PIGMENTARY GLAUCOMA
Pigmentary glaucoma is a secondary form of open-angle glaucoma produced by pigment dispersion in the anterior segment of the eye. This condition constitutes 1.0–2.5% of the glaucomas seen in many Western countries. Pigmentary glaucoma generally occurs in young adults but has been described in adolescents and older individuals as well. The disease preferentially involves men, and the women affected usually are a decade older than the men. Pigmentary glaucoma occurs most often in white individuals: it is diagnosed rarely in blacks and Asians. There is a strong association between pigmentary glaucoma and myopia: the typical pigmentary glaucoma patient is a myopic white man in his twenties or thirties. One study of black patients found that the average age of onset was 73 years, and the patients were more often hyperopic than myopic, indicating that there may be a different mechanism of disease in this subgroup. Although there have been a few reports of familial pigmentary glaucoma, most cases appear to be sporadic.
Pigmentary glaucoma is characterized by the release of pigment particles from the pigment epithelium of the iris. These particles are carried by the aqueous humor convection currents and then deposited on a variety of tissues in the anterior segment of the eye, including the corneal endothelium ( Fig. 18-1 ), trabecular meshwork ( Fig. 18-2 ), anterior iris surface, zonules ( Fig. 18-3 ), and lens. The loss of pigment from the iris is detected as a series of radial, spokelike, midperipheral transillumination defects. These defects can range in number from 1 or 2 to 65 or 70 and can be thin slits or coalescent areas. They are best seen in a darkened room by a dark-adapted observer. The defects can be highlighted by shining a small slit beam through the pupil with the light perpendicular to the plane of the iris. On rare occasions, transillumination defects are hidden by very heavy iris pigmentation. Patients with pigmentary glaucoma have very deep anterior chambers, a concave appearance of the peripheral iris, and mild iridodonesis.



The deposition of pigment on the corneal endothelium generally takes the form of a vertically oriented spindle called Krukenberg’s spindle (see Fig. 18-1 ), which can range in appearance from faint to striking. The spindle is neither pathognomonic of the disease nor invariably present, but it is a very useful sign. The spindle consists of extracellular as well as intracellular pigment granules phago-cytized by the corneal endothelium. Pigment also accumulates in the trabecular meshwork. In early cases of pigmentary glaucoma, the trabecular meshwork is moderately pigmented, with pigments varying from one portion of the meshwork to another. In advanced cases, the trabecular meshwork appears as a dark-brown velvet band that extends uniformly about the circumference of the angle (see Fig. 18-2 ). The pigment can cover the entire width of the angle from the ciliary face to the peripheral cornea; a pigment line anterior to Schwalbe’s line is often referred to as Sampaolesi’s line .
Pigment is also deposited on the zonules, posterior lens surface (Zentmayer’s ring or Scheie’s line), and anterior iris surface. The pigment on the anterior iris surface accumulates in the circumferential folds and can be sufficient to give a dull or even a heterochromic appearance if the pigment dispersion is asymmetric in the two eyes.
The anterior chamber is very deep and the peripheral iris has a concave configuration when viewed at the slit lamp or with gonioscopy ( Fig. 18-4 ). With the exception of pigmentary dispersion, pigmentary glaucoma resembles primary open-angle glaucoma (POAG) in most aspects, including elevated intraocular pressure (IOP), decreased outflow facility, optic nerve cupping, and visual field loss. Large diurnal IOP fluctuations are thought to occur more often in pigmentary glaucoma and can be sufficient to cause corneal edema, blurring, and halo vision. Patients with pigmentary glaucoma can have a sudden release of pigment with severe IOP elevations after pupillary dilation or exercise. At times, this release of pigment can be confused with active anterior segment inflammation. Pigment release and marked IOP elevation after exercise can be blocked by topical pilocarpine therapy.

