the posterior muscle border designates the path in which subcutaneous local anesthetic should be injected. Initially, 3 to 5 mL of local anesthetic is injected at the midpoint of the SCM using a 27-gauge needle. Using a 25-gauge Quincke point spinal needle, subcutaneous injections are then performed from this initial injection site in caudad and cephalad directions along the posterior edge of the SCM. Infiltration along these paths should require 6 to 8 mL of anesthetic in each direction. Aspiration prior to injection is important to avoid intravascular injection.
Deep Cervical Plexus
The deep cervical plexus (DCP) is the collection of the C2 –C4 nerve roots as they exit the “gutter” formed by the transverse process of the respective vertebrae. By injecting prior to the division of the cervical roots into dorsal and ventral spinal nerves, a more complete blockade of the ipsilateral neck is achieved—including both sensory and motor elements. This is not commonly used for otolaryngology procedures.
The patient is seated upright in a high Fowler position with a small towel behind the shoulders. The above-mentioned line is drawn between the mastoid process and the anterior tubercle of C6, which is palpable in the vast majority of patients. A line parallel to this is drawn 1.5 cm behind the first, and the posterior tubercle is palpated on C2, C3, and C4. Deep palpation can be uncomfortable and a light touch is indicated. After a small skin wheal with a 27-gauge needle, a short (2.5 cm) blunt needle is advanced to the posterior process of each of the three vertebrae and then “walked” laterally and anterior to the posterior tubercle. An advance of 1 mm beyond the bony tubercle will suffice. It is not uncommon for the patient to describe a light paresthesia in the dermatome of the root being blocked. After careful aspiration, 4 to 5 cc of local anesthetic (with epinephrine) is injected at each of the three levels. (C2, C3, C4). The proximity to the spinal column and major vascular structures increases the risk of intrathecal or intravascular injection.
Specific Nerve Blocks for the Upper Airway
Maxillary Division of the Trigeminal Nerve (Sphenopalatine Ganglion)
• The transnasal topical approach to the sphenopalatine ganglion involves application of local anesthetic to the mucous membranes surrounding the ganglion.
• Position the patient supine with neck extension. A local anesthetic (typically 80 mg of 4% cocaine) is applied to each nostril. Cotton-tipped applicators soaked in 4% cocaine are gently swirled and advanced into the nares. Each applicator is advanced a little further than the one prior, and once placed the applicator is left there as successive applicators are introduced. Each nostril should receive four to seven applicators as the opening allows. The applicators should remain in the nares for at least 20 minutes allowing the local anesthetic to diffuse through the mucosa overlying the ganglion.
• The sphenopalatine ganglion can also be approached through the greater palatine foramen located at the posterior portion of the hard palate. In this approach, the patient is positioned in the supine position with the neck extended. The foramina can be palpated medial to the gumline of the third molar. A small-gauge needle is advanced <2.5 cm through the foramen in a superior and slightly posterior direction. To avoid an intravascular injection, aspirate prior to injection.
Glossopharyngeal Nerve
• The glossopharyngeal nerve (CN IX) exits the skull at the jugular foramen and passes between the internal jugular vein and the internal carotid artery. It descends just dorsal to the styloid process before curving forward and anterior to innervate the palatine tonsil, the mucous membranes of the fauces and the base of the tongue. This nerve has motor, sensory, and autonomic components, and supplies lower motor neurons to the stylopharyngeus and parasympathetic innervation of the parotid and mucous glands.
Superior Laryngeal Branch of the Vagus Nerve
• The superior laryngeal nerve can be blocked as it passes into the thyrohyoid membrane inferior to the greater cornu of the hyoid bone and superior to the greater cornu of the thyroid cartilage. This block will provide anesthesia to the glottis above the vocal folds.
• With the patient seated in an upright (high Fowler position) with a towel roll transversely laid behind the shoulders, the thyroid cartilage is palpated. It can be helpful to lightly displace the thyroid cartilage toward the side of the block. Using a small-gauge needle, 2 to 3 mL of local anesthetic is injected near the cartilaginous greater cornu. Aspiration prior to injection will confirm that the needle has not entered the supraglottic air column. This procedure is then repeated on the other side.
Topical Anesthesia of the Subglottic Airway
• The recurrent laryngeal branch of the vagus nerve pierces the subglottic trachea to innervate all the laryngeal muscles other than the cricothyroid muscle as well as provide sensory innervation to the subglottic mucosa.
