Introduction
Perimetry and visual field testing have been used as diagnostic procedures for evaluation of visual function for nearly 200 years, and a historical review of these techniques may be found in several publications. During this period of time, a variety of new techniques have been developed, although the standard clinical procedure of detecting a small white target superimposed on a uniform background at different peripheral locations in the field of view is still the method that is most commonly used by eye care specialists. Perimetry is used clinically to detect functional losses produced by pathology to the visual pathways, for differential diagnostic purposes to identify the location of a visual deficit, to monitor the status of acute and chronic disease processes, and to evaluate the efficacy of treatment. In spite of the similarity of current test procedures to early techniques, significant progress has recently been made in the presentation, analysis and interpretation of visual field findings. This has resulted in the automation of procedures, standardization of methods, the immediate statistical evaluation of test results and enhanced efficiency of testing. In this chapter, we will provide an overview of the psychophysical basis for perimetry, a discussion of the types of perimetric test strategies, the data representation formats that are used for visual field testing, important factors for interpreting visual field results, recognition of patterns of visual field loss, strategies for monitoring progression of visual field changes, and guidelines for assessment of visual field information in a clinical setting. Additionally, we will briefly describe some of the new visual field test procedures that have been shown to provide salient information concerning the functional status of the visual system and have been reported by many investigators to be clinically useful.
The psychophysical basis for perimetry
For conventional perimetric testing, the patient’s task is to detect a small white target superimposed on a uniform background luminance at different visual field locations. The detection task is based on the increment or differential light threshold, which determines the minimum amount of light that must be added to a stimulus (ΔL) in order to make it distinguishable from the background (L) and is known as Weber’s Law, or ΔL/L = C. In order for Weber’s Law to be valid, the background adapting luminance of the perimeter must be in the photopic range (typically 31.5 apostilbs, or 10/candelas per meter squared), pupil size must be sufficiently large (greater than 2 mm), ocular media must be relatively clear, and other test conditions (stimulus size, stimulus duration, etc.) must be within appropriate levels. Under these conditions of photopic adaptation in a normal eye and visual system, the fovea is the visual field location with the highest sensitivity. As eccentricity from the fovea increases, there is a significant drop in sensitivity out to about 3 degrees, then a more gradual sensitivity decline out to about 30 degrees radius, followed by a more rapid decrease beyond 30 degrees. The temporal visual field (away from the nose) extends farther than the nasal visual field, and the inferior visual field extends farther than the superior visual field. The three-dimensional shape of the sensitivity profile of the visual field has therefore often been referred to as the “hill of vision” or the “island of vision in a sea of blindness”, as shown in Figure 35.1 . Note that there is a “hole” in the island of vision that represents the blind spot, a location where the optic nerve exits the eye where no photoreceptors or other retinal neural elements are present. The blind spot is represented as an area of non-seeing by a dark solid oval located approximately 15 degrees from the center of view (fixation) in the temporal visual field extending one-third above and two-thirds below the horizontal meridian. From a clinical standpoint, one of the primary purposes of perimetry and visual field testing is to search for departures from this normal sensitivity profile (sensitivity losses) and determine the shape and location of the deficit.

As with all psychophysical assessments, there is variability in the values obtained for repeated measures in the sensitivity to light increments. Several laboratories have indicated that under clinical testing conditions, the standard deviation of repeated measures is approximately 3 dB for individual visual field locations with normal sensitivity. As sensitivity declines in damaged visual field areas, there is an increase in variability of about 300–400 percent that extends the 95 percent confidence interval to nearly the entire operating range of the perimeter. One method of quantitatively evaluating this variability is by obtaining a frequency of seeing (FOS) curve, which plots the percentage of stimuli seen as a function of the luminance of the stimulus. The FOS curve is typically quite steep in areas with normal sensitivity, and becomes flatter at greater eccentricities and in damaged visual field areas. From a clinical perspective, it is important to minimize response variability so that subtle changes in sensitivity that are due to pathology can be more readily detected. This can be accomplished by using larger stimuli, modifying the type of stimulus used, adjusting the response estimate algorithm and several other procedures.
