Abstract
Purpose
Modern operative microscopes use light sources which possess the power to severely damage underlying tissue. Currently, manufacturers provide a safety warning of this possibility. However, they are unable to suggest specific settings due to a stated “lack of scientific publications on this topic”. We aim to radiometrically evaluate multiple otologic microscopes at variables which effect irradiance in order to determine reference emissions levels and provide guidelines for improved intraoperative safety.
Materials and methods
The optical radiance of four otologic microscopes was evaluated at variable field illumination sizes (spot size), intensity settings and working distances. The spectral emission of each microscope was separately measured. The energy absorbed in skin with representative properties was then calculated as a function of time for each microscope by accounting for the emission spectrum of the microscope and the absorption spectrum of skin.
Results
Microscopes showed a wide range of optical radiance based on model, spots size, intensity setting and working distances. Spectral emission of all four microscopes was centered in the visible spectrum with minimal ultraviolet or infrared contribution. A large amount of energy is absorbed by skin during usage of operative microscopes. The highest calculated absorption at 200 min of use was 736.26 J/cm 2 .
Conclusions
Operative microscopes have the ability to cause patient morbidity secondary to the energy they impart. In an effort to decrease potential injury we recommend that physicians be aware of their microscopes properties and how to control variables which effect irradiance of the skin.
1
Introduction
Significant improvements have been made in operative microscopes since their introduction in the early 20th century. Optics have been improved as well as illumination sources. Traditional lighting sources such as incandescent bulbs have been replaced with advanced lighting including xenon and halogen lamps. Newer light sources provide excellent illumination even at high magnification, and with a large field of illumination (spot size); however, this power can be damaging to underlying tissue. Reports of secondary damage due to high intensity lighting have been reported in multiple microsurgical fields including ophthalmology, plastic surgery, neurovascular surgery and otologic surgery . Morbidity includes blindness, skin burns, and in cases of reconstructive surgery possible increased rates of free flap failure due to pedicle damage and secondary venous occlusion . A review of the Food and Drug Administration’s (FDA) Manufacturer and User Facility Device Experience (MAUDE) database for voluntary reports demonstrated 82 cases of soft tissue burns secondary to operative microscopes since 2004. Of these, 19 reports were otologic in nature detailing 25 ears burned . Two incidences of third degree burns after otologic procedures have occurred in our department.
Light sources can be evaluated in two distinctly separate ways. Radiometric analysis measures radiant energy produced by a source. Radiometric measurements are expressed as radiance which is radiation emitted from a source (W/Sr/m 2 ), and irradiance which is the irradiation of a surface (W/m 2 ). Photometric analysis measures the perceived brightness of light by the human eye. Photometric measurements are expressed as luminance which is brightness perceived by a human observer measured in lux (lumen/m 2 ).
The purpose of our study was to conduct a radiometric analysis of multiple operative microscopes under variable conditions to further evaluate the potential for harmful levels of irradiance. Since some light source might produce radiation outside of the visible spectrum we also measured the output of energy in both the infrared and ultraviolet regions.
2
Materials and methods
After Yale IRB approval four otologic microscopes were identified in use at our institution; two Ompi Pentero 900 microscopes (Carl Zeiss: Oberkochen, Germany), one Ompi Sensera microscope (Carl Zeiss: Oberkochen, Germany) and one Ompi Pico microscope (Carl Zeiss: Oberkochen, Germany). A calibrated 818-SL/DB photodiode (Newport Corporation, Irvine, CA) equipped with a 30dB attenuator was used to measure irradiance of each microscope across multiple variables including spot size, working distance and light intensity setting. Ambient room light was accounted for and removed from the final calculations. In addition, the emission spectrum of each microscope was separately measured at intensities of 5% and 100% at a working distance of 20cm.
The total energy absorbed per cm 2 of skin, A , was calculated based on Eq. (1) , where I ( λ ) is the measured lamp intensity per unit area at a wavelength λ , σ ( λ ) is the absorption coefficient of skin at λ , and L is the skin thickness, which was assumed to be 0.9 mm . In Eq. (1) , the term in the brackets represents the fraction of energy absorbed at a given wavelength through 0.9 mm thick skin.
