Traumatic Optic Neuropathy: An Evidence-Based Perspective on Management
Harmeet S. Gill MD, FRCSC
Robert A. Kersten MD, FACS
Introduction
Approach to Traumatic Visual Loss
Patients can undergo visual loss after a traumatic insult to the eye or periorbital structures. First and foremost, we recommend establishing whether the injury falls into one or more of the following categories in order to guide management.
Globe injury. (Fig. 18.1A)
The management is primary repair of involved structures.
Optic nerve dysfunction. *look for decreased visual acuity and afferent pupillary defect
Orbital compartment syndrome. Signs of elevated orbital pressure include proptosis, high intraocular pressure, decreased eye motility, and decreased retropulsion (“tight” orbit). This may occur secondary to hemorrhage, edema, or air within the orbital space. The two main mechanisms of injury to the optic nerve are ischemic compartment syndrome (when orbital pressure exceeds mean arterial pressure) and mechanical optic nerve “stretch” from globe proptosis (Fig. 18.1B).
The management is immediate lateral canthotomy and cantholysis.
2. Direct optic nerve injury. Secondary to bone fragment or foreign body. (Fig. 18.1C).
3. Indirect optic neuropathy. Little anatomical disruption of orbital structures.
The purpose of this chapter is to provide guidelines regarding management for direct and indirect traumatic optic neuropathy.
Demographics
Any optic nerve dysfunction secondary to trauma is referred to as traumatic optic neuropathy (TON). This is an uncommon but severe cause of visual loss that can follow blunt or penetrating injury to the orbital or craniofacial structures. Most patients are young males in their early thirties.1 Common causes include high-speed vehicle collisions, falls, and physical assault with various weapons.2 Up to 20% of ocular combat injuries are associated with TON.3 The incidence varies between 0.5% and 5% after closed head injury, and rates are higher in patients with concomitant zygomaticomaxillary complex (ZMC) and other facial fractures.4,5,6 The prevalence of TON is about one in one million persons.2
Clinical Features
The majority of patients with TON will suffer immediate visual decline while up to 10% report delayed or progressive loss of vision.1,7 Visual deficit can present as decreased acuity, color vision, or variable field defects. Most patients will have 20/400 or worse acuity,8 and up to one-third may present with no perception of light.2 However, patients may be unconscious or unresponsive in the setting of severe head trauma precluding assessment of visual acuity. The presence of an afferent pupillary defect and otherwise normal ophthalmic examination is suggestive of TON. Pupillary responses can be difficult to assess in the presence of analgesic opioids causing miosis. It is imperative that reversible causes of vision loss (i.e., retinal
detachment, retrobulbar hemorrhage) and neurologic causes of a nonreactive, mydriatic pupil are ruled out. Visual evoked potential testing (pattern or flash) has been studied as a surrogate for visual acuity in TON patients but is often unrecordable and of limited benefit.9,10 Poor prognosticators for visual recovery include no light perception (NLP) at presentation11 and an afferent pupillary defect greater than 2.1 log units.12 Patients with loss of consciousness or lack of visual improvement after 48 hours also tend to have a poor visual prognosis. In the acute setting, the appearance of the optic nerve will vary depending on the anatomical site of involvement but after 4 to 6 weeks demonstrates signs of atrophy and axonal loss (Fig. 18.2).4 Reduced retinal nerve fiber layer thickness can be detected at this time using optical coherence tomography.13,14
detachment, retrobulbar hemorrhage) and neurologic causes of a nonreactive, mydriatic pupil are ruled out. Visual evoked potential testing (pattern or flash) has been studied as a surrogate for visual acuity in TON patients but is often unrecordable and of limited benefit.9,10 Poor prognosticators for visual recovery include no light perception (NLP) at presentation11 and an afferent pupillary defect greater than 2.1 log units.12 Patients with loss of consciousness or lack of visual improvement after 48 hours also tend to have a poor visual prognosis. In the acute setting, the appearance of the optic nerve will vary depending on the anatomical site of involvement but after 4 to 6 weeks demonstrates signs of atrophy and axonal loss (Fig. 18.2).4 Reduced retinal nerve fiber layer thickness can be detected at this time using optical coherence tomography.13,14
Mechanisms of Injury
