Purpose
To evaluate possible changes in the collagen and elastic components of the subepithelial connective tissue of human pterygium.
Design
Immunohistochemical study.
Methods
Immunohistochemical staining using antitropoelastin, anti-fibulin-2, and anti-fibulin-3 antibodies was performed in 10 normal conjunctival and 20 pterygium specimens. Masson trichome staining also was performed to study subepithelial connective tissue. Sirius red staining was used to identify collagen type I and III components. Tropoelastin, fibulin-2, and fibulin-3 messenger ribonucleic acid (mRNA) expressions were analyzed in 9 conjunctival and 12 pterygium specimens by quantitative real-time polymerase chain reaction assay.
Results
The subepithelial connective tissue and vessels were more predominant in pterygium compared with the normal conjunctival tissue. Amorphous subepithelial zones were observed in the areas of the pterygium tissue, but not in normal conjunctiva. Increased tropoelastin staining was seen in the pterygium tissue with areas of degenerative changes or immature formation of elastic fibers, as well an increase in tropoelastin mRNA, in contrast with fibulin-2 and fibulin-3 messenger levels. Fibulin-2 and fibulin-3 expression was colocalized in the subepithelial connective tissue and was distributed along blood and lymphatic vessels. Collagen type III, an immature form of collagen, was increased in the pathologic samples in association with a tissue remodeling process.
Conclusions
Elastin metabolism is dysregulated in the pathogenesis of human pterygium with tropoelastin, fibulin-2, and fibulin-3 overexpression in the subepithelial connective tissue.
A pterygium is a triangular growth of fibrovascular tissue extending from the bulbar conjunctiva onto the cornea. Pterygium is more prevalent in patients living in regions closer to the equator. Histologically, pterygium is characterized by an atrophic conjunctival epithelium and a highly vascularized mass of hypertrophic and elastotic degenerated connective tissue. The extent and severity of this fibrovascular growth is a reliable morphologic index for predicting pterygium recurrence after excision.
The pathogenesis of pterygium is uncertain. Many environmental factors such as ultraviolet (UV) irradiation, chronic irritation, and inflammation have been postulated to be causes. Chronic exposure to UV light is the main predisposing environmental factor for pterygium formation. Limbal stem cell deficiency resulting from chronic UV light exposure may result in concomitant breakdown of the limbal barrier and subsequent corneal conjunctivalization.
Elastodysplasia and elastodystrophy are 2 known manifestations present in the subepithelial connective tissue of the conjunctival portion of pterygium. Pterygium may result from newly synthesized elastic fiber precursors and abnormal maturational forms of elastic fibers (elastodysplasia) that undergo secondary degeneration (elastodystrophy). These structures are presumed to be formed by actinically damaged fibroblasts of the conjunctival substantia propria. Immunohistochemical studies confirmed that these fibers are elastic and do not result from elastotic degeneration of collagen fibers. Similar pathologic accumulation of abnormal elastic fibers is found in chronically photodamaged skin ; therefore, it is reasonable to associate the formation of elastoid degeneration in pterygium with UV irradiation.
Collagen and elastin, the major components of the extracellular matrix, are intrinsic indicators of physiologic and pathological states. The elastic function complements collagen fibrils, which impart tensile strength. However, to understand the healthy and diseased tissues, an investigation of the arrangement and modification of the major structural proteins, such as collagen and elastin, is crucial.
The elastic fibers are formed through the reticulation of an amorphous polymer composed of the protein elastin, known as tropoelastin, over a framework of fibrillin-rich microfibrils, and latent transforming growth factor-β–binding proteins. The fibulins are a family of proteins that are associated with basement membranes and elastic extracellular matrix fibers. Substantial evidence has implicated the fibulins in both elastic matrix fiber assembly and function ( Figure 1 ). Fibulin-1, fibulin-2, and fibulin-5 bind to tropoelastin. Unlike fibulin-1, fibulin-2 is found at the interface between the microfibrils and the elastin core. The ability of fibulin-2 to bind elastin and fibrillin-1 may indicate that fibulin-2 anchors fibrillin-containing microfibrils to elastin fibers. Fibulin-3 interacts with another basement membrane protein, extracellular matrix protein 1, and it also interacts with elastin monomer tropoelastin. These interactions likely contribute to the integrity of basement membrane zones and anchor other extracellular matrix structures such as elastic fibers to basement membranes.