The physiologic basis for perimetry
Proper interpretation of visual field results requires knowledge of the anatomical arrangement of the visual pathways. As shown in Figure 35.2 , a panoramic image of the visual field of view is formed on the retina by the optical components (cornea, lens, pupil) of the eye. The light image is converted into electrical impulses that are transmitted through more than 100 million photoreceptors to other retinal neural elements and eventually to approximately 1 million retinal ganglion cells. The retinal ganglion cell axons enter the optic nerve and travel back to eventually reach primary visual cortex. As the optic nerve fibers leave the eye and travel towards the brain, there is a separation of nerve fibers subserving the nasal and temporal visual field along the vertical meridian. This separation occurs at the optic chiasm, whereby the nasal visual field from one eye combines with the temporal visual field of the other eye, so that the left half of vision is represented in the right hemisphere and the right half of vision is represented in the left hemisphere. At this point in the visual pathways, there is not a point-for-point retinotopic mapping of locations between the two eyes. The nerve fibers continue on to a portion of the thalamus (the lateral geniculate nucleus) where they synapse with nerve fibers that convey information to primary visual cortex known as area 17 or V1. In primary visual cortex, there is a point-for-point mapping of retinotopic information from both eyes (under normal development) as a consequence of rearrangement of fibers traveling from the optic chiasm. Beyond primary visual cortex, there are complex interactions with other areas of the brain that are beyond the scope of this chapter.

Types of perimetric testing
Currently, there are three different forms of visual field testing that are performed clinically: kinetic perimetry, static perimetry, and suprathreshold static perimetry. Each of these procedures has particular advantages and disadvantages, as described below, and each of them serves distinct purposes for clinical diagnostic testing. In this view, it should be noted that these techniques should be regarded as complementary, with only moderate amounts of overlap in clinical performance.
Kinetic perimetry
As implied by its name, kinetic perimetry involves the movement of a small target on a uniform background consisting of a 1- or 2-meter dark tangent screen or a light hemispherical bowl perimeter (Goldmann or Goldmann-like perimeter). A skilled kinetic perimetrist usually requires a considerable amount of apprenticeship time (6–12 months) to become proficient in these techniques. A thorough description of kinetic perimetry strategies is beyond the scope of this chapter, although an excellent description of kinetic perimetric techniques and strategies may be found in the book by Anderson.
Kinetic perimetry consists of mapping the hill of vision (boundary between seeing and non-seeing) by moving a target of predetermined size and luminance from the periphery towards the fixation point along successive meridians separated by approximately 15 degrees of angular extent. The rate of smooth movement should be 4–5 degrees per second for greater eccentricities, and 1–2 degrees per second for locations near fixation. For a particular target, the boundary locations are connected by a line of equal sensitivity known as an isopter. Multiple isopters can be generated by changing the size or luminance of the target. For the far periphery, target size is usually varied, whereas luminance is altered for evaluation of nearer eccentricities. A normal isopter is relatively egg-shaped, with the long axis of the egg extending to the temporal visual field and the short portion of the axis of the egg projecting to the nasal visual field. Similarly, the inferior visual field extends farther peripherally than the superior visual field. If an isopter corresponds to this shape, then no further evaluation is needed. However, if local indentations of an isopter are present, further moving target scans should be directed from the periphery in a direction perpendicular to an imaginary line drawn between the two points. If further definition of the isopter contour is needed, this process should be repeated. Typically, between 3 and 6 isopters are required to provide a thorough characterization of the visual field.
Within the central 30–40 degrees radius of the visual field, a series of arcuate “spot checks” are usually performed between isopters and in locations where one or more isopters show an indentation. Static spot checks are performed with the kinetic isopter stimulus, inside the isopter and consist of a brief flash of the target. A location where a spot check is missed should be checked again to confirm non-detection. In these missed spot check areas or regions with local recession or depression of isopters, scotoma mapping should be performed, beginning at the presumed center of the defect and moving outwards in 8 radial directions (horizontal, vertical and oblique). Additional intermediate scans can also be performed to better characterize the shape of the deficit, and multiple targets should be used to indicate the depth of the deficit and the slope of its borders. The final result is a topographic mapping of the hill of vision to provide a two-dimensional representation of the three-dimensional structure of the visual field.
Advantages of kinetic perimetry include the ability to characterize the entire central and peripheral visual field, enhanced flexibility and interaction between the examiner and the participant, the ability to accurately characterize the shape of visual field deficits, and a highly efficient method of evaluating the far peripheral visual field. Disadvantages consist of higher variability than other forms of perimetric testing, large individual differences in clinical performance among examiners, poorly standardized procedures, limited normative population characteristics, and difficulty in characterizing generalized or widespread visual field sensitivity loss for different age groups.
Although there are no specific quantitative features that can be easily described, Figure 35.3 presents an example of a normal visual field. Most kinetic perimetric testing is performed manually, except that several automated perimeters have recently incorporated kinetic testing procedures.

