1
Introduction
Upper airway stimulation is a novel approach to the management of obstructive sleep apnea (OSA). Hypoglossal nerve stimulation (HNS) therapy has been shown to improve objective respiratory and subjective quality-of-life outcomes and is well positioned as a new second-line treatment for moderate to severe OSA.
2
Scientific Rationale
The development of HNS therapy has been driven by a need for new OSA treatments, as well as growing recognition of neuromuscular dysfunction as a contributing factor to OSA pathophysiology. Although first-line therapy of continuous positive airway pressure (CPAP) has the most evidence for effectively managing OSA and its comorbid cardiovascular risks, adherence rates are suboptimal. Two recent multicenter studies demonstrated that only 39% to 50% of participants met the minimum CPAP adherence criteria. Alternative treatments to CPAP, including oral appliance therapy, positional therapy, behavioral modifications, weight loss, and upper airway reconstructive surgery, have been shown to be effective in appropriately selected patients, but treatment response can be incomplete, and many patients often require additional therapy.
In addition to the structural upper airway vulnerability of OSA patients, increasing evidence indicates that the changes in the neuromuscular control of breathing during sleep also play a key role in the pathophysiology of OSA. A simplified model of the upper airway neuromuscular reflex has been described as an afferent pathway from the pharynx through the superior laryngeal nerve to the nucleus solitaris and an efferent pathway from the hypoglossal motor nucleus through the hypoglossal nerve to the genioglossus muscle, which is the primary upper airway dilator. Normal healthy subjects have been shown to respond to negative intraluminal pressure with a corresponding increase in genioglossus electromyographic (EMG) activity. The neuromuscular feedback loop is thought to be dysfunctional in patients with OSA. Recent studies have demonstrated abnormal genioglossus EMG patterns resembling that of denervation-renervation injuries, as well as delayed distal hypoglossal nerve latency and low motor amplitude in patients with OSA compared with normative data. Although the etiology of the defective negative pressure reflex remains unclear and is likely multifactorial, augmenting the efferent limb through electrical stimulation of the hypoglossal nerve has emerged as a promising new approach to the management of OSA.
The feasibility of electrical stimulation of the hypoglossal nerve was originally established through multiple animal studies, which demonstrated increases in airflow and airway stability in response to neuromuscular augmentation. Human studies subsequently corroborated these findings, demonstrating that neurostimulation was not only effective in improving airflow and airway stability, but also well tolerated and without adverse sequelae such as arousals, sleep fragmentation, or neuromuscular side effects. Key studies by Oliven et al. showed that selective stimulation of the genioglossus muscle (tongue protrusor) improved airflow and airway stability, whereas selective stimulation of the styloglossus and hyoglossus (tongue retrusors) increased collapsibility of the airway. Coactivation of both protrusor and retrusor muscles produced a net improvement in airflow and reduction in critical closing pressure, confirming the genioglossus as the dominant upper airway muscle. These observations led to advanced analysis of the terminal branches of the hypoglossal neuroanatomy and the use of selective hypoglossal nerve branch stimulation in the development of the first commercially available implantable neurostimulation devices. Furthermore, the use of nasopharyngoscopy demonstrated that selective neurostimulation resulted in an enlargement and stabilization of not only the retrolingual portion of the airway (130% increase), but also the retropalatal space (180%). This finding was one of the first indications that single-modality HNS therapy could provide multilevel upper airway treatment in contrast to many other traditional site-specific pharyngeal surgical procedures ( Fig. 48.1 ).

3
Clinical Trials
After the first human pilot study in 2001, multiple investigators and medical device companies spent a decade improving upon the technology and studying their devices in larger trials. Eastwood et al. (n = 21) and Kezirian et al. (n = 31) studied the Hypoglossal Nerve Stimulation System and showed significantly reduced mean Apnea/Hypopnea Index (AHI) at 6 months and 12 months, respectively, with reported adverse events including a combined total of two infections requiring device removal and three cuff electrode dislodgements. Mwenge et al. (n = 14) studied the Aura6000 System, which consists of a cuff electrode that is continuously activated and therefore does not require a respiratory sensor, and demonstrated a significant improvement in mean AHI at 12 months, with reported adverse events including two participants with transient tongue paresis.
Van de Heyning et al. studied the Inspire II UAS System in an initial feasibility study that identified AHI <50, body mass index (BMI) ≤32, and absence of complete concentric retropalatal collapse on drug-induced sleep endoscopy (DISE) as predictors of response to therapy. Subsequent prospective enrollment of patients based on these inclusion criteria demonstrated a significant AHI reduction at 6 months and built the groundwork for the largest multicenter prospective trial to date: the Stimulation Therapy for Apnea Reduction (STAR) trial. One hundred and twenty-six participants who met the criteria of (1) moderate to severe OSA (AHI 20–50) with CPAP intolerance, (2) BMI ≤32, and (3) absence of a complete circumferential retropalatal collapse on DISE underwent implantation and were followed at 12-, 24-, and 36-month intervals.
Primary outcome measures (AHI, 4% oxygen desaturation index) and secondary outcomes measures (Epworth Sleepiness Scale [ESS], Functional Outcomes of Sleep Questionnaire [FOSQ]) all demonstrated statistically significant improvements at 12 months. There was no permanent hypoglossal nerve weakness, no serious device-related infection requiring explantation, and significantly less postoperative discomfort compared with traditional upper airway surgeries. The most common adverse therapy-related side effect was tongue discomfort due to stimulation itself or abrasion of the tongue from an adjacent tooth. Nightly adherence by self-report was 86% at the 12-month mark. Reported data at the 24-month mark showed continued significant improvement in ESS and FOSQ scores, and data at the 3-year mark supported sustained long-term improvements in AHI as well as ESS and FOSQ scores. After Food and Drug Administration (FDA) approval of the Inspire II system in 2014, the first report of HNS therapy outcomes in a cohort from routine clinical practice, outside of a clinical trial setting, demonstrated few adverse events (which were minor), high therapy adherence rates based on device download, and objective OSA outcomes that even exceeded previously published trial results.