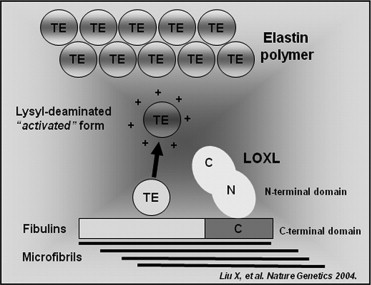
Several recent findings have indicated that fibulins are involved in human inherited ocular disorders like age-related macular degeneration and macular dystrophies. However, they have never been tested in the pterygium pathogenesis. Therefore, this study focused on evaluating the expression of collagen and elastic components in human pterygium.
Methods
Patients
Surgical specimens of primary pterygia and normal conjunctiva from age-matched cataract patients were obtained, according to the Ethics Committee of the University Hospital Principe de Asturias and conforming to the tenets of the Declaration of Helsinki of 1975. Written informed consent was obtained from all patients.
The following groups were established: for the immunohistochemical studies, control group (n = 10) specimens were obtained from normal conjunctiva from subjects with a mean age ± standard deviation (SD) of 53.2 ± 5.2 years, and pterygium group (n = 20) specimens were obtained from active pterygium from patients with a mean age ± SD of 49.3 ± 3.2 years. For quantitative real-time polymerase chain reaction assays (PCR), control group (n = 9) specimens had a mean age ± SD of 47.3 ± 14.6 years and pterygium group (n = 12) specimens had a ± SD of 47.3 ± 12.9 years. Immediately after harvesting the tissue samples, the conjunctival parts of pterygia specimens were placed in sterile minimal essential culture medium and transferred at 4 C to the laboratory.
Light Microscopy
Tissue specimens were fixed in Bouin solution or paraformaldehyde 4%, dehydrated, embedded in paraffin, cut into 5-μm serial sections using a rotating microtome (Microm HM-325; MICROM International GmbH, Walldorf, Germany), deparaffinized, and rehydrated. These sections were used in the morphologic and immunohistochemical analyses.
Sections for histologic examination were stained using different techniques to examine the different components of the subepithelial connective tissue: hematoxylin and eosin, Masson trichrome (Goldner-Gabe variant), orcein, and Sirius red before observation under a light microscope (Zeiss Axiophot, Jena, Germany).
Sirius red was used to localize and assess the maturity of the collagen component of the subepithelial connective tissue from the normal conjunctival and pterygium specimens. This technique is based on the orientation and interaction between the sulphone groups of the dye and the amine groups of lysin and hydrolysin and guanidine groups of arginine in the collagen fibers; the colors differ depending on the degree of collagen maturity. Collagen type I (mature collagen) stains reddish-orange, and type III collagen (immature collagen) has a yellow-green hue. After staining, the tissue sections were examined by polarized light microscopy. Ten digitalized histologic images of Sirius red staining per patient were obtained using a digital camera fitted to the microscope and were analyzed using image analysis software (Axiovision AC 4.1; Carl Zeiss, Jena, Germany) to obtain staining intensity percentages.
Immunohistochemistry
The sections were deparaffinized, hydrated, and equilibrated in phosphate-buffered saline (pH 7.4). A rabbit polyclonal antitropoelastin antibody (1:500; donated by Dr Mecham) and rabbit monoclonal anti-fibulin-2 and anti-fibulin-3 antibodies (1:200; donated by Dr Sasaki) were used as primary antibodies. The antigen–antibody reaction was detected by alkaline phosphatase or peroxidase-labeled avidin–biotin procedures. The chromogenic substrate contained α-napthol and fast-red or diaminobenzidine. Nuclei were counterstained with Carazzi hematoxylin. After immunostaining, the tissue sections were examined by light microscopy. Using a computerized Microm image analyzer (MICROM International GmbH) on the stained sections, the labeling percentages were obtained for the different antibodies. For the quantitative analysis, 10 tissue sections per patient immunolabeled using each antibody were examined. A negative control of the technique was performed without the primary antibody.