Static perimetry
To date, static perimetry is the most common method of clinical visual field testing, particularly for automated perimeters. Static perimetry involves the detection of a small stationary target that is superimposed on a uniform white background. At each visual field location that is tested, the measurement consists of the minimum amount of light increment (differential light threshold) that can be just distinguished from the background. Different visual field locations are tested along various radial meridians between the central point of fixation and eccentric locations, or according to a Cartesian grid of locations that usually bracket the horizontal and vertical meridians. A variety of test strategies have been developed for static perimetry, including the ascending method of limits, bracketing or staircase procedures, forecasting (Bayesian) threshold estimation procedures, and other efficient and adaptive testing strategies. Most eye care specialists today use some form of adaptive forecasting procedure such as the Swedish Interactive Threshold Algorithm (SITA), Zippy Estimation of Sequential Thresholds (ZEST), Tendency Oriented Perimetry (TOP) or a similar procedure. Additionally, methods of evaluating test performance (false positive responses, false negative responses, and blind spot fixation checks) and alignment (eye and head position) tracking have also been developed for static perimetry.
The representation of visual field results from automated static perimetry is typically displayed as numerical sensitivity values, general and pattern deviations from average expected normal values for a specific age group, gray scale sensitivity representations of visual field sensitivities, probability plots (deviations from average normal), and in some instances three-dimensional sensitivity density plots. Also, for the Humphrey Field Analyzer, summary statistics known as visual field indices are also determined to provide an indication of generalized or widespread sensitivity loss (mean deviation [MD]), localized sensitivity loss (pattern standard deviation [PSD]), visual field loss produced by asymmetric superior and inferior visual hemifield loss characteristic of glaucoma (glaucoma hemifield test [GHT]), and visual field progression (glaucoma progression analysis [GPA]) and rate of progression (glaucoma progression index [GPI]). Similar types of analyses are available for other automated perimeters.
Advantages of automated static perimetry include the use of a standardized test procedure, the ability to exchange information from one device and office to another, availability of an age-corrected normative database, immediate comparison of individual results to normal population characteristics, and methods for monitoring response reliability, attention and cooperation, proper alignment and greater reproducibility. Disadvantages of automated static perimetry consist of time-consuming and limited methods of evaluating the entire peripheral visual field, less flexible test procedures, a more demanding burden on the patient’s attention and performance, and very high test–retest variability when sensitivity is less than 20dB for a size III target.
Figure 35.4 presents examples of gray scale plots of static perimetry conducted for (a) a normal visual field, (b) a generalized or widespread visual field loss and (c) a localized visual field deficit.

Suprathreshold static perimetry
Suprathreshold static perimetry is generally used as a rapid screening procedure to detect visual field deficits. However, there are several drawbacks to suprathreshold static visual field testing using standard automated perimetry: (1) with a few exceptions, the vast majority of basic research in this area has been directed towards threshold estimation strategies rather than suprathreshold screening procedures, (2) there are a limited number of clinical studies that have been performed to evaluate the performance characteristics of these procedures, (3) threshold estimation strategies have become considerably more efficient and can actually take less time than some suprathreshold screening procedures, and (4) there is no consensus as to which suprathreshold visual field screening procedures are most suitable for routine use.
There are a wide variety of forms of suprathreshold static perimetric methods that are currently in use. The stimulus presentation patterns range from a small number of targets (less than 30) to nearly 200 locations. Some strategies present all targets at a fixed stimulus luminance, others use multiple stimulus luminance levels that are varied according to eccentricity, some perform threshold estimates for locations that have sensitivity below normal limits, some present multiple stimulus luminances in damaged locations to estimate the magnitude of sensitivity loss, some provide a retest of missed locations to confirm suspected sensitivity losses, and some use an adaptive strategy that provides greater spatial resolution of test points for suspicious visual field locations. Only a few studies have compared results obtained from different procedures or have evaluated screening results in comparison to threshold visual field techniques. This has created an opportunity for future investigations to determine methods that are appropriate for visual field screening in a clinical setting as well as population-based screening.
Suprathreshold static perimetry has the advantage of providing simple and efficient visual field screening techniques for the patient and the examiner, standardized procedures and the ability to test the entire peripheral visual field. Disadvantages include limited quantitative information, lower sensitivity and specificity in comparison to threshold techniques, a lack of appropriate decision rules and scoring systems that have been validated, and limitations in the ability to monitor progressive changes.
Figure 35.5 presents visual field screening results for the right eye of a patient with superior arcuate glaucomatous visual field loss using a multiple stimulus luminance test strategy for the Humphrey Field Analyzer.

