Traditional upper airway surgery directly modifies skeletal and soft tissue structures surrounding the airway to treat obstructive sleep apnea (OSA). Upper airway stimulation (UAS) attempts to treat upper airway obstruction and OSA by stimulating the hypoglossal nerve. The Inspire II implant has been approved for clinical UAS. Basic science data support that UAS prevents obstruction and improves airflow. Clinical results demonstrate that UAS improves respiratory sleep metrics and improves both objective and subjective self-reported sleep and quality-of-life outcomes. In a substantial number of individuals who meet inclusion criteria, UAS appears to be a viable, long-term, low-morbidity treatment of moderate-to-severe OSA.
Key points
- •
Obstructive sleep apnea (OSA) in adults is often a multifactorial process, with both mechanical and physiologic processes contributing to airway collapse during sleep.
- •
Contraction of the base of tongue provides support and stabilization of the posterior oropharynx through a complex interaction with the palate and oropharyngeal constrictors. This assists in maintaining a patent airway.
- •
Muscle tone during sleep is influenced by the loss of tonic muscle activity to the upper airway with sleep onset, CO 2 -mediated ventilatory instability, and an inadequate activation of phasic genioglossus muscle reflex.
- •
Traditional surgical interventions for OSA address the mechanical (anatomic) contributions to OSA by altering soft tissue. The Inspire II implant is the first surgical intervention to address the physiologic processes that contribute to OSA.
- •
In a multicenter, prospective cohort study, patients were implanted with the Inspire II system and followed for 12 months. Apnea Hypopnea Index (AHI) decreased by 68% after implant, and 63% of patients had a postimplant AHI of less than 15 events per hour.
Introduction
Upper airway stimulation acts to reduce or eliminate upper airway collapse and resultant obstructive sleep apnea (OSA) by augmenting genioglossus muscle tone during sleep. It is unique among surgical interventions in that it does not attempt to reconstruct the structure of the upper airway, but rather augments mechanisms of physiologic compensation to prevent OSA. A novel approach warrants an understanding of these processes.
Introduction
Upper airway stimulation acts to reduce or eliminate upper airway collapse and resultant obstructive sleep apnea (OSA) by augmenting genioglossus muscle tone during sleep. It is unique among surgical interventions in that it does not attempt to reconstruct the structure of the upper airway, but rather augments mechanisms of physiologic compensation to prevent OSA. A novel approach warrants an understanding of these processes.
Mechanisms of upper airway collapse and physiology obstructive sleep apnea
Overview
Airway collapse in OSA is the result of a complex interaction of both upper airway structural characteristics and ventilatory physiology during sleep. It is an imbalance between a structurally smaller (and therefore at-risk) upper airway and physiologic mechanisms that sustain breathing during sleep. The contribution of these 2 processes is highly variable between individuals and results in vast differences in the underlying physiologic disturbance responsible for an individual’s OSA. But the consequence of this imbalance is an upper airway that is unable to maintain adequate ventilation to sustain life.
Anatomic Considerations
Multiple anatomic features contribute to the abnormal upper airway in OSA in adults. It is the common scenario that when combined they all contribute to a small upper airway, which when exposed to the stress of sleep onset and ventilatory (loop gain) instability leads to obstruction. Multiple structural phenotypes may exist that contribute to the risk of OSA.
Tongue anatomy
The tongue is a vital muscle that is responsible for a variety of functions, from speech and mastication, to maintenance of a patent airway and pharyngeal stability. The anterior tongue is responsible for speech and mastication, while the posterior tongue is responsible for maintaining pharyngeal stability. In people, the posterior tongue anatomically is not in the oral cavity but is the anterior wall of the pharynx. The differences in these functions are highlighted by distinct differences in both the anatomy and physiology of the anterior and posterior tongue musculature.