The mode of injury (direct versus indirect trauma) is often used to classify TON. Direct TON results from a penetrating orbital foreign body or bone fragment that transects or impinges on the optic nerve (Fig. 18.1C). These patients usually suffer immediate, severe, and irreversible loss of vision. In the absence of obvious anatomical disruption, more subtle injury to the optic nerve microvasculature and/or shearing injury to axons occurs.15,16 This is called indirect TON, which typically results from blunt trauma to the frontal bone at the supraorbital rim with transmission of force across the orbital roof concentrated at the orbital apex and optic canal combined with deceleration injuries. An example is a patient hitting his or her head on the pavement after a fall. Most clinical studies focus on the management of indirect TON, which is much more common and typically portends a better visual prognosis than direct TON. Other mechanisms causing optic nerve axonal loss, common to both direct and indirect trauma, include avulsion, compression, swelling, ischemia, and concussion. A computed tomographic (CT) scan with 1 mm cuts of axial and coronal planes of the orbit (and/or head, facial bones) is helpful in the setting of orbitofacial trauma and can help differentiate direct versus indirect TON. The optic canal is formed by the sphenoid bone, which can be fractured in up to 50% to 80% of TON cases.17 The presence of blood in the adjacent sphenoid sinus raises the clinical suspicion of an optic canal fracture. The falciform anterior clinoid process on an axial CT series is a useful landmark to help identify the optic canal.
Optic Nerve Anatomy
Knowledge of the anatomy and course of the optic nerve and its relationship with other orbitocranial structures is necessary
to understand why TON occurs and how it is treated. The optic nerve can be separated into anterior (optic nerve head) and posterior (intraorbital, intracanalicular, and intracranial) segments. Injury to the anterior optic nerve or its blood supply may occur at its point of insertion into the eye after forceful globe rotation. With complete or partial avulsion of the nerve from the globe, an intraocular hemorrhage at the nerve head is seen during funduscopic examination (Fig. 18.3). Injury to the nerve anterior to the central retinal artery entry point will disrupt retinal circulation causing occlusive retinopathy or retinal hemorrhages. More posterior injury is, however, much more common and the optic nerve and fundus will appear normal. The disruptive forces following closed head injury are concentrated at the orbital apex and optic canal, making the intracanalicular optic nerve the most vulnerable segment. The dural sheath that surrounds this segment is tightly adherent to the periosteum within the optic canal. Axonal loss may result from the primary insult of bony impingement following an optic canal fracture, shearing injury, or disruption of nerve microvasculature. A secondary insult then follows. Optic nerve swelling within the canal produces a localized compartment syndrome that precipitates ischemic damage and further axonal loss.7 The intracranial optic nerve segment lies near the falciform dural fold and is perhaps the second most common site of injury. This segment is more commonly implicated in cases of bilateral TON.18
to understand why TON occurs and how it is treated. The optic nerve can be separated into anterior (optic nerve head) and posterior (intraorbital, intracanalicular, and intracranial) segments. Injury to the anterior optic nerve or its blood supply may occur at its point of insertion into the eye after forceful globe rotation. With complete or partial avulsion of the nerve from the globe, an intraocular hemorrhage at the nerve head is seen during funduscopic examination (Fig. 18.3). Injury to the nerve anterior to the central retinal artery entry point will disrupt retinal circulation causing occlusive retinopathy or retinal hemorrhages. More posterior injury is, however, much more common and the optic nerve and fundus will appear normal. The disruptive forces following closed head injury are concentrated at the orbital apex and optic canal, making the intracanalicular optic nerve the most vulnerable segment. The dural sheath that surrounds this segment is tightly adherent to the periosteum within the optic canal. Axonal loss may result from the primary insult of bony impingement following an optic canal fracture, shearing injury, or disruption of nerve microvasculature. A secondary insult then follows. Optic nerve swelling within the canal produces a localized compartment syndrome that precipitates ischemic damage and further axonal loss.7 The intracranial optic nerve segment lies near the falciform dural fold and is perhaps the second most common site of injury. This segment is more commonly implicated in cases of bilateral TON.18
Goals of Treatment
The primary insult typically results in irreversible axonal death. The overriding goal of intervention is to improve or preserve visual function by minimizing the effect of secondary insults, including optic nerve swelling, vasospasm, ischemia, and release of neurotoxic factors. The four main options are high-dose systemic corticosteroid therapy, optic canal decompressive surgery (OCDS), a combination of steroids and surgery, or observation alone (no treatment). There is sound biologic rationale for considering these options. High-dose systemic corticosteroids decrease inflammatory mediators and surgical expansion of the bony optic canal creates space for a swollen nerve, minimizing the damaging effect of compressive forces. However, some data suggest that such interventions may actually do more harm than good. Furthermore, there are relatively high rates of spontaneous visual recovery (50% or greater) following indirect TON with observation alone. Steroids and surgery have not been shown to produce significantly better results. Neuroprotective therapies are emerging that may prove effective for TON, superseding both steroids and surgery.