Detection of perimetric sensitivity loss and interpretation of results
Perhaps the best method of providing instruction about interpretation of visual field results is to conduct a systematic overview of the findings that are presented on the printed output. Figure 35.6 presents a single visual field analysis of the results for the left eye of a patient with an inferior acruate glaucomatous visual field deficit that was obtained using the 24-2 SITA Standard test procedure on the Humphrey Field Analyzer II.

The top portion of the visual field printout shown in Figure 35.6 provides (from left to right and top to bottom) an indication of the patient’s name, the eye that was tested, the patient’s ID code and date of birth. Below this is information pertaining to the test conditions used (background luminance, stimulus size, fixation target, fixation monitoring method), the test strategy (SITA, Full Threshold, Suprathreshold screening), performance characteristics (false positive errors, false negative errors, fixation losses), the patient’s age, and ocular conditions (distance refraction, pupil size, visual acuity). False negative errors that exceed 33 percent are outside normal limits, although this occurs frequently in eyes with visual field loss. Excessive false positives are infrequent, and are often associated with excessive fixation losses because patients are “trigger happy”. For the Full Threshold procedure a criterion of 33 percent is used, but for SITA a new algorithm is being used, and 15 percent is the appropriate criterion for excessive false positives. Fixation losses that exceed 33 percent are considered to be excessive but methods can be employed to dramatically reduce excessive fixation losses, and head tilts can falsely elevate this percentage.
Below this information are the patient’s foveal threshold (if it is enabled for the test), an XY graphical presentation of sensitivity values, and a gray scale representation of visual sensitivity. The sensitivity values are indicated in decibels (dB), which for standard automated perimetry is defined as the contrast (increment threshold or Weber fraction, ΔL/L) needed to just detect a stimulus superimposed on the uniform background and reflects visual field sensitivity on a logarithmic scale. A value of 0 dB represents the brightest stimulus that can be produced by the instrument and superimposed on the background. Each 1 dB increment represents a 0.1 log unit increase in stimulus luminance. Thus, a 10 dB sensitivity represents an ability to detect a target that is 10 times dimmer than the maximum stimulus luminance, a 20 dB stimulus represents an ability to detect a target that is 100 times dimmer than the maximum stimulus luminance, and so forth. The gray scale representation of visual field sensitivity provides an immediate indication of the sensitivity properties of the hill of vision, and is able to convey areas of reduced sensitivity in the form of darkened portions of the graph. This provides a very rapid method of depicting the location, size, shape and depth of area of reduced visual field sensitivity.
Immediately below the gray scale representation are the values for the Mean Deviation (MD), Pattern Standard Deviation (PSD) and Glaucoma Hemifield Test (GHT) calculations. MD indicates the deviation of the patient’s visual field from the average normal results for an individual of the same age. PSD is the departure (root mean square [RMS] deviation) from the average decline in sensitivity from the fovea to the periphery out to 30 degrees radius (0.6 dB per degree for a Size III target). In some ways, PSD may be regarded as a quantitative indicator of visual field perturbation (irregularities in visual field surface with eccentricity), particularly because the edges of scotomas provide a distinct transition from a smooth sensitivity profile. The Glaucoma Hemifield Test (GHT) compares the sensitivity of clustered points for mirror image regions of the superior and inferior hemifields, as shown in Figure 35.7 . The five regions in the superior and inferior hemifields are designed to be representative of arcuate nerve fiber bundle areas that are most frequently involved in glaucomatous visual field losses (nasal steps, paracentral losses, partial arcuate and arcuate defects). In normal eyes, there are only minimal differences in sensitivity between these regions in the superior and inferior hemifields. However, in glaucoma and some other optic neuropathies, the damage to retinal locations corresponding to the superior and inferior hemifields is often asymmetric, resulting in large sensitivity differences between regions in the superior and inferior hemifields.

To the left of the visual field indices are the Total Deviation and Pattern Deviation graphs. The upper left graph for Total Deviation indicates the deviation of the patient’s threshold sensitivity from the average normal observer of the same age (plus values indicate higher than average normal sensitivity and minus values reflect lower than average normal sensitivity). The Pattern Deviation values to the right are derived by adjusting the patient’s visual field according to the 85th percentile (7th most sensitive point for the 24-2 stimulus presentation pattern) to account for widespread or generalized sensitivity differences (i.e. higher sensitivity values for the patient are adjusted down and lower sensitivity values are adjusted up). Once this is accomplished, the deviation from average normal sensitivity for a person of the same age is calculated. Below the numerical values for the Total and Pattern Deviation calculations are the probability plot graphs. For each visual field location, these plots display single dots for values that are within the normal 95 percent confidence limits, and dithered symbols of increasing darkness and density to indicate locations with sensitivity below the average age-adjusted normal 5 percent, 2 percent, 1 percent and 0.5 percent probability levels, respectively. Comparison of the Total and Pattern Deviation probability plots can be very useful for interpreting the visual field.