The human tongue is comprised of 8 pairs of skeletal muscles: 4 paired extrinsic muscles (with a bony attachment to anchor the tongue and movements) and 4 paired intrinsic muscles, with no bony attachments. Extrinsic muscle fibers originate from external bony attachments and terminate in the tongue, whereas intrinsic muscles both originate and terminate within the tongue. As a general rule, whole-tongue movements are attributed to extrinsic muscle function, whereas lingual-shaped changes are attributed to intrinsic musculature. The posterior tongue muscles are significantly more fatigue resistant than those of the anterior fibers because of a higher percentage of slow-twitch type I fibers. This is similar to the fatigue-resistant composition of cardiac and diaphragmatic muscle fiber composition, which are designed to withstand fatigue and constant, sustained activity. The high ratio of fatigue-resistant fibers is critical for functions requiring sustained, tonic contractions, as is seen in swallowing and maintaining airway stability.
Tongue-palate-oropharynx interaction
The anatomic and functional relationships between the tongue base and the superior pharyngeal constrictor and the tongue base and palate are key to understanding the potential benefit in using hypoglossal nerve stimulation to improve airway stability. The central third (the anterior wall) of the oropharynx is formed by the posterior aspect of the tongue. The superior pharyngeal constrictor muscle forms the muscular ring of the hypopharynx. Studies have shown that movements of the tongue create a forward mechanical drag on the superior constrictor muscle, which makes the pharyngeal wall stiffer and less compliant, therefore less prone to collapse. The stability of the oropharynx is not only dependent on the stiffness of the constrictor muscles themselves, but also in part depends on the stiffness of the posterior lingual muscles, although some of these are known tongue retractors. A similar, but less well understood relationship exists between the palate and the tongue. Velopharyngeal patency may be mediated by the palatoglossal arch, direct compressive effects of the tongue, or oropharyngeal surface interactions. Contractions of the tongue musculature, regardless of whether they are protrusive or retrusive functions, potentially stabilize and stiffen the palatoglossal fold by applying mechanical drag. In 2003, Isono and colleagues examined the dynamic interaction between the posterior tongue and the soft palate in patients with sleep apnea. It was noted that a close apposition between the tongue and soft palate (the tongue–soft palate interface) occurred with variable magnitude in all patients with sleep-disordered breathing, and that there was a progressive increase in contact pressure between the tongue and soft palate during obstructive apneic events. This contributed to maintaining retropalatal closure. Release of this vector of force would assist in opening the palate in some patients. Finally, surface tension forces interact to contribute to upper airway collapse and reopening. Forward movements of the tongue may displace the palate, which is adherent by surface tension forces.
Physiologic Considerations
No single physiologic process contributes to OSA. A major contributor to the transmural or stabilizing pressure of the upper airway is upper airway muscle tone. Muscle tone during sleep is influenced by: (1) the loss of tonic muscle activity to the upper airway with sleep onset, (2) loss of upper airway muscle tone due to CO 2 -mediated ventilatory instability, and (3) an inadequate activation of phasic genioglossus muscle reflex. All are ultimately mediated by activity of the hypoglossal nerve (CN XII).
Historically, abnormal timing of the activation of the diaphragm and upper airway muscles was considered a major physiologic factor in OSA. The early onset of diaphragm contraction, which exposed the relatively passive upper airway to significant negative inspiratory forces, could potentially obstruct the airway. An earlier preactivation of the genioglossus muscle could preempt this and prevent obstruction.
Although asynchronous timing of upper and lower airway muscles does occur in sleep, it is more likely that the partial or complete loss of compensatory upper airway muscle tone is the major contributor to sleep apnea. This includes the sleep-related loss of the negative pressure-mediated genioglossus muscle reflex, the progressive loss of tonic muscle tone at sleep onset and with various sleep stages, and the massive changes in muscle tone (zenith and nadir) that occur due to the CO 2 -mediated changes in ventilatory drive during nonrapid eye movement (non-REM) sleep. Upper airway stimulation (UAS) interacts with all these to reduce the propensity of the upper airway to obstruct.