Ribonucleic Acid Extracted and Quantitative Real-Time Polymerase Chain Reaction
Total ribonucleic acid (RNA) isolated using Trizol (Invitrogen, Carlsbad, California, USA) and complementary deoxyribonucleic acid synthesis by reverse transcription with M-MLV reverse-transcriptase enzyme (Invitrogen) were carried out as described previously. To detect genomic deoxyribonucleic acid contamination, another reverse transcription was carried out without enzyme. To quantify messenger RNA (mRNA) expression, quantitative real-time PCR was performed with iQ SYBR Green Supermix following the manufacturer’s instructions (Bio-Rad Laboratoires, Hercules, California, USA), in a StepOnePlus real-time PCR system (Applied Biosystems, Foster City, California, USA). For quantification, we performed a standard curve experiment on the instrument. Negative control with UltraPure DNase/RNase-free distilled water (Invitrogen) was added in each reaction. The specific human primers amplified are the following: tropoelastin, 5′-CGA ACT TTG CTG CTG CTT TAG-3′ (sense) and 5′-GTG TAT ACC CAG GTG GCG TG-3′ (antisense); fibulin-2, 5′-CTC AGC CAT ATG CTC CTG TTT-3′ (sense) and 5′-GAT GTC CAC ACA GTT GCC TTC-3′ (antisense); fibulin-3, 5′-CAG GCT ACG AGC AAA GTG AAC-3′ (sense) and 5′-ACA GTT GAG CCT GTC ACT GCT-3′ (antisense); and glyceraldehyde 3-phosphate-dehydrogenase, 5′-GGA AGG TGA AGG TCG GAG TCA-3′ (sense) and 5′-GTC ATT GAT GGC AAC AAT ATC CAC T-3′ (antisense). The thermal cycling conditions were: an initial stage at 95 C for 10 minutes, followed by 40 cycles of 95 C for 15 seconds, 60 C (each primer) and 64 C (tropoelastin primers) for 30 seconds, and 72 C for 1 minute. Products were submitted to 2% agarose gel electrophoresis and were visualized with UV light. Gene expression was normalized against the expression of the constitutive gene glyceraldehyde 3-phosphate-dehydrogenase.
Statistical Analysis
All data were expressed as mean ± standard deviation (SD). Data analysis was performed using the Graph Pad Prism 4 package (GraphPad Software Inc., La Jolla, California, USA). Image analysis data were subjected to descriptive statistical analysis. Mean data were compared among the groups using the Mann–Whitney U test. The level of significance was set at P < .05.
Results
Light Microscopy
Staining of the different samples showed that subepithelial connective tissue generally was more predominant in the pathologic tissue than in the normal conjunctiva. In these specimens, angiogenesis was more evident than in the control group and showed a large number of vessels in the subepithelial tissue ( Figure 2 ). Subepithelial zones with amorphous and fibrillar material were not observed in normal conjunctiva ( Figure 2 , Top), but were observed in the areas of the pterygium tissue ( Figure 2 , Bottom). These amorphous or fibrillar zones failed to stain with Sirius red, indicating that these structures were not collagen fibers. The collagen fibers surrounding these amorphous areas were associated with some isolated fibers located within the structures ( Figure 3 , Right).
The Sirius red staining in healthy specimens showed that collagen types I and III were present in similar proportions ( Table and Figure 3 , Top left). Sirius red staining in the pterygium samples showed that collagen types I and III were presented in the subepithelial areas; however, in these pathologic specimens, the immature form of collagen (collagen III) increased compared with the normal conjunctival specimens ( P = .032) and showed a tissue remodeling process ( Table and Figure 3 , Top right).