Several reports have indicated that pigment dispersion lessens with time so that Krukenberg’s spindles and trabecular pigmentation become less prominent. In some cases, this is accompanied by an improvement in aqueous humor dynamics. Ritch has proposed that this disappearance of pigment may explain some non-progressive cases of normal-tension glaucoma. That is, a patient who has optic nerve cupping, visual field loss, and normal IOP may have had pigmentary glaucoma and elevated IOP in the past. Remission of pigmentary dispersion also has been reported after glaucoma surgery and lens subluxation.
The differential diagnosis of pigmentary glaucoma includes any condition that produces pigmentation of the trabecular meshwork. These include normal eyes with aging, POAG, uveitis, cysts of the iris and ciliary body, pigmented intraocular tumors, previous surgery (including laser surgery), trauma, angle-closure glaucoma, amyloidosis, diabetes mellitus, herpes zoster, megalocornea, radiation, siderosis, and hemosiderosis. These conditions should be readily distinguished from pigmentary glaucoma by the history and physical examination. The condition most likely to be confused with pigmentary glaucoma is exfoliation syndrome. However, the pattern of iris atrophy in exfoliation syndrome is usually central and geographic, and the pigment accumulation in the trabecular meshwork consists of larger particles that are unevenly distributed about the angle.
It is important to emphasize that many individuals have pigment dispersion without glaucoma or abnormal aqueous humor dynamics. Pigment dispersion syndrome (PDS) was found in 2.45% of 934 patients undergoing glaucoma screening, and is thus much more common than pigmentary glaucoma, which results from a decreased facility of outflow following pigment deposition in the trabecular meshwork in a subset of patients with PDS. Pigment dispersion syndrome can be asymptomatic, and have varying levels of expression, and is therefore likely to be overlooked or underdiagnosed. The true prevalence of PDS in the population is not known; most cases of mild pigment dispersion are probably never detected. Pigment dispersion appears to be at least as common as pigmentary glaucoma and occurs with equal frequency in both sexes. In some cases, pigment dispersion progresses to pigmentary glaucoma over time, which can be as long as 12–20 years. However, most individuals with PDS maintain normal visual fields and aqueous humor dynamics, even on long-term follow-up. This good prognosis is especially likely if the patient has a normal tonographic outflow facility when first seen. Generally the degree of pigment dispersion does not correlate well with the presence or future development of glaucoma. Patients with PDS and normal IOPs should receive a careful initial examination, including visual field examinations and optic nerve photographs. These individuals should then be followed up periodically without treatment.
Any theory about the pathogenesis of pigmentary glaucoma must explain two phenomena: pigment release and diminished outflow facility. Campbell has proposed a mechanical theory to explain pigment dispersion. He postulated that the concave shape of the peripheral iris allows it to rub against the zonules, causing pigment release and dispersion. Campbell noted that the pattern of iris transillumination defects corresponds with the arrangement of the anterior zonular packets. He also noted that the number and extent of the iris transillumination defects correlate with the progression of pigmentary glaucoma. When patients receive miotic treatment, the ensuing pupillary block lifts the peripheral iris off the zonules and allows the transillumination defects to fill in and even disappear. Lord and colleagues measured refraction, keratometry, and axial length of 13 patients with pigmentary glaucoma and 17 controls. They found that the pigmentary glaucoma and PDS patients had flatter keratometry than myopic controls, suggesting a difference in their anterior segment architecture.