There remains a lack of consensus regarding which management option for TON is best. It is difficult to draw meaningful conclusions from the literature, which is mostly comprised of small, retrospective studies without controls and with differing recruitment criteria, treatment regimens, time to diagnosis of TON, and initiation of therapy. The purpose of this chapter is to provide clinically relevant guidelines regarding the treatment of TON based on the best available evidence. In each section, the implications for practice are highlighted prior to a detailed review of the relevant studies. Areas of future research are summarized at the end of each section.
High-Dose Systemic Corticosteroid Therapy
The main rationale for using high-dose corticosteroid therapy in TON is to reduce optic nerve swelling and prevent axonal loss. Additional benefits may include antioxidant, free radical scavenging, prevention of lipid peroxidation, or other neuroprotective effects on the injured nerve.19,20 The most commonly studied corticosteroid for TON is intravenous (IV) methylprednisolone. Dexamethasone has also been used by some groups.17,21 There are several systemic risks of high-dose corticosteroids that include sepsis, pneumonia, gastrointestinal bleeding, hepatic failure, and wound complications. Rarely, serious life-threatening infections (i.e., mucormycosis) have been reported after high-dose steroid therapy for TON.22 Some early experimental animal models of spinal cord injury showed that methylprednisolone improves neurologic recovery.23 The therapeutic doses used in such studies helped develop protocols for the clinical studies that followed. In 1982, Anderson et al. first reported the use of corticosteroid therapy for six patients with TON.24 Half demonstrated visual recovery, but this study provided only anecdotal evidence. Several additional small, retrospective case series without controls followed, which also suggested that steroids may be an effective treatment option for TON.17,25,26 However, there was a lack of consensus regarding steroid type, dosage, and timing. In 1990, a large prospective study was published in the neurosurgical literature that validated high-dose steroid therapy as a treatment option for traumatic nerve injury.
The National Acute Spinal Cord Injury Studies
In 1985, the National Institutes of Health (NIH)-sponsored National Acute Spinal Cord Injury Studies (NASCIS) II trial27 was initiated to determine if high-dose corticosteroid therapy improves neurologic recovery following acute spinal cord injury (ASCI). This multicenter, randomized, double-blind, placebo-controlled trial recruited 487 patients
that suffered ASCI within 12 hours who met the inclusion criteria. Patients with involvement of the nerve root or cauda equina only, those with gunshot wounds, pregnant patients, those addicted to narcotics, those receiving maintenance steroids for other reasons, those under 13 years of age, those having already received corticosteroids pre-randomization, or those suffering life-threatening morbidity were excluded. Patients were randomly assigned to one of three treatment arms within 12 hours after injury: high-dose methylprednisolone (162/487), naloxone (154/487), or placebo (171/487). The treatment protocol for IV methylprednisolone consisted of a loading dose (30 mg/kg) over 15 minutes followed by a continuous infusion of 5.4 mg/kg/hour for 23 hours. Naloxone is an opiate receptor antagonist that was given as a 5.4 mg/kg bolus followed by 4 mg/kg/hour for 23 hours. Motor and sensory functions were assessed by systemic neurologic examination at admission, 6 weeks and 6 months after injury. At 1-year follow-up, the mean difference in motor scores between steroid and placebo groups was significant (5.20, 95% confidence interval [CI] 0.53-9.87). This was not the case for sensory scores (2.41, 95% CI -1.72-6.54). Treatment benefit was limited to patients who began methylprednisolone therapy within 8 hours after injury. There was a trend toward higher rates of gastrointestinal bleeding and wound infections in the steroid group that was not statistically significant. The investigators concluded that high-dose methylprednisolone improves neurologic recovery after traumatic ASCI compared with placebo when administered within 8 hours of trauma. However, this study suffers several important limitations. The 8-hour stratification was based on post hoc data analysis of a subgroup of patients (129 of 487, 26%). When this stratification is removed, the methylprednisolone group actually had worse overall outcomes compared with placebo.28,29 Other concerns include lack of proper randomization, with a bias in favor of steroid therapy. Finally, although neurologic improvement was statistically significant, it resulted in only minimal clinical improvement in daily functioning. The Third National Acute Spinal Cord Injury Study (NASCIS III) found that patients treated within 3 hours of injury could be maintained on continuous IV infusion for 24 hours, while those treated within 3 to 8 hours after injury benefited from 48 hours of continuous IV infusion.30 This study found an increased risk of sepsis and pneumonia in the steroid group.