• The patient is positioned in a high Fowler position with a towel roll laid transversely behind the shoulders. Moderate neck extension is helpful. After skin disinfection, the thyroid cartilage is identified. The next palpable cartilage inferiorly is the cricoid cartilage. The palpable gap between these two structures overlies the cricothyroid membrane. A 22-gauge needle containing local anesthetic (2 to 4 mL 3% chloroprocaine or 2 to 4 mL 4% lidocaine) is advanced perpendicular to the skin while gentle aspiration is applied to the syringe plunger. Air will be freely aspirated when the needle penetrates the cricothyroid membrane, entering the trachea. The patient should be alerted that the injection will induce coughing. The local anesthetic should be rapidly administered and the needle withdrawn. The patient will cough and should be encouraged to do so several times to enhance spread of the anesthetic.
Further Reading
Barash PG, Cullen BF, Stoelting RK, et al. Clinical Anesthesia. 6th ed. Philadelphia, PA: Lippincott Williams & Wilkins; 2009
Morgan GE, Mikhail MS, Murray MJ. Clinical Anesthesiology. 4th ed. New York: McGraw-Hill Medical; 2005
1.4.3 Anesthesia Drugs
Opioids
Opioids work by binding to specific receptors located throughout the central nervous system (CNS) and other tissues (Table 1.11). The pharmacodynamic effect of the administered opiate depends on which receptor is bound, the affinity of the binding, and whether the receptor is activated or inhibited. There are four opioid receptors: mu, delta, kappa, and sigma (Table 1.12).
Morphine
Because morphine is a hydrophilic compound it has a slower onset with a longer clinical effect. Morphine can lead to hypotension secondary to histamine-induced vasodilation as well as decreased sympathetic tone. Morphine is metabolized in the liver to morphine 3-glucuronide and morphine 6-glucuronide, metabolites that are excreted by the kidneys. Patients with renal failure can have prolonged duration of action given that between 5 and 10% of morphine is excreted unchanged in the urine. Morphine 6-glucuronide can lead to respiratory depression and narcosis.
Dosing: Preoperatively, postoperatively 5–15 mg intramuscular (IM)/IV; 0.05–0.2 mg/kg IV
Fentanyl
Fentanyl is a synthetic compound with a higher lipid solubility; fentanyl has a rapid onset and short duration of action. Because of fentanyl’s high lipid solubility it can accumulate in adipose tissue creating a “reservoir.” Prolonged respiratory depression is seen after large doses or prolonged fentanyl infusions.
Dosing: 0.5–1 μg/kg IV, 1–5 μg/kg per hour infusion. Larger doses may be used during general anesthesia.
Remifentanil
Remifentanil is an ultrafast acting narcotic with an elimination half-life of less than 10 minutes. The ester structure of this drug renders it susceptible to metabolism by plasma and tissue esterases. Remifentanil is metabolized so efficiently that during long infusion times the drug is not allowed to accumulate in adipose or other tissues. The length of infusion time therefore has no effect on wake-up time. Because the biotransformation of remifentanil is extrahepatic, narcotic toxicity can be avoided in patients with hepatic dysfunction.
Dosing: 0.025–0.2 μg/kg per minute infusion
Organ System | Physiologic Response |
---|---|
Cardiovascular | In general, opioids do not impair cardiovascular function. |
At high doses opioids can lead to bradycardia. | |
Meperidine, structurally similar to atropine, can cause tachycardia. | |
Hypotension seen with opioid use is secondary to bradycardia and in the case of morphine and meperidine histamine-induced vasodilatation. | |
Respiratory | Opioids depress ventilation, particularly respiratory rate. |
The hypoxic drive, the body’s ventilatory response to CO2, is decreased. The result is an increase in PaCO2 and decreased respiratory rate. | |
The apneic threshold, the highest PaCO2 at which a patient will remain apneic, is increased. | |
In patients susceptible to histamine-induced reactive airway disease, morphine and meperidine can lead to bronchospasm. | |
Chest wall rigidity, severe enough to prevent adequate ventilation, can be seen with fentanyl and remifentanil. | |
Central nervous system | Opioids reduce cerebral oxygen consumption, cerebral blood flow and intracranial pressure. |
Minimal changes are seen on EEG except for meperidine, which can cause an increase in EEG frequency. | |
Even at high doses opioids do not reliably produce amnesia. The high doses necessary to establish unconsciousness can lead to physical dependence. | |
Gastrointestinal | Opioids slow peristalsis resulting in decreased gastric emptying and constipation. |
Contraction of the biliary sphincter leading to biliary colic is also common. | |
Endocrine | Opiates block the release of catecholamines, ADH, and cortisol associated with surgical stress. |
Drug interactions | Combined with opiates barbiturates, benzodiazepines, and other CNS depressants have a synergistic effect on level of sedation and respiratory depression. |
Abbreviations: ADH, antidiuretic hormone; CNS, central nervous system; EEG, electroencephalogram.