Figure 35.8 presents four examples of visual field results represented by the Total and Pattern Deviation probability plots. Figure 35.8A displays a normal Total Deviation plot, with many abnormal results for the Pattern Deviation plot. This indicates that the patient’s eye had sensitivity values that were above the 95 percent normal age-adjusted values, which is commonly caused by a “trigger happy” patient who inappropriately responds to targets that are not usually detected by normal individuals. Fixation losses and false positive errors are often outside normal limits in these cases as well. Figure 35.8B shows abnormalities that appear for the Total Deviation probability plot, but are not present for the Pattern Deviation probability plots. This is indicative of widespread or diffuse visual field loss. Figure 35.8C shows Total and Pattern Deviation probability plots that appear to be nearly identical, which is indicative of localized visual field sensitivity loss. Finally, Figure 35.8D shows abnormal visual field locations for both the Total and Pattern Deviation probability plots, with some locations indicated as abnormal for both graphs and other locations demonstrating an abnormality on the Total Deviation plot but not on the Pattern Deviation plot. This is a common presentation, which indicates a mixture of localized and widespread or diffuse visual field sensitivity loss.

Below this is the name and address of the eye clinic in the lower right, and a printout of gaze tracking from left to right. Gaze tracking is a valuable component of the visual field printout. During the test, a video camera is monitoring the location of the first Purkinje image (corneal reflex) relative to the edges of the pupil (retinal red reflex) from an infrared light source that does not interfere with the test procedure. This method can then be used to monitor eye and head alignment during the test, as well as eye blinks and droopy lids during the visual field test procedure. The visual field printout has a time line from left to right that presents eye blinks and upper eyelid drooping (a contiguous series of downward deflections) as well as eye and head alignment (upward tick mark deflections) throughout the test procedure. As shown in Figure 35.9 , this information can reveal much about the attention and cooperation of the patient during the test procedure. Figure 35.9A shows the results for a patient with good fixation and blinking behavior throughout the test. Figure 35.9B shows the results for a patient who develops excessive blinking and tear film breakup that may be related to the tested eye drying out from exposure to the infrared light (a rest break can assist in minimizing this problem). Figures 35.9C–E present results for a patient who becomes sleepy (droopy upper eyelid) throughout the test, a patient that becomes fatigued as the test progresses, and a patient with head and eye alignment problems during the test, respectively.

It is important to keep in mind that all of the information provided on this printout is essential, and that proper interpretation of visual fields requires taking all of these results into account. Often, there is a temptation to concentrate on portions of the printout and ignore or discount other information that is presented. This is not a good habit to establish, however, because it can lead to misinterpretation of the visual field findings and artifactual results due to specific testing circumstances that can be missed. It is also critical to remember that visual field testing is only one part of the vision examination, and that additional information from the patient’s exam, ocular and medical history, family medical history, and other factors need to be included in the final assessment.
Patterns of visual field loss associated with different pathologic conditions
Proper evaluation of patterns of visual field loss is probably the most vital aspect of visual field interpretation. It is important to keep in mind the three-dimensional nature of visual field properties so that X (width), Y (height) and Z (depth) coordinates are all taken into account. The shape and extent of a visual field defect, whether it has steep or sloping boundaries, and whether it “respects” (terminates abruptly) certain anatomical borders are all important features to consider. Figure 35.10 presents some schematic representations of fibers at different levels in the visual pathways and the patterns of visual field loss that correspond to damage at these locations.

In this section, we will outline some key features and patterns of visual field loss that will be of assistance in visual field interpretation.
- •
Generalized or widespread visual field sensitivity loss . This can be produced be a variety of conditions, ranging from changes in the ocular media to pathology to various portions of the visual pathways to attentional and cognitive factors. It is therefore a rather non-specific diagnostic sign.
- •
Ring scotomas . These defects (either a total or a partial ring of sensitivity loss in the mid periphery surrounded by normal visual field locations) are typically indicative of retinitis pigmentosa or some other type of retinal degeneration.
- •
Central scotomas . Generally, these types of defects are associated with diseases of the macular region of the retina, although optic neuropathies can also produce central scotomas.
- •
Defects that “respect” the nasal horizontal meridian . Generally these types of defects are associated with the retinal ganglion cell nerve fiber bundles, and are indicative of glaucoma or some other type of optic neuropathy. However, compromises of the retinal vasculature (e.g. a branch artery occlusion) can also produce this type of defect.