that suffered ASCI within 12 hours who met the inclusion criteria. Patients with involvement of the nerve root or cauda equina only, those with gunshot wounds, pregnant patients, those addicted to narcotics, those receiving maintenance steroids for other reasons, those under 13 years of age, those having already received corticosteroids pre-randomization, or those suffering life-threatening morbidity were excluded. Patients were randomly assigned to one of three treatment arms within 12 hours after injury: high-dose methylprednisolone (162/487), naloxone (154/487), or placebo (171/487). The treatment protocol for IV methylprednisolone consisted of a loading dose (30 mg/kg) over 15 minutes followed by a continuous infusion of 5.4 mg/kg/hour for 23 hours. Naloxone is an opiate receptor antagonist that was given as a 5.4 mg/kg bolus followed by 4 mg/kg/hour for 23 hours. Motor and sensory functions were assessed by systemic neurologic examination at admission, 6 weeks and 6 months after injury. At 1-year follow-up, the mean difference in motor scores between steroid and placebo groups was significant (5.20, 95% confidence interval [CI] 0.53-9.87). This was not the case for sensory scores (2.41, 95% CI -1.72-6.54). Treatment benefit was limited to patients who began methylprednisolone therapy within 8 hours after injury. There was a trend toward higher rates of gastrointestinal bleeding and wound infections in the steroid group that was not statistically significant. The investigators concluded that high-dose methylprednisolone improves neurologic recovery after traumatic ASCI compared with placebo when administered within 8 hours of trauma. However, this study suffers several important limitations. The 8-hour stratification was based on post hoc data analysis of a subgroup of patients (129 of 487, 26%). When this stratification is removed, the methylprednisolone group actually had worse overall outcomes compared with placebo.28,29 Other concerns include lack of proper randomization, with a bias in favor of steroid therapy. Finally, although neurologic improvement was statistically significant, it resulted in only minimal clinical improvement in daily functioning. The Third National Acute Spinal Cord Injury Study (NASCIS III) found that patients treated within 3 hours of injury could be maintained on continuous IV infusion for 24 hours, while those treated within 3 to 8 hours after injury benefited from 48 hours of continuous IV infusion.30 This study found an increased risk of sepsis and pneumonia in the steroid group.