4
Patient Selection
All patients who are being considered for HNS therapy should undergo a comprehensive sleep medicine history and upper airway evaluation. HNS therapy is still considered second-line therapy for those patients with moderate to severe OSA who are intolerant or unable to achieve adequate adherence with positive pressure therapy. Current published screening criteria also include BMI ≤32 and absence of complete concentric pattern of retropalatal collapse on DISE. It is important to recognize, however, that these screening criteria are an oversimplification of the patient selection process and must be interpreted within the broader clinical context of the patient’s sleep medicine history, comorbid medical conditions, and OSA pathophysiology, as well as their specific nasal, pharyngeal, and skeletal anatomic phenotype.
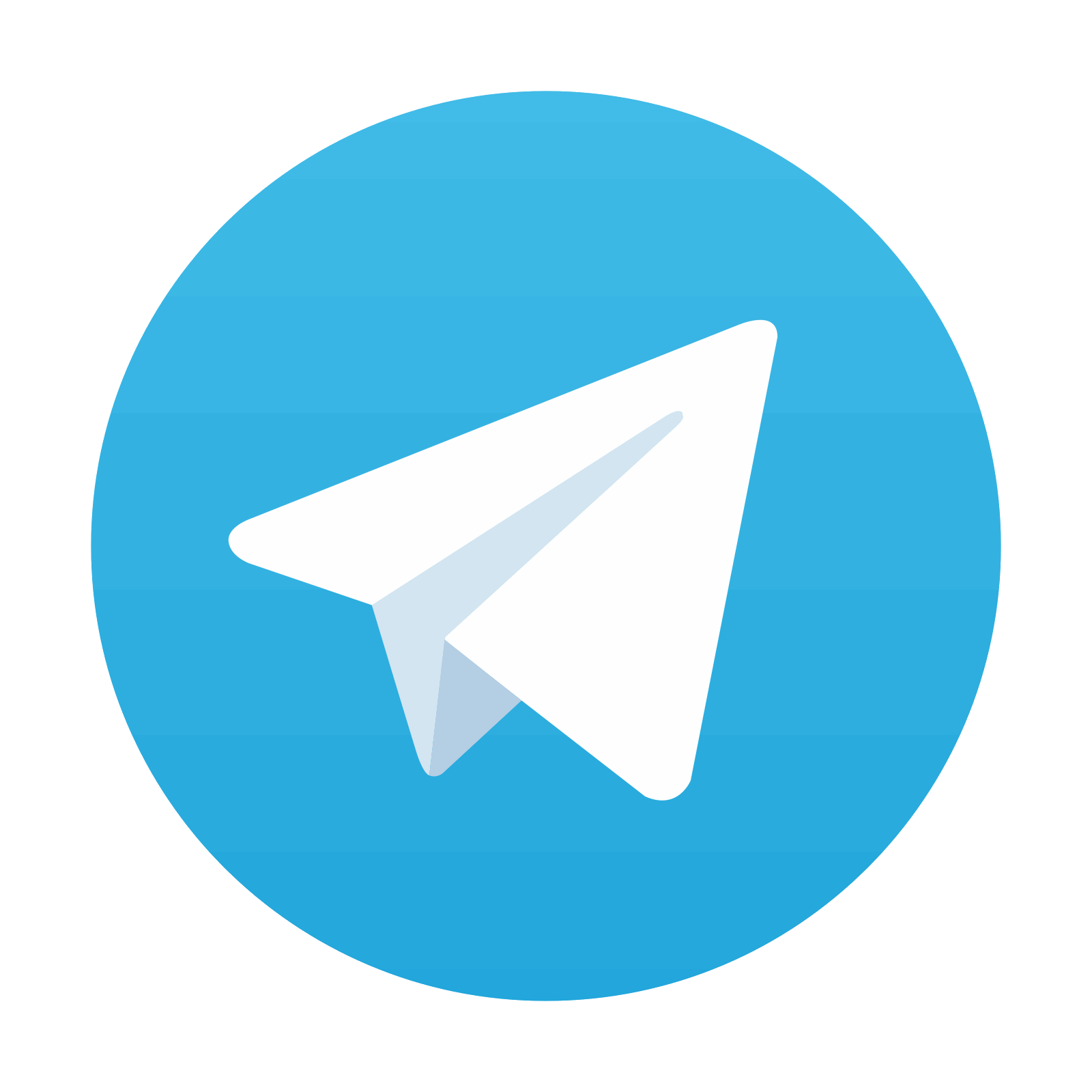
Stay updated, free articles. Join our Telegram channel

Full access? Get Clinical Tree
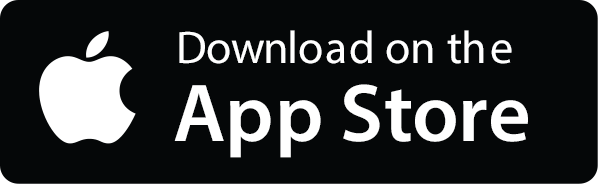
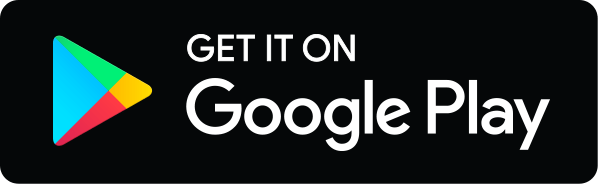