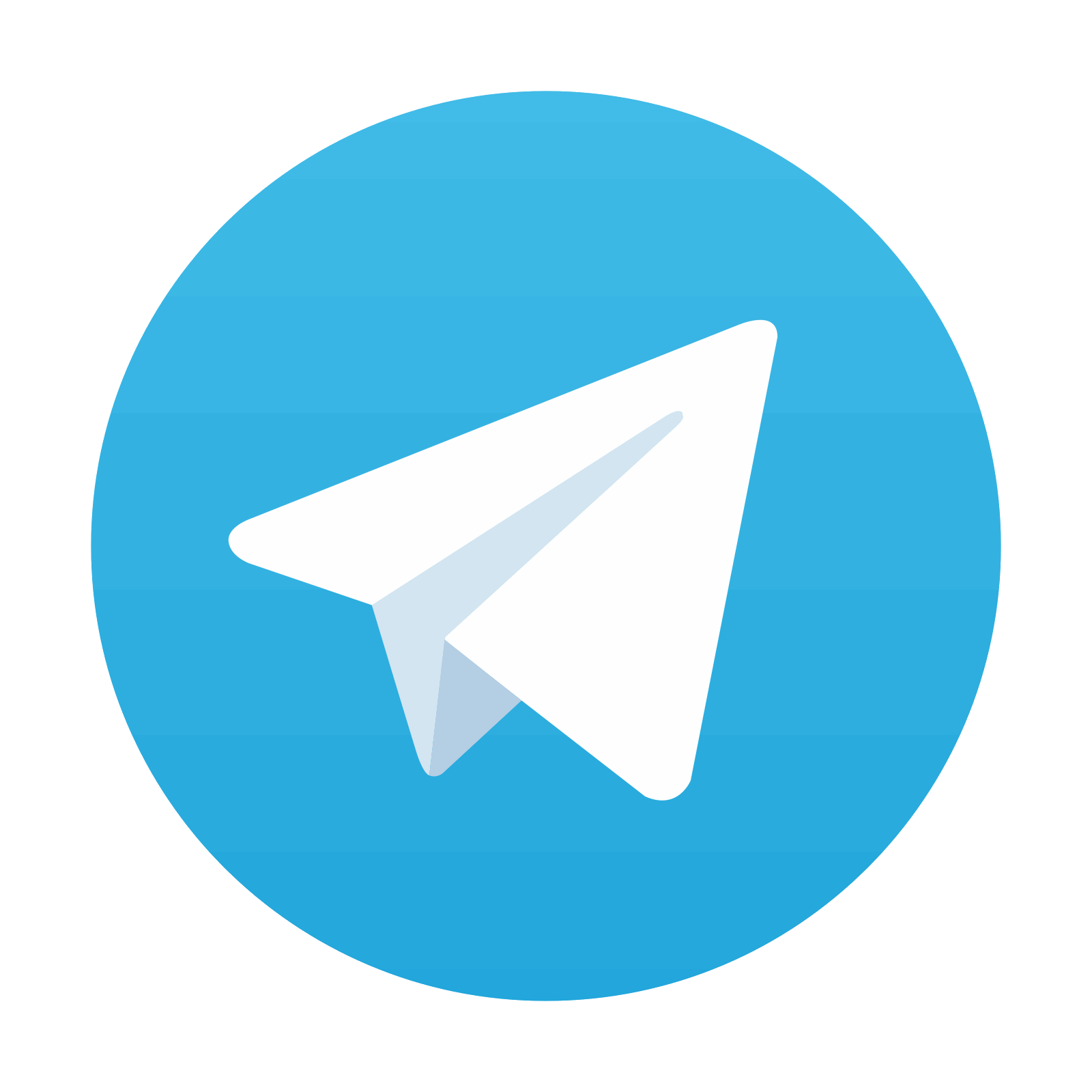
Stay updated, free articles. Join our Telegram channel

Full access? Get Clinical Tree
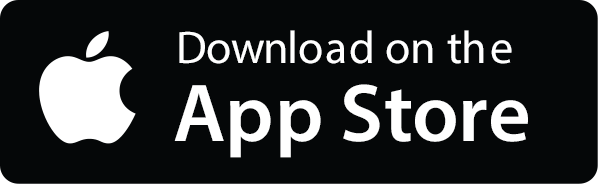
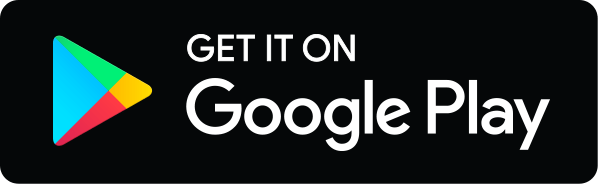