Extrapolating National Acute Spinal Cord Injury Studies to Traumatic Optic Neuropathy Management
The results of NASCIS II were extrapolated to TON management by the ophthalmic community leading to widespread use of high-dose corticosteroids. From 1990 to 2010, 43 case series (each with a minimum of 10 participants with TON) have been published.31 From the cumulative 1,906 participants identified in these studies, 76% were treated with corticosteroid therapy either alone or in combination with OCDS. Drawing meaningful conclusions from the published case series is difficult because of numerous methodologic flaws. These include small sample sizes, retrospective analysis, lack of randomization, unknown time interval between injury and treatment initiation, and lack of consistent visual function assessment and follow-up. In the older studies, patients were treated relatively late postinjury (i.e., weeks) and had reportedly worse outcomes compared with newer studies, in which patients were treated more rapidly (i.e., hours to days) from injury onset. A debate regarding whether or not steroids should be used in TON has persisted. In addition to equivocal clinical evidence, the biologic rationale for using steroids is also questionable. The optic nerve is a white matter axonal tract whose cell bodies reside in the retina while the spinal cord is a mixed axonal tract made up of both white and grey matter. To clarify whether or not steroids are effective for TON, a large, multicenter, prospective study was organized in 1994 by 76 investigators in 16 countries known as the International Optic Nerve Trauma Study (IONTS).1
International Optic Nerve Trauma Study
The main purpose of this study was to determine whether high-dose corticosteroid therapy
or OCDS improves visual recovery after indirect TON.1 It was designed as a multicenter, prospective, randomized clinical trial but converted to a nonrandomized, comparative interventional study after 2 years due to lack of enrollment. Participants comprised 133 patients with indirect TON (127 unilateral, 6 bilateral) that were evaluated within 3 days and treated within 7 days following injury. The three treatment arms for unilateral TON patients were corticosteroid therapy (85/133), OCDS (33/133), or observation (9/133). Methylprednisolone regimens were defined as megadose (greater than 5,400 mg/day), very high dose (2,000-5,400 mg/day), high dose (500-1,999 mg/day), moderate dose (100-499 mg/day), and low dose (less than 100 mg/day). Most patients received either a megadose (40%) or a very high dose (18%) regimen, and all of the patients in the surgical treatment arm, except one, received concomitant steroids; 104 cases were available for follow-up at 1 month and 40 cases at 6 months. The main outcome measure was improvement in best-corrected visual acuity (BCVA) after adjusting for baseline. BCVA improved by 3 lines or more in 52% of patients in the steroid group, 32% of patients in the surgery group, and 57% of patients in the observation group (p = 0.22). Dose or timing of steroid therapy was not associated with probability of visual recovery. The investigators concluded that neither corticosteroids nor OCDS had significantly better outcomes than observation alone. The most important criticism of this study is the bias toward treatment (94% of patients received corticosteroids at varying doses).31 There was also a lack of uniform steroid dosing, timing of treatment, and indication for OCDS. Other case series have similarly found that 40% to 60% of patients with TON improve with steroids,17,32,33 a rate comparable to spontaneous recovery without treatment.
or OCDS improves visual recovery after indirect TON.1 It was designed as a multicenter, prospective, randomized clinical trial but converted to a nonrandomized, comparative interventional study after 2 years due to lack of enrollment. Participants comprised 133 patients with indirect TON (127 unilateral, 6 bilateral) that were evaluated within 3 days and treated within 7 days following injury. The three treatment arms for unilateral TON patients were corticosteroid therapy (85/133), OCDS (33/133), or observation (9/133). Methylprednisolone regimens were defined as megadose (greater than 5,400 mg/day), very high dose (2,000-5,400 mg/day), high dose (500-1,999 mg/day), moderate dose (100-499 mg/day), and low dose (less than 100 mg/day). Most patients received either a megadose (40%) or a very high dose (18%) regimen, and all of the patients in the surgical treatment arm, except one, received concomitant steroids; 104 cases were available for follow-up at 1 month and 40 cases at 6 months. The main outcome measure was improvement in best-corrected visual acuity (BCVA) after adjusting for baseline. BCVA improved by 3 lines or more in 52% of patients in the steroid group, 32% of patients in the surgery group, and 57% of patients in the observation group (p = 0.22). Dose or timing of steroid therapy was not associated with probability of visual recovery. The investigators concluded that neither corticosteroids nor OCDS had significantly better outcomes than observation alone. The most important criticism of this study is the bias toward treatment (94% of patients received corticosteroids at varying doses).31 There was also a lack of uniform steroid dosing, timing of treatment, and indication for OCDS. Other case series have similarly found that 40% to 60% of patients with TON improve with steroids,17,32,33 a rate comparable to spontaneous recovery without treatment.