Not all patients with a diagnosis of pigmentary glaucoma show iridozonular contact on ultrasound biomicroscopy. Pillunat and colleagues studied 28 eyes of 28 patients with pigmentary glaucoma and found that iridozonular contact was present in only 10. In these 10 eyes, laser peripheral iridectomy predictably relieved the characteristic reverse pupillary block, and interestingly led to a 25% decrease in IOP (from a pretreatment mean of 24.6 mmHg to a post-treatment mean of 18.3). In the 18 eyes without iridozonular contact, the pressure dropped only slightly, from 25.1 to 23.1. These findings differ from our experience in that we have had no success in lowering pressures in patients with pigmentary glaucoma and ultrasonically confirmed iridozonular contact. Perhaps iridectomy may help during phases of the syndrome when pigment granules are being liberated actively, but iridectomy seems unlikely to benefit established cases when the damage has been done. Richter and colleagues observed active pigment dispersion in 31 of 55 PDS and pigmentary glaucoma patients followed for between 6 and 43 months (mean 27 months). Active pigment dispersion was defined as an increase in transillumination, an increase in corneal pigmentation, or the presence of pigment granules on the surface of the lens in the pupil. There were no differences in frequency of active dispersion or worsening of glaucoma in patients less than 44 years old, between 45 and 64, or over 65. Although we have not seen pressure lowering in pigmentary glaucoma patients following laser peripheral iridectomy, iridectomy may have prevented or limited future pressure rise.
In addition to the mechanical theory of pigment dispersion, Anderson and colleagues found that DBA/2J(D2) mice develop a form of pigmentary glaucoma involving pigment dispersion and iris stromal atrophy. Using high-resolution mapping techniques, sequencing, and functional genetic tests, they showed that these conditions resulted from mutations in genes encoding melanosomal proteins. They postulate that pigment production and mutant melanosomal protein genes may contribute to human pigmentary glaucoma. Further study is needed to confirm this hypothesis.
When iris pigment is infused into animal or enucleated human eyes, outflow facility decreases and IOP increases. Repeated infusions of pigment, however, do not produce chronic glaucoma in animal eyes. Some authorities believe that patients with pigmentary glaucoma must have an underlying developmental abnormality of the outflow channels. As evidence for this theory, they cite (1) the high prevalence of prominent iris processes in patients with pigmentary glaucoma; (2) patients with pigmentary glaucoma who have angles that resemble infantile glaucoma, and (3) families who have some members with pigmentary glaucoma and other members with congenital glaucoma.
Other authorities propose that pigmentary glaucoma is a variant of POAG. These investigators point to families that have members with both pigmentary glaucoma and POAG. However, patients with pigmentary glaucoma do not resemble those with POAG when corticosteroid testing is considered.
Histopathologic examination of specimens from eyes with pigmentary glaucoma demonstrates pigment and debris in the trabecular meshwork cells. With advanced disease, the trabecular cells degenerate and wander from their beams, allowing sclerosis and eventual fusion of the trabecular meshwork. Some propose that excessive phagocytosis of foreign material damages the trabecular endothelial cells and causes them to migrate.
The treatment of pigmentary glaucoma resembles that of POAG in that the usual progression is from medical therapy to argon laser trabeculoplasty (ALT) to filtering surgery. β-Adrenergic antagonists, adrenaline (epinephrine), dipivefrin, and carbonic anhydrase inhibitors (CAIs) are useful in the management of pigmentary glaucoma. Miotic agents reduce IOP in pigmentary glaucoma and are theoretically appealing because they increase pupillary block and lift the peripheral iris from the zonules. However, cholinergic drugs are generally poorly tolerated by these young patients. Some reports also indicate that patients with pigmentary glaucoma have a high incidence of retinal detachment; thus a careful peripheral retinal examination is mandatory before cholinergic agents can be prescribed. Thymoxamine, an α-adrenergic antagonist, might be useful in this situation because it constricts the pupil without inducing a myopic shift in refraction.