- •
Arcuate scotomas . These types of defects again typically affect either the arcuate nerve fiber bundles (glaucoma or other optic neuropathies) or the retinal vasculature.
- •
Nasal steps . These defects are primarily associated with glaucoma or other optic neuropathies.
- •
Centrocecal scotomas . These defects represent pathology to the papillomacular bundle of nerve fibers that subserve the region between the blind spot and fixation. Usually, the deficit is produced by optic neuropathies, but they can also be created by retinal disease, particularly those that involve edema (e.g. central serous retinopathy).
- •
Visual field constriction . This is a deficit that can be produced by a variety of causes, ranging from retinal disease to optic neuropathies.
- •
Defects that “respect” the vertical meridian . Because of the separation of nerve fibers along the vertical border at fixation, these defects are found for damage to the visual pathways at the optic chiasm or after the optic chiasm.
- •
Bitemporal defects . In nearly all cases, a bitemporal visual field defect that respects the vertical meridian indicates damage to the visual pathways at the optic chiasm.
- •
Defects that resemble a horizontal tongue . This is an uncommon visual field deficit that is only infrequently seen. The deficit can be for the “tongue”, or the tongue can be the only remaining visual field areas of seeing. It is produced by damage to visual pathway fibers at the level of the lateral geniculate body.
- •
Homonymous visual field defects . Damage to the visual pathways that is on the same side (right or left) of vision for both eyes is homonymous, and indicates a deficit that has occurred after the optic chiasm. A total homonymous hemianopic visual field defect (the entire right or left side of vision is missing) is indicative of a defect after the optic chiasm, but can be at any location within the optic radiations.
- •
Congruous and incongruous defects . In general, the greater the amount of incongruity of homonymous defects between eyes, the closer the site of damage is to the optic chiasm. Deficits for primary visual cortex (occipital lobe) are highly congruous, parietal lobe and temporal lobe deficits are semi-congruous.
- •
Pie in the sky defects . A homonymous visual field deficit that resembles a piece of pie missing from the superior visual field of both eyes is usually associated with a temporal lobe lesion.
- •
Pie on the floor defects . A homonymous visual field deficit that resembles a missing piece of pie that has fallen to the floor is more often the result of a parietal lobe lesion.
- •
Cookie-cutter punched out lesions . Visual field deficits that look as though one used a hole punch or cookie cutter to represent loss in both eyes is highly indicative of an occipital lobe (primary visual cortex) lesion.
We hope that these guidelines will assist you in the interpretation of visual field information.
Determination of visual field progression
The ability to accurately determine visual field progression and the rate of visual field progression is a vital component in following the functional visual status of patients over time. However, there is currently no general agreement or consensus concerning the best method of following visual field progression. This is best depicted by the fact that every multicenter clinical trial that has employed visual field results as an outcome measure has used a different method of defining progression. A review of the various methods used to determine visual field progression has been reported by several sources. Basically, visual field progression techniques can be divided into the categories of clinical judgment methods, classification systems, event analyses and trend analyses. Each procedure has its advantages and disadvantages. It should also be noted that most of the procedures for determining visual field progression have been directed towards glaucoma.
Clinical judgment consists of a practitioner using his/her knowledge and experience to make a determination of whether or not the visual field has undergone systematic changes over time. The main advantage of this procedure is that it is an easy, efficient method of evaluating visual field progression. The primary disadvantage is that there is a large amount of variability among eye care specialists for interpreting visual field progression. Previous investigations have demonstrated that even highly experienced, competent eye care specialists have a tendency to “overcall” visual field progression.
Classification systems consist of staging the amount of visual field damage into a series of categories. A historical review of visual field staging and classification systems for glaucoma has recently been published. In general, most classification systems use between 5 and 7 different levels of visual field damage to characterize the spectrum of loss. Exceptions to this include the systems derived by the Advanced Glaucoma Intervention Study (AGIS) and the Collaborative Initial Glaucoma Treatment Study (CIGTS), which use 20 stages. Advantages of classification systems include their use by several multicenter clinical trials and the ability to assign a quantitative value to visual field damage, whereas disadvantages consist of the use of a discrete outcome rather than a continuous function, and the lack of knowing the measurement scale (linear, non-linear, etc.) that the system is based upon.
Event analysis is most commonly performed by comparing the most recent visual field to those obtained at baseline visits. For this type of analysis, the intent is to determine whether visual field changes from baseline (progression or improvement) are within or outside expected limits. This technique has been used by several multicenter trials and is available on commercially available automated perimeters. Advantages of this procedure are that it is able to monitor visual field changes over time, and the application of more rigorous statistical procedures to analyze results. Included for disadvantages are the absence of evaluating interim visual fields between the current and baseline results, and the large influence of spurious results (meaningfully higher or lower sensitivity values for a single visit).