Because the results of IONTS were inconclusive, a single-center, randomized, double-blind, placebo-controlled study was done by Entezari et al. in 2007 to determine if high-dose methylprednisolone therapy is better than observation alone for TON.34 Patients with indirect TON evaluated within 7 days were enrolled. Unconscious patients, those with penetrating trauma, direct TON, hazy ocular media, presence of blow-out fracture, and candidates for OCDS were excluded. From 31 eligible participants, 16 received steroids and 15 placebo. Each participant was randomly assigned to either the placebo or the treatment arm. Treatment consisted of IV methylprednisolone (250 mg every 6 hours for 3 days) followed by oral prednisone (1 mg/kg daily for 14 days). Participants and clinicians performing ophthalmic examinations were masked to treatment assignment. Mean BCVA by logarithmic of the minimum angle of resolution (logMAR) was measured at presentation and at the 3-month follow-up. Recovery of visual acuity was defined as a decrease of at least 0.40 logMAR units after 3 months. Three-month follow-up data was available for all 31 participants. The mean final BCVA was 1.78 +/− 1.23 logMAR (Snellen equivalent 20/1,205) in the placebo group compared with 1.11 +/− 1.14 logMAR (Snellen equivalent 20/258) in the steroid group, which was not statistically significant (p = 0.13). The mean BCVA improved by 0.40 logMAR in eight eyes (53.3%) in the placebo group and in 11 eyes (68.8%) in the steroid group, which was not statistically significant (p = 0.38). The authors concluded that a high rate of spontaneous visual recovery occurs and there is no evidence that steroids provide any additional benefit compared with observation alone. The main limitations of this study were a relatively small sample size and the issue of many patients receiving treatment days after injury, at which point steroids may confer less benefit than an earlier intervention.
Cochrane Review: Steroids for Traumatic Optic Neuropathy
In 2011, Yu-Wai-Man and Griffiths performed a systematic review of randomized controlled trials (RCTs) to determine whether steroids are effective and safe in TON based on the best available evidence.35 Trials were included if patients were diagnosed with either direct or indirect TON. Bilateral cases were excluded. Only RCTs for TON treated with the following regimens were included: 1. any steroid regimen versus
no treatment; 2. any steroid regimen versus any form of surgical optic nerve decompression, and 3. any steroid regimen versus a combination of steroids and surgery. The primary outcome measure was number of lines of visual acuity gained or lost at 3 and 6 months follow-up. Snellen ratios were converted to logMAR decimal values. Secondary outcome measures included other validated tests of visual function, adverse outcomes related to treatment, and validated quality-of-life measures. The authors searched databases including CENTRAL, MEDLINE, EMBASE, LILACS, mRCT, ClinicalTrials.gov, and Web of Science CPCI-S. There were no language or date restrictions in their search for trials and the last search was on November 23, 2010. Other resources were searched including reviews and book chapters and contacting trial investigators and experts to identify additional published and unpublished studies. Two review authors independently extracted data from and appraised the studies for methodologic quality. The risk for bias was assessed for parameters including generation of randomization sequence, allocation concealment, masking (blinding) clinicians, and extent of follow-up for each study. A total of 501 references were identified after deduplication. The only study that met inclusion criteria was by Entezari et al.34 This study was graded as having a low risk of bias. Based on this review, the authors found no convincing evidence that steroids provide any additional visual benefit in TON. Similarly, Lee et al.2 have studied epidemiologic data in the United Kingdom on TON incidence, management, and visual recovery and found no significant benefit of steroid or surgical therapy. They highlight a trend among physicians toward conservative management.
no treatment; 2. any steroid regimen versus any form of surgical optic nerve decompression, and 3. any steroid regimen versus a combination of steroids and surgery. The primary outcome measure was number of lines of visual acuity gained or lost at 3 and 6 months follow-up. Snellen ratios were converted to logMAR decimal values. Secondary outcome measures included other validated tests of visual function, adverse outcomes related to treatment, and validated quality-of-life measures. The authors searched databases including CENTRAL, MEDLINE, EMBASE, LILACS, mRCT, ClinicalTrials.gov, and Web of Science CPCI-S. There were no language or date restrictions in their search for trials and the last search was on November 23, 2010. Other resources were searched including reviews and book chapters and contacting trial investigators and experts to identify additional published and unpublished studies. Two review authors independently extracted data from and appraised the studies for methodologic quality. The risk for bias was assessed for parameters including generation of randomization sequence, allocation concealment, masking (blinding) clinicians, and extent of follow-up for each study. A total of 501 references were identified after deduplication. The only study that met inclusion criteria was by Entezari et al.34 This study was graded as having a low risk of bias. Based on this review, the authors found no convincing evidence that steroids provide any additional visual benefit in TON. Similarly, Lee et al.2 have studied epidemiologic data in the United Kingdom on TON incidence, management, and visual recovery and found no significant benefit of steroid or surgical therapy. They highlight a trend among physicians toward conservative management.