Meperidine
Meperidine (Demerol, Sanofi-Aventis Pharmaceuticals, Paris, France) is N-demethylated in the liver to normeperidine, an active metabolite that is associated with myoclonus and seizures. Patients with renal disease are particularly susceptible as this metabolite is renally cleared. Meperidine is useful in the postanesthesia care unit (PACU) setting in the treatment of shivering. Note that postoperative oral meperidine use/prescription is discouraged due to adverse side effects.
Dosing: Preoperatively; postoperatively 50–150 mg IM/IV
Receptor | Physiological Characteristics |
---|---|
Mu | μ1 receptor is responsible for producing analgesia, miosis, nausea/vomiting, urinary retention, and pruritus. |
The endogenous stimulus for μ1 receptors are enkephalins. | |
μ2 receptor activation leads to euphoria, respiratory depression, sedation, bradycardia, ileus, and physical dependence. | |
Delta | Activation leads to analgesia and contributes to physical dependence. |
These receptors are highly selective for endogenous enkephalins. | |
Kappa | Activation leads to analgesia, sedation, dysphoria, and psychomimetic effects. |
Pure Kappa agonists do not lead to respiratory depression. | |
Stimulation leads to vasopressin release and subsequent diuresis. | |
Sigma | Activation leads to dysphoria, hallucinations, tachypnea, and mydriasis. |
Reversal of Opioids
Naloxone
Naloxone is a competitive antagonist at opioid receptors, particularly the mu class. There is no significant agonist activity. Naloxone is indicated in cases of narcotic overdose. Respiratory depression secondary to narcotic overdosage is rapidly reversed with naloxone (1–2 minutes). Care should be taken to titrate low doses as abrupt reversal of analgesia can result in abrupt sympathetic stimulation and acute withdrawal symptoms in those who are opioid dependent. Naloxone has a short duration of action (30–40 minutes) and redosing is usually required when reversing long-acting opioids.
Dosing: 0.5–1 μg/kg every 5–10 minutes; maximum total dose of 10 mg
Benzodiazepines
Benzodiazepines interact with specific receptors in the CNS that enhance the action of specific neurotransmitters (Table 1.13). In particular, the action of gabapentin (GABA), an inhibitory neurotransmitter, is enhanced. As a result, benzodiazepines produce amnesia, anxiolysis, sedation, and prevent seizures. Benzodiazepines have no direct analgesic properties. Like barbiturates, benzodiazepines are highly protein-bound and rely on redistribution for their offset of action. Biotransformation of benzodiazepines occurs in the liver and metabolites are excreted mainly in the urine. Duration of action may be prolonged in patients with renal failure as metabolites are pharmacologically active.
Organ System | Physiologic Response |
---|---|
Cardiovascular | Minimal depression of the cardiovascular system even at high doses. |
Slight decreases in arterial blood pressure, cardiac output, and peripheral vascular resistance are often observed. | |
Midazolam decreases arterial blood pressure and peripheral vascular resistance more than lorazepam or diazepam. | |
Variability in heart rate during administration suggests that Midazolam has vagolytic properties. | |
Respiratory | Ventilatory response to CO2 is reduced with benzodiazepine administration. This response is particularly pronounced when administered with other respiratory depressants, such as opioids. |
Neurologic | Benzodiazepines are very effective in seizure prophylaxis. |
Cerebral oxygen consumption and cerebral blood flow are reduced. | |
Drug interactions | When administered with opiates, benzodiazepines work synergistically to depress ventilation. |
When administered with heparin, diazepam is displaced from its protein binding sites causing an increased free drug concentration. | |
The MAC of volatile anesthetics is reduced up to 30%. CNS depressants such as alcohol and barbiturates potentiate the sedative effects of benzodiazepines. |
Abbreviations: CNS, central nervous system; MAC, minimum alveolar concentration.
Diazepam
Diazepam is insoluble in water and requires propylene glycol, which may cause venous irritation. Diazepam has a long duration of action secondary to slow hepatic extraction and a large volume of distribution. The elimination half-life is nearly 30 hours.
Dosing: 5–20 mg orally (PO), 2–10 mg IV
Midazolam
At low pH, midazolam is water soluble. At physiologic pH, midazolam becomes more lipid soluble resulting in fast onset of action. Midazolam has the shortest elimination half-life (2 hours) because of a high hepatic extraction ratio. Midazolam’s high potency and steep dose response curve require careful titration and close monitoring of respiration as even small doses can lead to apnea.