Trend analysis has also been used for many multicenter clinical trials, most commonly as a linear least-squares regression. This procedure analyzes all visual fields that have been obtained over time and determines the best fitting equation (usually a linear function) to describe the data. The slope of the line can then be used to provide an estimate of visual field change. Advantages of this procedure include the use of all the visual field data to obtain an estimate of change, based on a long-standing formal statistical model. Limitations of this procedure are related to a requirement for the slope to be statistically significantly different from zero, and a minimum of 6 to 8 visual fields that are necessary to achieve appropriate high clinical performance. Recently, there have been several enhancements that have been introduced to improve the performance of trend analysis, but it still requires a minimum of 5 to 6 visual fields before satisfactory performance can be achieved (i.e. high sensitivity and specificity). Note that the requirement of at least 5 to 6 visual fields holds for all of the current procedures for analyzing visual fields, which means that an accurate, precise and reliable estimate of visual field status may require several years of follow-up before an informed decision can be made with existing techniques. Clearly from a clinical perspective, there is a strong need for new, innovative approaches to this perplexing problem.
How do the various methods of determining visual field progression compare? Several laboratories have made such comparisons using the same visual field data set to evaluate according to the various methods. In summary, these studies indicate that there are considerable differences in the amount of time to detect progression, the number of reliable visual fields that are determined to be progressing, the number of progressing visual fields that are determined to be unchanged, and the influence of variability (long and short term) on final outcomes. Moreover, there is agreement among the various methods only about 50–60 percent of the time.
Given these difficulties, what can be done to assist the eye care specialist in determining visual field progression at the present time? Here are a few helpful hints:
- •
Examine the complete visual field history of the patient, not just the current and the most recent previous results. Otherwise, false positives (inappropriate evaluation of change) and false negatives (inappropriate evaluation of stability) will frequently occur.
- •
Use all of the available methods of assessing longitudinal visual field status (e.g. Overview Analysis, Change Analysis, Glaucoma Change Probability, Glaucoma Progression Analysis, Glaucoma Progression Index, Progressor, etc.).
- •
Compare visual field results with other clinical examination findings, the patient’s ocular and general medical history, and other pertinent information (visual fields alone do not provide all of the necessary information).
- •
When in doubt, perform an additional visual field test, use another type of visual field testing, and perform assessments of structural retinal, optic disc or neural (imaging) characteristics to confirm suspected visual field findings.
- •
Consult with colleagues to determine their analysis and interpretation of results.
In the future, what can be done to improve our ability to determine visual field changes over time? We believe that there are four factors that can assist us in this endeavor:
- (1)
The development of visual field techniques that provide larger changes that are related to pathology. As described in a later section, several techniques have been developed that appear to detect changes earlier than standard automated perimetry.
- (2)
The development of procedures to reduce variability in visual field measures, both within a single session and from one session to another. There have been some methods that have been introduced that appear to be of assistance in this regard.
- (3)
Development of better, more sophisticated statistical analysis techniques. Again, there have been some advances in statistical, neural network and related analytical approaches that have been made in recent times. However, clinical demands on optimizing patient care would be tremendously advanced if more reliable, accurate, precise and efficient methods could be developed for evaluating a patient’s visual field status over time.
- (4)
Development of methods to analyze “noise” and variability to search for meaningful signals of pathology. Traditionally, visual field variability has been viewed as something that needs to be eliminated, and there has been little research directed towards a systematic analysis of this information to look for markers of pathology. There are many areas of medicine and ophthalmology that have shown value in seeking out such markers. It is likely that there are “signatures” of specific patterns of “noise” or variability that are indicative of pathology of a dysfunctional visual system.
In summary, each of these suggestions for the future is directed towards improving the ability to extract meaningful signals and markers of visual system pathology and changes in visual status that can be revealed through visual field testing.
Finally, one of the most important factors that eye care specialists must consider in the management of patients is the rate of visual field progression. This is a crucial issue in determining the management and treatment regimen for patients, and in assessing how their visual impairment may affect their quality of life and activities of daily living. In this regard, Progressor and the Glaucoma Progression Index provide a means of forecasting the future visual field characteristics for the patient. However, it should be kept in mind that these procedures are based on a linear regression model, and it is not clear whether this or non-linear methods would be most appropriate for predicting future visual field outcome for the patient. This topic has only recently received attention by the research community, and it is clear that much additional work is needed.