The most intriguing therapy for pigmentary glaucoma is peripheral iridectomy to cure the ‘reverse’ pupillary block that is responsible for the characteristic peripheral iris concavity. Ultrasonic biomicroscopy is helpful in indicating those eyes that are most likely to benefit from iridectomy. In eyes with deep peripheral concavity, the effects of peripheral iridectomy are almost immediate. Within seconds of completing the iridectomy the peripheral iris moves forward and assumes a more normal configuration ( Fig. 18-5 ). The long-term effects of peripheral iridectomy are unclear. Ideally peripheral iridectomy would provide effective prophylaxis for patients with PDS before they develop glaucomatous visual field loss. Although it is impossible to determine exactly which patients with pigmentary dispersion syndrome will develop glaucoma, it may be most appropriate to treat patients at the first sign of significant IOP elevation. Patients who show elevation following exercise may also be good candidates for peripheral iridectomy. Pilocarpine and other miotics can reduce exercise-induced pressure rises, but parasympathetics routinely cause significant ocular side effects in the young adults most likely to have pigmentary glaucoma. Peripheral iridectomy has the same beneficial effects and is well tolerated by patients of all ages.


If medical management does not control IOP, ALT should be performed. Because of the heavy pigmentation of the angle, ALT is done with relatively low energy settings in the range of 200–600 mW. Selective laser trabeculoplasty also works.
Many individuals with pigmentary glaucoma eventually require filtering surgery. Despite the young age of these patients, the results of surgery are generally successful.
As mentioned previously, pigment dispersion may diminish with age. Some patients require less medical therapy and eventually discontinue therapy as they reach 60 or 70 years of age. This does not occur invariably, however, and some individuals with pigmentary glaucoma require life-long treatment.
There have been several reports of pigment dispersion and secondary glaucoma from posterior chamber intraocular lenses (IOLs). This subject is discussed in the section on glaucoma after cataract surgery, p. 273.
EXFOLIATION SYNDROME (PSEUDOEXFOLIATION SYNDROME)
Exfoliative syndrome (previously or classically known as pseudoexfoliation syndrome) occurs when several ocular tissues synthesize an abnormal protein. This protein may obstruct the trabecular meshwork and cause glaucoma. Exfoliation syndrome and its associated glaucoma are known by a variety of other names, including pseudoexfoliation, senile exfoliation, senile uveal exfoliation, glaucoma capsulare, and iridociliary exfoliation. At one time, most cases of exfoliation syndrome were diagnosed in Scandinavia. Although it is now clear that this condition occurs throughout the world, some areas seem to have a higher prevalence of the disease than do others, with particularly high rates in Scandinavia and parts of Africa. It is, however, difficult to compare the published prevalence of exfoliation syndrome precisely because various studies used different techniques and definitions and did not match the patients for age. The prevalence of exfoliation syndrome is closely linked to age, reaching a maximum in the seventh to ninth decades of life. The prevalence of exfoliation syndrome in the Framingham study was 0.6% in patients younger than 65 years of age, 2.6% in patients 65–74 years of age and 5.0% in patients 75–85 years of age. Others have reported that exfoliation syndrome occurs in 3–28% of patients with open-angle glaucoma in the United States, with most estimates in the range of 3–10%. In the Blue Mountains Eye Study, the exfoliation syndrome prevalence was 2.7% of the entire population of 3645 that participated. The prevalence of glaucoma among exfoliation syndrome patients was 14.7%. In contrast, in some areas of Scandinavia, more than 50% of patients with open-angle glaucoma have evidence of exfoliation. Exfoliation syndrome appears to be common among Russian immigrants to the U.S.A.
Exfoliation syndrome is more common in women than in men, but the combination of exfoliation syndrome and glaucoma occurs equally in both sexes. Most cases appear to be sporadic, but Allingham and associates found a series of Icelandic families in which there appears to be an X-linked inheritance pattern. In their study, Allingham and colleagues identified six Icelandic families that met the entry criteria of having at least three members over 70 years of age, at least one of whom had exfoliation syndrome. In each of these families, at least one person in the next generation had pseudoexfoliation and glaucoma. In all cases in which a parent and child were found to have exfoliation syndrome, the parent was always the mother.