Dosing: Adult 3–5 mg IM, 0.5–5 mg IV; pediatric 0.025–0.1 mg/kg IV; 0.25–0.5 mg/kg PO 30 minutes before procedure
Lorazepam
Like diazepam, lorazepam is insoluble in water and requires propylene glycol, which may cause venous irritation when administered. Because of its moderate lipid solubility lorazepam has a slower onset of action secondary to slower brain uptake. The lower lipid solubility of lorazepam limits its volume of distribution and decreases its elimination half-life (15 hours) despite the same hepatic extraction ration of diazepam.
Dosing: Adult 1–4 mg PO/IM/IV; pediatric 0.05 mg/kg PO/IM/IV preoperatively
Benzodiazepine Reversal
Flumazenil
Flumazenil is a specific benzodiazepine receptor antagonist that reverses most of the effects of benzodiazepines. Onset is rapid (<1 minute) for the hypnotic effects of benzodiazepines. The amnestic effect is less reliably reversed. This agent is a competitive inhibitor at the benzodiazepine receptor. Elderly patients in particular are prone to resedation and should be observed for respiratory depression after flumazenil administration.
Dosing: 0.2 mg (titrate every minute until desired degree of sedation reversal is observed). Sedation reversal typically requires 0.6–1.0 mg. Infusions at 0.5 mg/h are indicated for overdoses of long-acting benzodiazepines.
Alpha-2 Agonists
Dexmedetomidine
Dexmedetomidine (brand name Precedex, Hospira, Inc., Lake Forest, IL) is an β2 agonist with 8 to 10 times greater receptor affinity than clonidine. It has sympatholytic, analgesic, and sedating properties. The effect on the cardiovascular system is to lower heart rate and blood pressure, blunting the typical surgical response. β2 Agonists have minimal respiratory depression. Head and neck surgeons will find this drug useful for conscious sedation cases, augmented sleep studies, and fiberoptic intubations and tracheotomy placement. Also of interest to the otolaryngologist who employs the use of topical cocaine intraoperatively, recent research has suggested dexmedetomidine to be an effective treatment for the dangerous cardiovascular symptoms of cocaine intoxication.
Dosing: Loading dose of 1 μg/kg over 10 minutes followed by IV infusion at 0.2–0.7 μg/kg per hour
Anesthesia Induction Medications
Propofol
Propofol is a rapidly acting induction agent, which produces unconsciousness within 30 seconds of dosing and effects last between 2 to 8 minutes (Table 1.14). Propofol enhances the inhibitory action of GABA. Propofol is metabolized in the liver; however, offset of action results from redistribution, which is rapid secondary to high lipid solubility. Compared with other induction agents, propofol provides a faster recovery with less “hangover” than barbiturates or etomidate. Additionally, this agent has antiemetic, antipruritic and anticonvulsion properties. At low (subhypnotic) doses (10–15 mg) propofol can ameliorate nausea and vomiting. Propofol does not provide analgesia, but does enhance the analgesic effects of narcotics. Careful titration is advised in hypovolemia or coronary vascular disease, as propofol can lead to a profound decrease in blood pressure secondary to decreased systemic vascular resistance. Venous irritation with administration can be avoided with concomitant administration of lidocaine (20–80 mg). Because propofol is an emulsion it should be avoided in patients with disorders in lipid metabolism.
Dosing: Induction 2–2.5 mg/kg IV (pediatric dosing: 2.5–3.5 mg/kg); infusion 100–200 μg/kg per minute; sedation 25–75 μg/kg per minute
Organ System | Physiologic Response |
---|---|
Cardiovascular | Increased peripheral vasodilatation, decreased cardiac contractility, and decreased preload combine to cause hypotension. |
The normal vagal response to hypotension is also impaired. | |
The hypotension with propofol is greater than that produced by barbiturates. | |
Respiratory | Respiratory depression |
Central nervous system | Reduced intracranial pressure by reducing cerebral blood flow |
Etomidate
Etomidate depresses the reticular activation system by binding to the GABA receptors and enhancing the inhibitory effects of this neurotransmitter (Table 1.15). Etomidate is dissolved in propylene glycol leading to pain on injection. This can be reduced by injecting lidocaine prior to induction. Myoclonic movements are common after etomidate induction. Etomidate is characterized by a rapid onset secondary to high lipid solubility at physiologic pH. Etomidate is metabolized into inactive end products by hepatic microsomal enzymes and plasma esterases. Metabolites are excreted in the urine. Etomidate has very little effect on the cardiovascular system and is therefore the induction agent of choice in cardiovascular disease and severe hypovolemia.