Staining | Control Tissue | Pterygium Tissue |
---|---|---|
Tropoelastin | 9.5 ± 3.1 a | 30.3 ± 5.4 |
Fibulin-2 | 11.6 ± 4.2 a | 22.1 ± 2.5 |
Fibulin-3 | 15.8 ± 2.9 a | 34.1 ± 6.3 |
Collagen I | 16.9 ± 5.3 | 14.3 ± 7.6 |
Collagen III | 12.2 ± 3.2 a | 26.8 ± 5.7 |
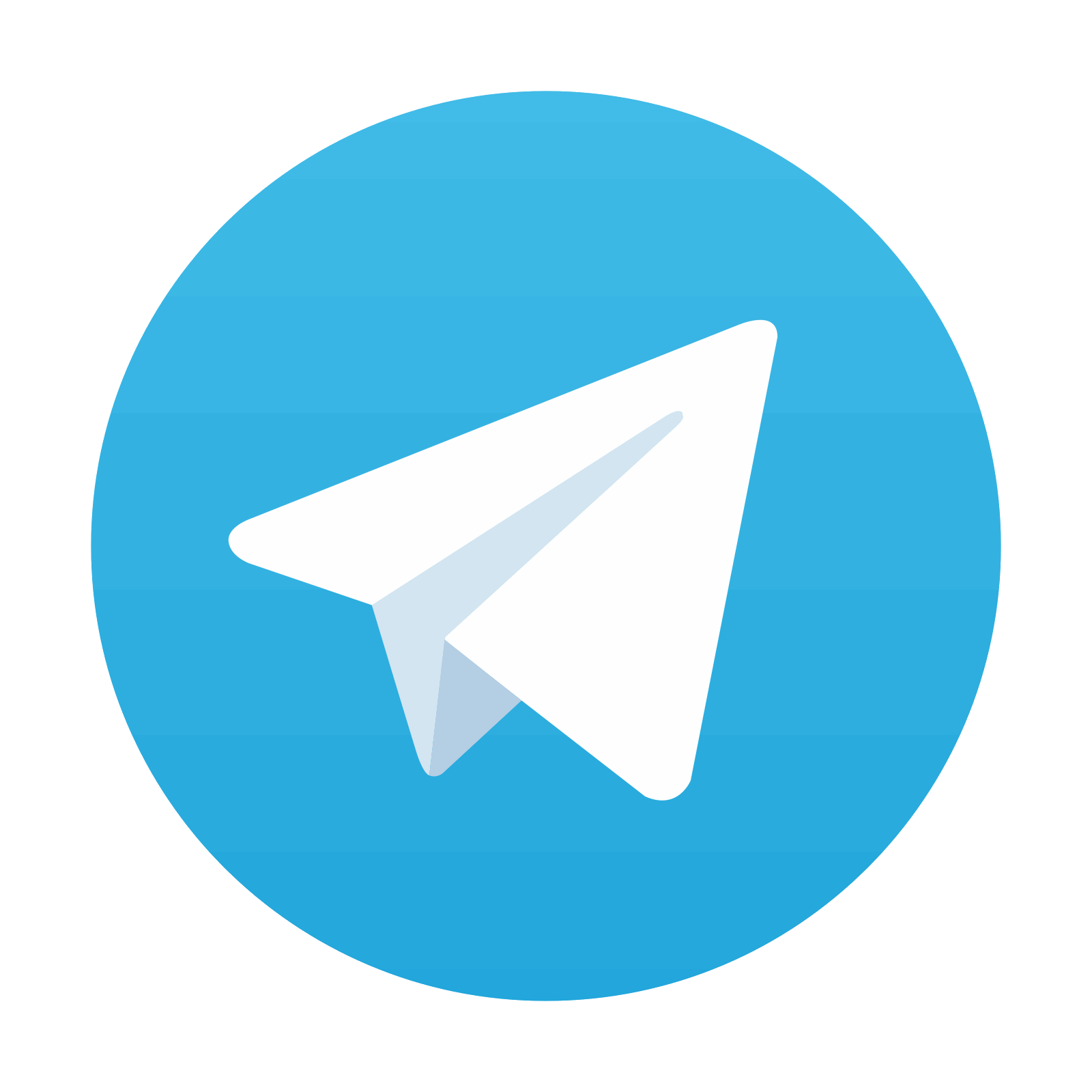
Stay updated, free articles. Join our Telegram channel

Full access? Get Clinical Tree
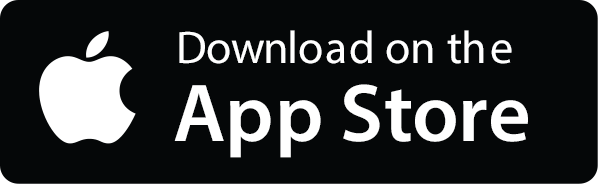
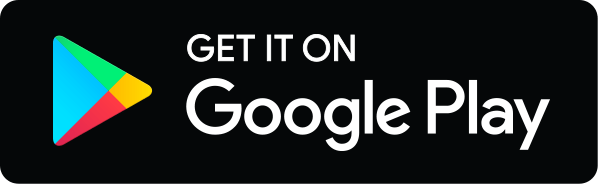