1 History, Principles, and Instrumentation of Optical Coherence Tomography
1.1 History
Optical coherence tomography (OCT) is a newer medical investigational modality that has been widely used in ophthalmology. It performs real-time, high-resolution, micrometer-scale, cross-sectional imaging of ocular tissues in less time. Although it has been used in other medical fields, the ophthalmologic specialty has used this application widely for the diagnosis, prognosis, and management of ocular pathologies. OCT uses low-coherence interferometry to produce a two-dimensional image of optical scattering from internal tissue microstructures in a way that is analogous to ultrasonic pulse-echo imaging.
The noninvasive nature and higher resolution compared with other imaging techniques, such as ultrasound, magnetic resonance imaging, and computerized tomography, have made OCT one of the standard diagnostic tests for many ocular disorders. OCT was first used for in vitro imaging in the peripapillary area of the retina and in the coronary artery, two clinically relevant examples that are representative of transparent and turbid media, respectively. In 1991, Huang and associates demonstrated the OCT evaluation of the retina using infrared light of ~ 800-nm wavelength and axial resolution 10 µm. 1 They reported OCT correlation with the histologic appearance of the retina. Their images were displayed as false color scale, depending on the magnitude of the backscattering. OCT was also used in cadaver eyes for ex vivo imaging of the retina and optic nerve head. It was the effort and vision of the physical scientists and ophthalmologists, namely, Doctors David Huang, Joel Schumann, James Fujimoto, Eric Swanson, and Carmen A. Puliafito, that led to the conversion of the interferometry principle to a widely used imaging modality in the eye. 2 OCT gained popularity in ophthalmology as a result of the transparent nature of the ocular media and the ease in evaluation of anterior and posterior segments of the eye.
Initially, OCT was used for the evaluation of retinal structures and the optic nerve head. 3 , 4 , 5 , 6 The wavelength of light used for retinal study was 830 nm; however, this wavelength was not adequate for imaging the anterior ocular structures, such the cornea, anterior-chamber, angles, and lens. OCT imaging of the anterior segment with a longer wavelength of 1310 nm was developed later. Izatt et al first introduced micrometer-scale resolution imaging of the anterior eye with OCT in 1994. 7 It had the advantages of better penetration through sclera as well as real-time imaging at eight frames per second. 8
Since the introduction of OCT in ophthalmology, this imaging method has been used extensively for the diagnosis of various clinical disorders of the retina. Quantification of macular edema, diagnosis of central serous retinopathy, grading of macular holes, assessment of age-related macular degeneration, and determination of epiretinal membrane are some of the widely applied fields of macular OCT. 9 , 10 , 11 , 12 , 13 In analysis of the optic nerve head, OCT was initially used for determination of the thickness of the retinal nerve fiber layer, which is an important parameter for glaucoma assessment. 5 , 14 In 1995, Schuman and associates showed that the thickness of the retinal nerve fiber layer as measured by OCT demonstrated a high degree of correlation with the functional status of the optic nerve as measured by visual field examination. 5 Emergence of OCT in anterior-segment evaluation has opened the new world of using OCT for corneal surgeries, especially refractive surgeries. 15 , 16 , 17 , 18 With the evolution of OCT, new clinical entities have been identified, and innovative OCT-guided surgical techniques are being performed. 19 , 20 , 21 , 22 The last decade saw massive innovative applications of OCT in ophthalmology. 18 , 19 , 20 , 21 , 22 , 23 , 24 High-speed anterior-segment OCT has been applied to visualize anywhere from the cornea to the lens. 24 , 25 , 26 , 27 In 2010, anterior-segment OCT was combined with femtosecond laser for cataract extraction. 28
1.2 Principle
Interferometry is the basic physical principle used in OCT. The Michelson interferometer causes interference by splitting a beam of light into two parts. Each part is made to travel a different path and brought back together, where they interfere according to their path length difference. 2
1.2.1 Coherence
Coherent lights are light waves that are “in phase” with one another (Fig. 1.1a). Two waves are coherent if the crests of one wave are aligned with the crests of the other and the troughs of one wave are aligned with the troughs of the other. Otherwise, these light waves are considered incoherent. Light produced by lasers is coherent light. Light from light bulbs or the sun, however, is incoherent light (Fig. 1.1b,c).

1.2.2 Interferometer
Light from the source (Fig. 1.2) strikes the beam splitter (B). The beam splitter allows 50% of the radiation to be transmitted to the translatable mirror M1. The other 50% of the radiation is reflected resected to the fixed mirror M2. The compensator plate P is introduced along this path to make each path have the same optical path length when M1 and M2 are the same distance from the beam splitter. After returning from M1, 50% of the light is reflected toward the frosted glass screen. Likewise, 50% of the light returning from M2 is transmitted to the glass screen. At the screen, the two beams are superposed, and one can observe the interference between them.

1.2.3 Interferometry in OCT
Low coherence was initially described by Sir Issac Newton. 29 This principle of interferometry has been used technically in fiber-optic instrumentation in the last two decades. Distance or spatial information may be determined from the time delay of reflected echoes according to the formula ΔT=z/v, where ΔT is the echo delay, z is the distance that the echo travels, and v is the velocity of the sound wave or light wave. 2 Low-coherence interferometry was first applied in ophthalmology to perform precision measurements of axial eye length and corneal thickness. 29 Light from a source is directed onto a beam splitter, and one of the beams is incident onto the sample to be imaged; the second beam travels a reference path with a variable path length and time delay (Fig. 1.3). The backscattered light from the sample is interfered with reflected light from the reference arm and detected with a photo detector at the output of the interferometer. If the light source is coherent, interference fringes will be observed as the relative path lengths are varied. However, if low-coherence or short-pulse light is used, interference of the light reflected from the sample and reference path can occur only when the two path lengths match to within the coherence length of the light. The echo time delay and intensity of backscattered light from sites within the sample can be measured by detecting and demodulating the interference output of the interferometer while scanning the reference path length.