Dosing: induction 0.2–0.6 mg/kg
Ketamine
Ketamine has multiple effects through the CNS and has been demonstrated to be an N-methyl-D-aspartate (NMDA) antagonist (Table 1.16). By effectively “disconnecting” the thalamus from the limbic system, a state of “dissociative anesthesia” results. In this state the patient appears conscious, but is unable to process or respond to sensory stimulation. Ketamine is a structural analogue to phencyclidine (PCP), and as with PCP, hallucinations can ensue even at low doses. Ketamine is metabolized in the liver, resulting in pharmacologically active metabolites (norketamine). Products of hepatic metabolism are renally excreted.
Organ System | Physiologic Response |
---|---|
Cardiovascular | Slight decrease in peripheral vascular resistance leads to slight decrease in arterial blood pressure. |
Myocardial contractility and cardiac output are unchanged. | |
Respiratory | Ventilation is reduced less than with other induction agents. |
Even at induction doses, apnea usually does not occur unless opioids are also administered. | |
Central nervous system | Cerebral metabolic rate, cerebral blood flow, and intracranial pressure are reduced. |
Due to cardiovascular stability, cerebral perfusion pressure is maintained. | |
Etomidate lacks analgesic properties. | |
Endocrine | Transient inhibition of enzymes responsible for cortisol and aldosterone synthesis occurs with intubation doses. |
Long-term infusions lead to adrenocortical suppression. |
Dosing: 1–2 mg/kg IV; 3–5 mg/kg IM
Organ System | Physiologic Response |
---|---|
Cardiovascular | Increased cardiac output, heart rate, and blood pressure secondary to increased sympathetic outflow |
Respiratory | Minimally effects ventilatory drive |
Ketamine is a potent bronchodilator and has benefits to patients with asthma. | |
Increased salivation can be resolved by pretreatment with anticholinergic medications. | |
Central nervous system | Increased cerebral blood flow, cerebral oxygen consumption, and intracranial pressure |
Hallucinations, delirium, and disturbing dreams are decreased in children and those who receive benzodiazepines prior to ketamine. | |
Ketamine produces analgesia, amnesia, and unconsciousness. |
Barbiturates
Barbiturates have several sites of action resulting in suppression of excitatory neurotransmitters and activation of the inhibitory effects of GABA. The result is inhibition of the reticular activation system. Methohexital and thiopental are the commonly used barbiturates used for induction. As more titratable induction agents have come into use, barbiturates have fallen out of favor. Barbiturates have no analgesic properties and cause dose-related depression of the respiratory, cardiac, and central nervous systems. Thiopental has a short duration of action secondary to a high rate of redistribution from the brain to inactive tissues, which secondary to a high lipid solubility. Barbiturates are contraindicated in patients with intermittent porphyria. Side effects include venous irritation, myoclonus, and hiccupping.
Dosing: Thiopental 3–6 mg/kg IV; methohexital 1–2 mg/kg IV
Inhaled Anesthetics
In the OR, general anesthesia is commonly maintained with inhaled anesthetics. These agents also provide some analgesia, amnesia, and muscle relaxation. In pediatric patients in whom there is no IV access, anesthesia may be induced by inhalation. All of the inhaled anesthetics, with the exception of nitrous oxide, are bronchodilators and may be useful in those with reactive airways. Most inhaled agents reduce blood pressure. The onset of anesthetic induction as well as emergence from anesthesia is based on the lipid solubility characteristics of the inhaled anesthetic: the more insoluble the anesthetic agent, the faster the induction of anesthesia. The agents with high lipid solubility prolong the emergence from anesthesia.
Minimum alveolar concentration (MAC) is defined as the alveolar concentration at which 50% of test subjects will not respond to a surgical stimulus. Dosing of inhaled agents is based on the MAC of each particular agent. The MAC of inhaled agents depends on the individual gas properties of each agent. MAC is additive such that ½ MAC isoflurane combined with ½ MAC nitrous oxide is equivalent to one MAC of sevoflurane.
Isoflurane
Compared with other inhaled anesthetics (sevoflurane, desflurane), isoflurane has a relatively high lipid solubility, leading to increased induction and emergence time. Isoflurane causes minimal cardiac depression and decreased blood pressure secondary to decreased systemic vascular resistance. Like other volatile anesthetics, isoflurane causes respiratory depression with a decrease in minute ventilation (Table 1.17). Despite its ability to cause airway irritation, isoflurane induces bronchodilation.
Desflurane
Other than the substitution of a fluoride atom for a chloride atom, the structure of desflurane is very similar to that of isoflurane. This composition makes desflurane highly insoluble. Because of its low lipid solubility, induction and emergence from anesthesia are rapid. The time required for patients to awaken is approximately half as long as that observed following isoflurane administration. Desflurane has cardiovascular and cerebral effects similar to those of isoflurane. Like isoflurane this agent is irritating to the airway making gas induction difficult.