Steroids are Dangerous in Patients with Traumatic Brain Injury
In 2005, the Corticosteroid Randomization After Significant Head Injury (CRASH) study36,37 by Edwards et al. was published. This was a multicenter, randomized, double-blind, placebo-controlled study whose purpose was to determine whether high-dose corticosteroid therapy is effective and safe in patients with acute head trauma. Adults with head trauma and a Glasgow Coma Scale score of 14 or less presenting within 8 hours of injury were recruited. This international collaboration planned to enroll 20,000 patients, but the data monitoring committee disclosed unmasked results leading to no further recruitment after 10,008 patients. Participants were randomly assigned to receive a 48-hour infusion of IV methylprednisolone (2 g over 1 hour loading dose followed by 0.4 g/hour for 48 hours) or placebo. The primary outcome measures were death within 2 weeks of injury and death or disability at 6 months. Analyses were done on an intention-to-treat basis. The effect measure used was relative risk (RR) with 95% CI for the overall risk and 99% CI for the results of subgroups. Homogeneity in treatment effects within subgroups was assessed with a chi-squared test on two degrees of freedom at a 5% significance level. Data at 6 months were obtained for 9,673 (96.7%) patients. The risk of death from all causes within 2 weeks was higher in the steroid group (21.1% versus 17.9%, p = 0.0001). This relatively higher mortality rate was independent of injury severity (p = 0.22) and time postinjury (p = 0.05). At 6 months follow-up, the risk of death was higher in the steroid group compared with placebo (25.7% versus 22.3%; RR 1.15, 95% CI 1.07-1.24, p = 0.0001). This was also true for the risk of death or severe disability (38.1% versus 36.3%; RR 1.05, 95% CI 0.99-1.10, p = 0.079). The timing or severity of injury was not significant. The authors concluded that high-dose corticosteroid therapy in the setting of acute traumatic brain injury is associated with increased rates of mortality and disability. A mechanism that explains why this occurs remains unknown. A Cochrane review of corticosteroids for acute traumatic brain injury had similar conclusions to CRASH.38