When light enters the eye, it goes to the interior of the eye, hits the ocular structures, and comes back. Thus, the light is back-reflected from tissue boundaries and backscattered with varying intensities from tissues of different optical properties. 2 The distances and dimensions of the intraocular structures are determined from the amount of light backreflected and backscattered from the biological tissue by quantifying the echo time delay. 30 The echo time delay depends on the distance travelled by the light and its velocity. We know that the velocity of light is faster than that of sound; thus, resolution of an image acquired by OCT is higher.
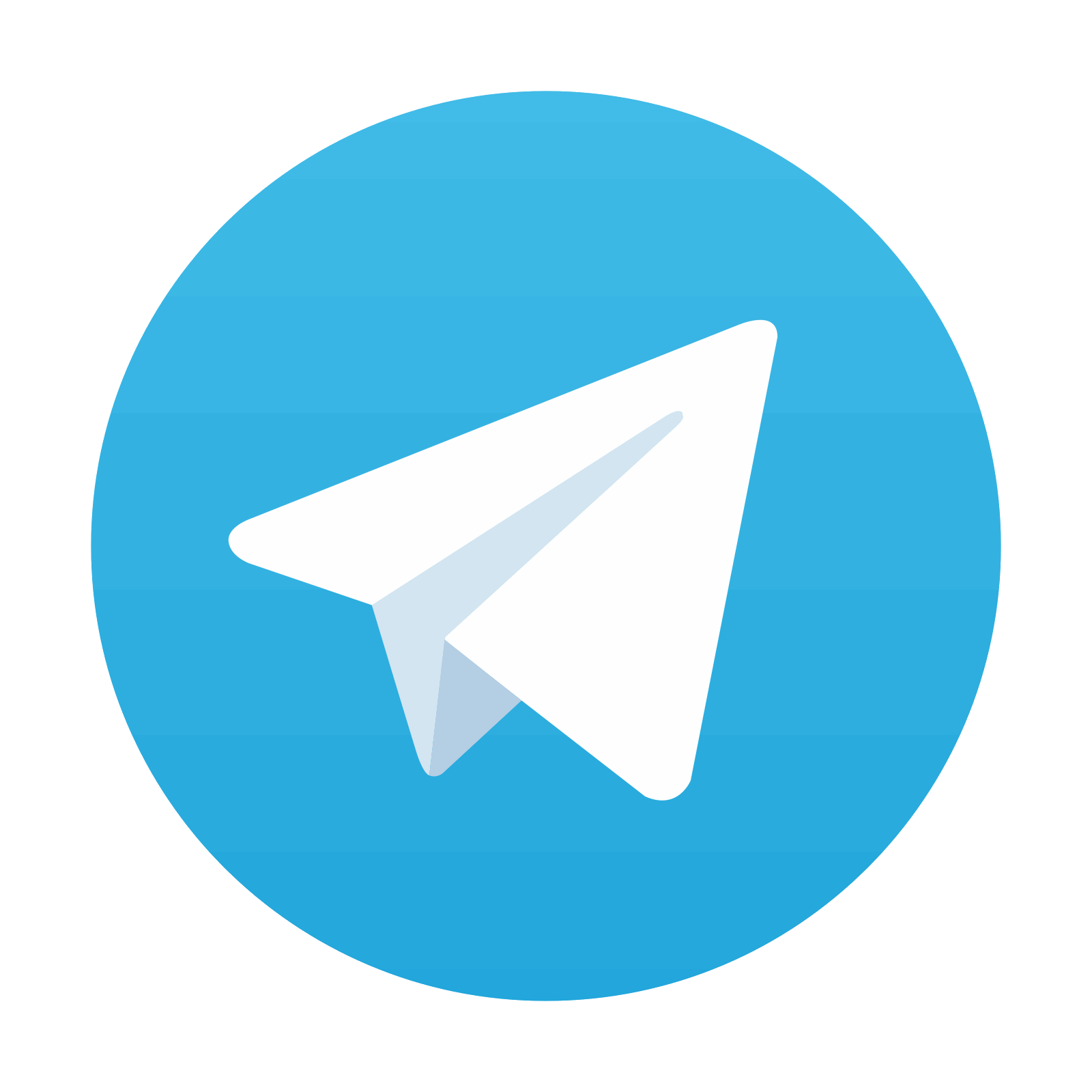
Stay updated, free articles. Join our Telegram channel

Full access? Get Clinical Tree
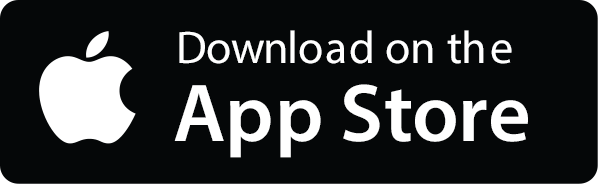
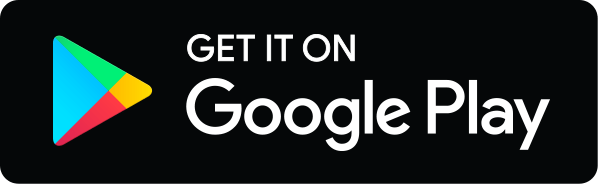