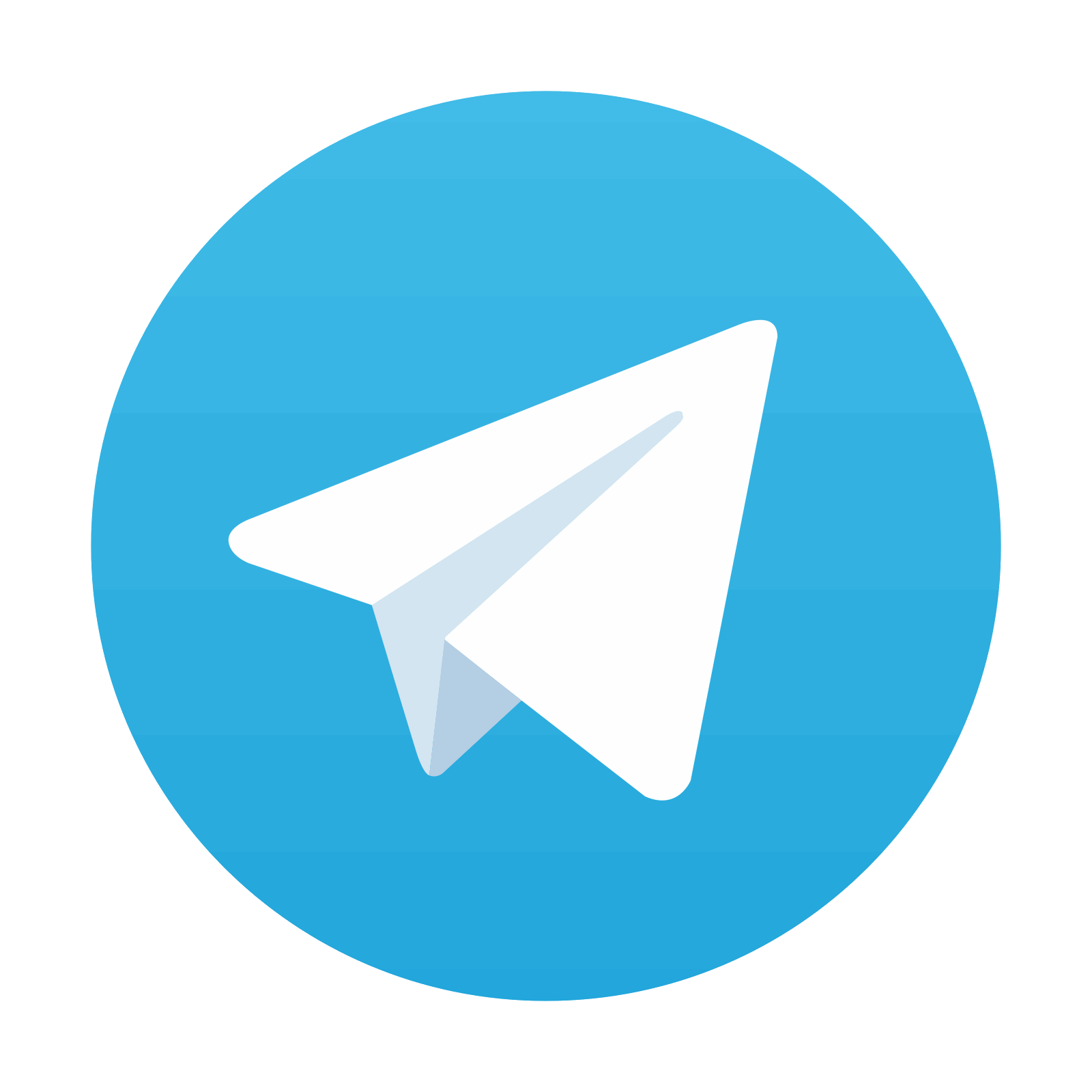
Stay updated, free articles. Join our Telegram channel

Full access? Get Clinical Tree
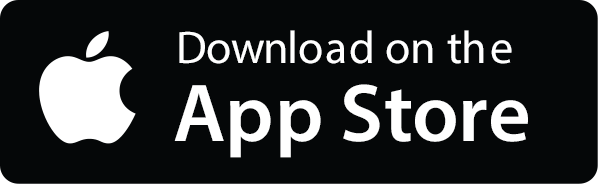
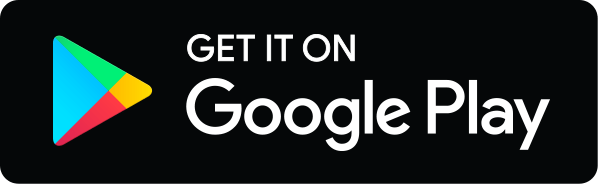