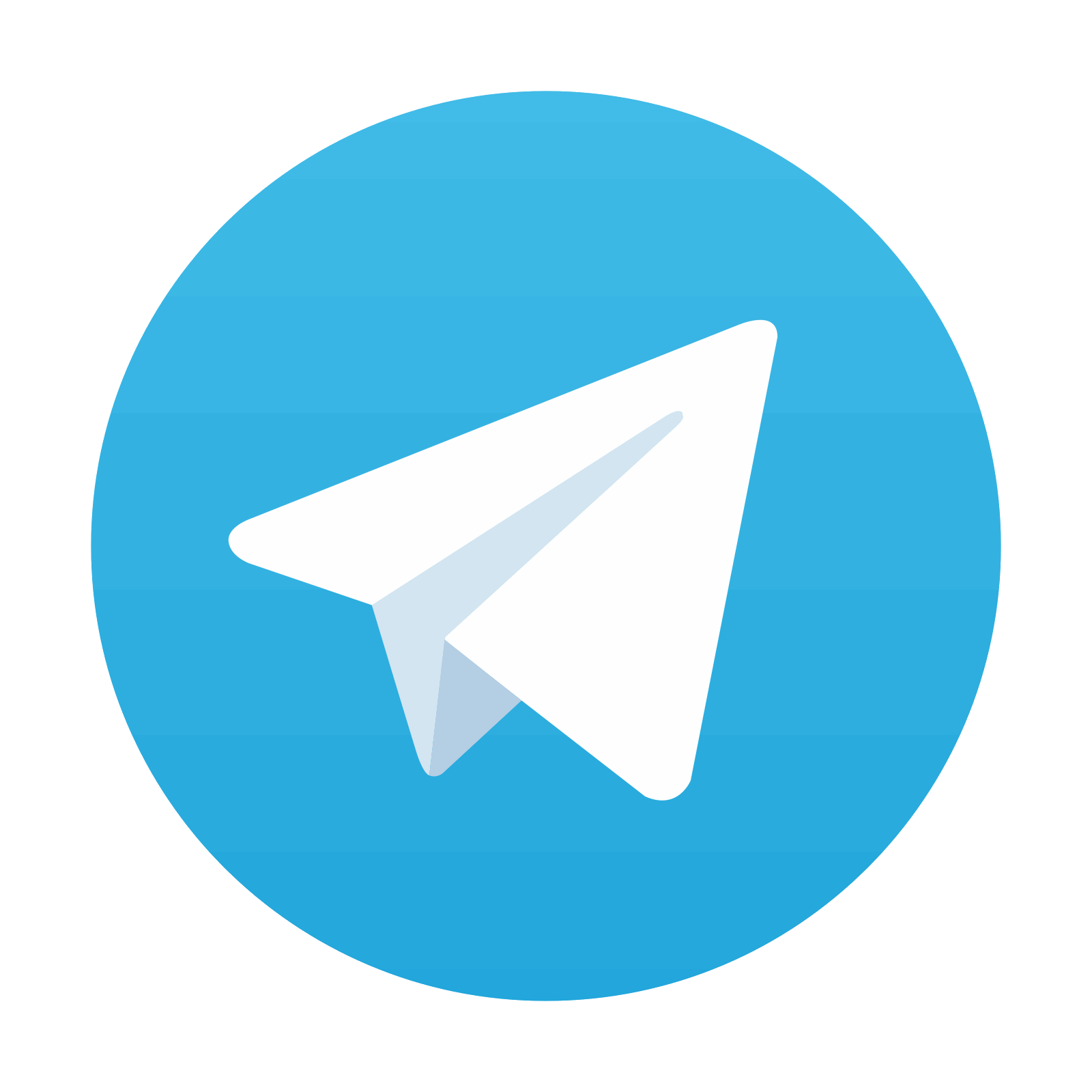
Stay updated, free articles. Join our Telegram channel

Full access? Get Clinical Tree
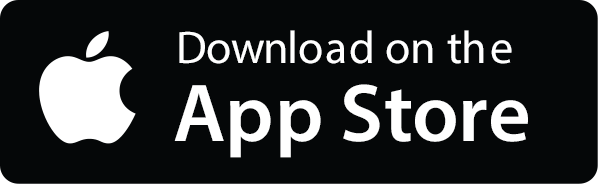
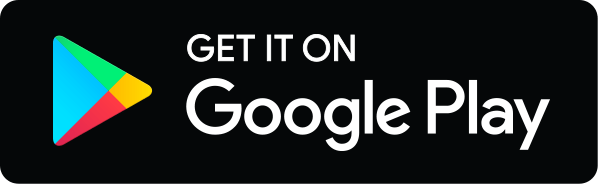