Organ System | Physiologic Response |
---|---|
Cardiovascular | Decreased systemic vascular resistance leads to lower arterial blood pressure. |
Respiratory | Can cause airway irritation and cause bronchodilation |
Respiratory depression | |
Neurologic | At high concentrations, increased cerebral blood flow and intracranial pressure can develop. |
Decreased cerebral metabolic oxygen requirements may provide cerebral protection. | |
Neuromuscular | Skeletal muscle blood flow is increased. |
Renal | Decreases renal blood flow, glomerular filtration rate, and urinary output. |
Sevoflurane
Sevoflurane is the primary inhaled anesthetic agent used in anesthesia induction when an IV induction cannot be performed, such as in pediatric patients. Nonpungency and a rapid increase in alveolar anesthetic concentration make it an excellent choice where inhalational induction is necessary. The blood solubility of sevoflurane is slightly greater than that of desflurane. Sevoflurane mildly depresses myocardial contractility and systemic vascular resistance. Arterial blood pressure declines slightly less than with isoflurane or desflurane. Like isoflurane and desflurane, sevoflurane causes slight increases in cerebral blood flow and intracranial pressure.
Nitrous Oxide
The uptake and elimination of nitrous oxide are relatively rapid compared with other inhaled anesthetics. This is the result of its low blood–gas partition coefficient. Nitrous oxide produces analgesia, amnesia, mild myocar-dial depression, and mild sympathetic nervous system stimulation. It does not significantly affect heart rate or blood pressure. Nitrous oxide is a mild respiratory depressant, although less so than the volatile anesthetics. The elimination of nitrous oxide is via exhalation.
Muscle Relaxation
Neuromuscular blocking agents are used most commonly for facilitation of endotracheal intubation and when patient movement is detrimental to the surgical procedure. Prior to administration, ventilation must be ensured by the anesthesiologist. Ventilation can be achieved with a mask until the endotracheal tube is placed in the trachea. Neuromuscular blockers have no intrinsic sedative or analgesic properties and must be used in concert with anesthetic agents. Inadequate sedation and hypnosis while using neuromuscular blockers can produce recall by patients causing long-term side effects. There are two classifications of neuromuscular blocking agents: depolarizing and nondepolarizing.
Depolarizing Muscle Relaxants
Depolarizing agents have a similar chemical structure to acetylcholine. They induce paralysis by binding to acetylcholine receptors at the skeletal muscle neuromuscular junction causing depolarization. Paralysis ensues because these agents have a higher affinity for the postsynaptic receptor preventing the reestablishment of its ionic gradient. Clinically, fasciculations are seen prior to relaxation after dosing. The only medication in this class that is still in use today is succinylcholine (Table 1.18).
Nondepolarizing Muscle Relaxants
Nondepolarizing muscle relaxants induce paralysis by binding to the postsynaptic receptor at the skeletal muscle neuromuscular junction. Essentially, these medications compete with acetylcholine for binding sites at the receptor. Unlike depolarizing blockade, the postsynaptic receptors are not activated and fasciculations do not occur. Nondepolarizing blockade can be reversed by increasing the acetylcholine concentration at the neuromuscular junction. This is achieved by administration of medications such as neostigmine, which prevent the breakdown of acetylcholine. The commonly used nondepolarizing agents are summarized in Table 1.19.