The results of the CRASH trial have largely resulted in the abandonment of high-dose corticosteroids in the setting of acute brain injury because of an increased mortality rate. These findings make it
particularly important to look for evidence of concomitant acute head trauma prior to considering therapeutic options for TON. In addition to the lack of supportive clinical data, several recent animal models of TON have shown either no effect39 or a detrimental effect of high-dose methylprednisolone on retinal ganglion cell (RGC) survival and axonal regeneration.40,41,42 In fact, Steinsapir et al. found that high-dose methylprednisolone exacerbated axonal loss following experimental optic nerve crush injury in rats in a dose-dependent fashion (P < 0.02).41 Another animal model of high-dose methylprednisolone for optic neuritis also showed greater axonal loss in the steroid group compared with controls.43 One experimental study showed that head trauma preceding optic nerve injury confers a neuroprotective effect to optic nerve axons, which is lost after administration of high-dose methylprednisolone.44
particularly important to look for evidence of concomitant acute head trauma prior to considering therapeutic options for TON. In addition to the lack of supportive clinical data, several recent animal models of TON have shown either no effect39 or a detrimental effect of high-dose methylprednisolone on retinal ganglion cell (RGC) survival and axonal regeneration.40,41,42 In fact, Steinsapir et al. found that high-dose methylprednisolone exacerbated axonal loss following experimental optic nerve crush injury in rats in a dose-dependent fashion (P < 0.02).41 Another animal model of high-dose methylprednisolone for optic neuritis also showed greater axonal loss in the steroid group compared with controls.43 One experimental study showed that head trauma preceding optic nerve injury confers a neuroprotective effect to optic nerve axons, which is lost after administration of high-dose methylprednisolone.44
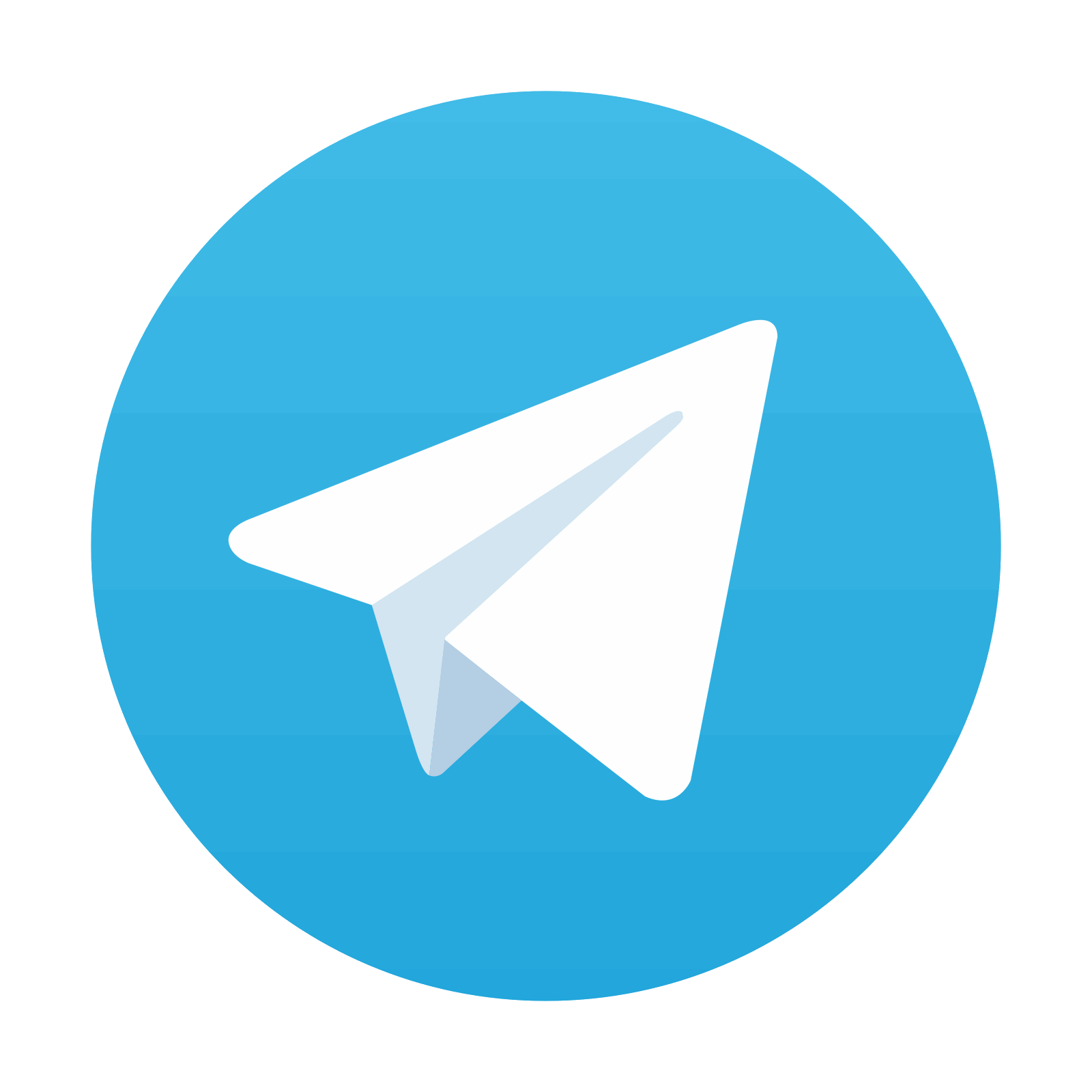
Stay updated, free articles. Join our Telegram channel

Full access? Get Clinical Tree
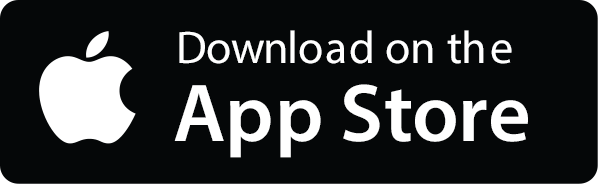
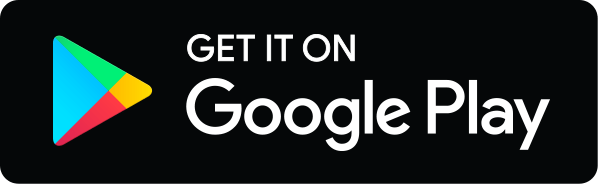