Agent | Intubating Dose, Onset, Duration | Clinical Considerations |
---|---|---|
Succinylcholine | Dose: 1–1.5 mg/kg Onset: 30–60 s Duration:<10 min Maximum: 150 mg total dose | Succinylcholine is contraindicated for routine intubation in pediatric patients because of the risk of cardiac arrest with hyperkalemia in those with undiagnosed myopathies. Agent of choice for rapid sequence induction. Bradycardia can follow dosing, particularly in pediatric patients. Fasciculations with receptor activation typically occur and can result in myalgias postop. Potassium release with succinylcholine-induced depolarization can increase by 0.5 mEq/dL. Avoid in hyperkalemic patients. Transient increases in intracranial and intraocular pressure Avoid in patients with a history of malignant hyperthermia Metabolized by pseudocholinesterase |
Agent | Intubating Dose, Onset, Duration | Clinical Considerations |
---|---|---|
Rocuronium | Dose: 0.5–0.9 mg/kg IV Onset: 1–2 min Duration: 40–90 min | At doses of 0.9–1.2, rocuronium can have rapid onset (60–90 s) and substituted for succinylcholine for rapid sequence induction. Rocuronium undergoes no metabolism and is eliminated in the bile and slightly by the kidneys. Severe hepatic failure and pregnancy can prolong duration of action. |
Vecuronium | Dose: 0.1 mg/kg IV Onset: 2–3 min Duration: 25–30 min | Vecuronium has a short duration of action. Hepatic metabolism with renal excretion Lacks hemodynamic side effects Can be administered as an infusion (1–2 μg/kg/min) |
Cisatracurium | Dose: 0.2 mg/kg IV Onset: 1–2 min Duration: 50–60 min | Cisatracurium undergoes organ-independent Hoffman degradation at physiologic pH and temperature. May be safely administered to patients with renal or liver failure Lacks hemodynamic side effects |
Pancuronium | Dose: 0.1 mg/kg IV Onset: 5 min Duration: 60–100 min | Provides long duration of action Renal elimination Has vagolytic effects causing tachycardia, particularly after bolus administration |
Further Reading
Barash PG, Cullen BF, Stoelting RK, eds. Clinical Anesthesia. 6th ed. Philadelphia, PA:Lippincott Williams & Wilkins; 2009
Morgan GE, Mikhail MS, Murray MJ. Clinical Anesthesiology. 4th ed. New York: McGraw-Hill Medical; 2005
1.4.4 Anesthetic Emergencies
Potential anesthetic emergencies are numerous. Emergencies related to management of the airway are covered in Chapters 1.2 and 6.1. Importance of prevention, early recognition, and prompt appropriate management are common to all potential anesthetic emergencies. In particular, airway fire and malignant hyperthermia are conditions with the potential for high morbidity or mortality and are thus reviewed here.
Airway Fires
The prevention of airway fires is crucial. Lower oxygen concentration to 21 to 40% FiO2 as tolerated when using cautery intraorally (i.e., tonsillectomy) or a laser. Laser precautions such as the use of a laser-safe endotracheal tube and packing and covering surrounding field with wet gauze or towels must be standard. Be sure there is not an endotracheal tube cuff leak. Turn off oxygen temporarily when using cautery during facial procedures requiring sedation and nasal cannula. Communication between the anesthetist and the surgeon is crucial. If there is a fire, turn off the oxygen, turn off the nitrous oxide, immediately remove the endotracheal tube, extinguish and remove any burning material, and reintubate the patient.
Malignant Hyperthermia
Malignant hyperthermia (MH) is a hyperdynamic crisis caused by an uncontrolled increase in skeletal muscle metabolism (Table 1.20). The underlying pathophysiology is an inability of the sarcoplasmic reticulum to control intracellular calcium causing prolonged activation of muscle contractile units. This results in heat production, oxygen consumption, and acidosis. MH is an autosomal dominant myopathy that is triggered by inhalational anesthetics and depolarizing muscle relaxants (e.g., succinylcholine). “Triggers” should be avoided in those who have a history of MH or a family history of the disorder. Patients with a history of MH can be safely anesthetized with a “nontriggering anesthetic,” which includes IV agents (TIVA) and nondepolarizing agents. Complications from MH are severe: they include cardiac arrest, cerebral or pulmonary edema, renal failure, diffuse intravascular coagulation (DIC), and death. Treatment is outlined in Table 1.21.
Unexplained tachycardia, tachypnea, and/or rigidity |
Increased end tidal carbon dioxide |
Hyperthermia |
Hypoxia/cyanosis |
Arrhythmias |
Hyperkalemia |
1. Stop anesthesia and surgery |
2. Hyperventilate with 100% oxygen |
3. Administer dantrolene 2 mg/kg every 5 min to 10 mg/kg |
4. Treat hyperthermia: institute cooling measures |
5. Treat hyperkalemia: insulin (10 units) with glucose (50 mL D50) |
6. Treat acidosis: bicarbonate 2–4 mEq/kg slow intravenous push |
7. Treat arrhythmias |
8. Invasive monitoring: Foley catheter, arterial line, central venous catheter |
9. Administer high volumes of crystalloid, as tolerated |
Further Reading
Barash PG, Cullen BF, Stoelting RK, et al. Clinical Anesthesia. 6th ed. Philadelphia, PA: Lippincott Williams & Wilkins; 2009
Morgan GE, Mikhail MS, Murray MJ. Clinical Anesthesiology. 4th ed. New York: McGraw-Hill Medical; 2005
1.5 Fluids and Electrolytes
Key Features
• Appropriate fluid balance perioperatively can be estimated from established formulas.
• Attention to fluids and electrolytes is especially important in the patient who is not capable of maintaining normal oral intake.
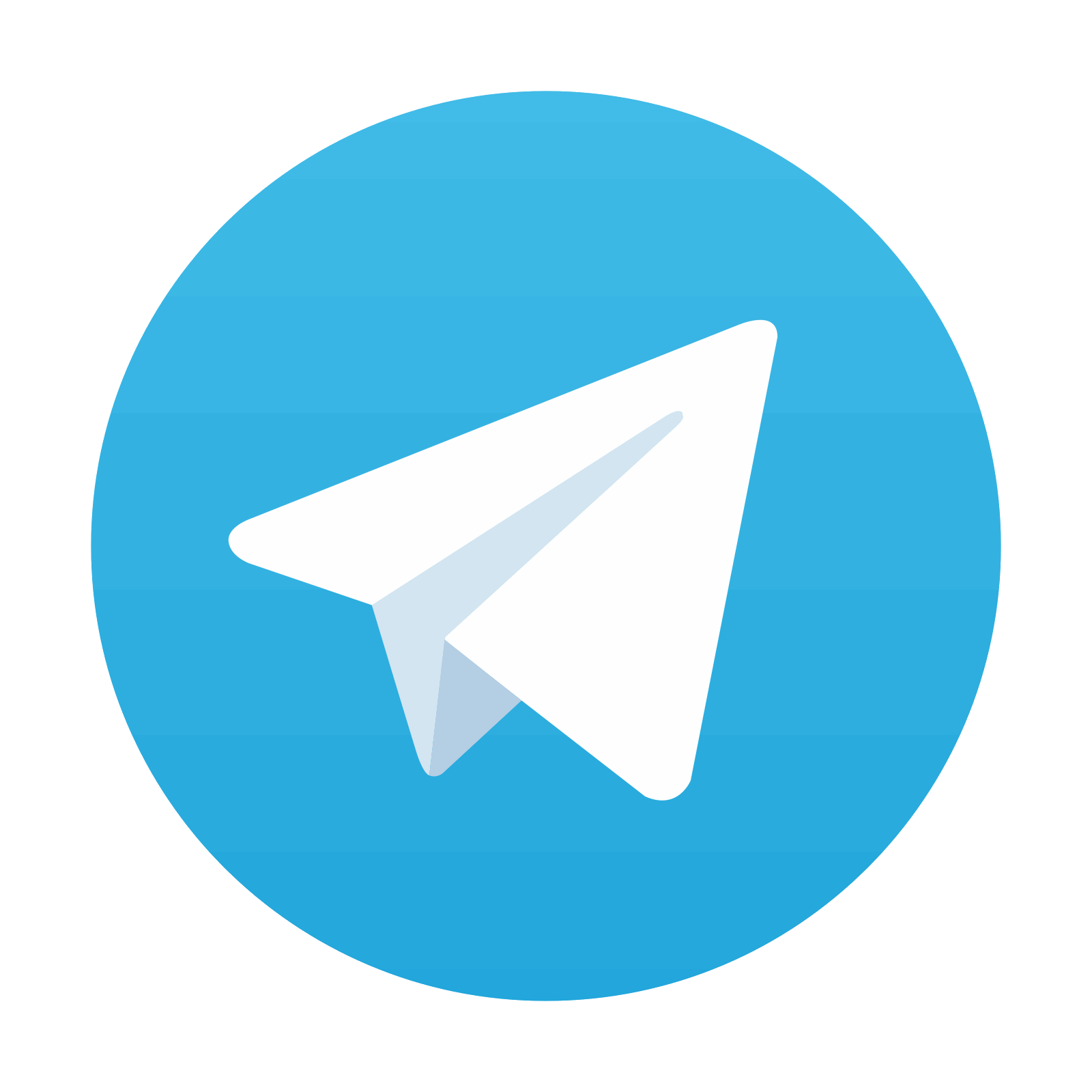
Stay updated, free articles. Join our Telegram channel

Full access? Get Clinical Tree
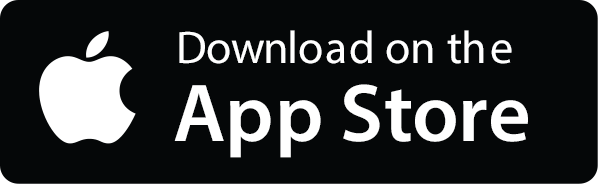
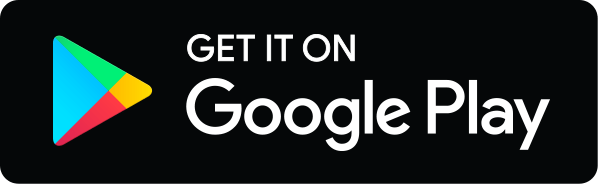