Recently, a polymorphism in exon 1 of the LOXL1 gene has been found to be highly associated with exfoliative syndrome in Iceland and in Sweden where the prevalence of exfoliative syndrome is very high. The same polymorphisms have been confirmed in populations with exfoliative syndrome in other parts of Europe, India, the United States, Australia, and Japan. These same polymorphisms are NOT associated with POAG and appear to be specific for exfoliative syndrome and glaucoma. Unfortunately, the polymorphisms can not be used for diagnostic purposes since they appear quite frequently in the ‘normal’ population. Whether these polymorphisms in normal individuals are truly markers for future development of exfoliative syndrome or glaucoma or whether some other genetic or environmental facilitator must be present to generate the syndrome remains unknown at this time. That some ‘second hit’, either genetic or environmental, must be present is hinted at by the study of Hewitt and co-workers which suggests that Australians of European extraction have a much lower penetrance from the polymorphisms than in the Nordic peoples. The LOXL1 family of proteins is associated with extracellular matrix formation and stability so it is not surprising that formation of abnormal proteins by genetic variants might affect trabecular outflow and the lens epithelium, among other structures. Inhibitors of matrix metalloproteinases have been found to be upregulated and actual matrix metalloproteins have been found to be downregulated in the aqueous humor of eyes with exfoliative syndrome compared to eyes without.
One-third to one-half of the cases of exfoliation syndrome are unilateral at detection, but 14–43% of these cases become bilateral over 5–10 years. The prevalence of glaucoma in exfoliation syndrome is reportedly 0–93%. However, many of these studies diagnosed glaucoma on the basis of elevated IOP or even abnormal provocative tests. Using strict definitions, recent studies detected glaucoma in 6–7% of patients with exfoliation syndrome and detected elevated IOP in an additional 15%. In patients who had exfoliation syndrome and normal IOPs at diagnosis, glaucoma developed in 3–15% over 3–15 years. In the Ocular Hypertension Treatment Study, the rate of new glaucoma among untreated ocular hypertensive patients was approximately 2% per year.
Exfoliation syndrome is not associated with any known systemic disorder. However, a retrospective study suggests that patients with exfoliative syndrome have a higher risk of developing acute cerebrovascular diseases and chronic cerebral conditions such as Alzheimer disease than those with POAG. However, this same group was unable to show any overall increase in mortality associated with exfoliative syndrome except as related to use of acetazolamide.
Another study showed higher levels of plasma homocysteine in exfoliative patients compared to those with normal-tension glaucoma. Increased levels of plasma homocysteine have been associated with increased risk of cardiovascular disease. It is clear that prospective longitudinal studies are needed to determine if these associations noted in retrospective and cross-sectional studies are truly clinically important.
The clinical presentation of exfoliation syndrome includes a classic pattern on the anterior lens surface consisting of a central translucent disc surrounded by a clear zone, which in turn is surrounded by a granular grey-white ring with scalloped edges ( Fig. 18-6 ). This is best appreciated when the lens is examined with the slit lamp after pupillary dilation; if the pupil is not dilated, many cases can be missed because the characteristic ring may not be visible within a small pupil. The central and peripheral zones can be entirely separate or can be joined by bridges of material. It has been postulated that the movement of the central iris polishes the lens and produces the clear zone. In some cases, the central disc is not present. The peripheral zone may have radial striations and raised edges. Dandruff-like flakes of exfoliation material are deposited on the corneal endothelium, trabecular meshwork, anterior and posterior iris, pupillary margin, zonules, and ciliary processes as well as the anterior hyaloid face in aphakic eyes. The peripupillary iris has an irregular, moth-eaten pattern of transillumination. This is often the finding that alerts the examiner to the possibility of exfoliation syndrome. The pigment released from the iris is deposited in the trabecular meshwork, in the anterior iris, and to a lesser extent on the corneal endothelium. The angle pigmentation is moderate to heavy in amount and somewhat patchy in distribution. A wavy pigmented line (Sampaolesi’s line) may be seen anterior to Schwalbe’s line. When the pupil is dilated, a shower of pigment may be released. Fluorescein angiography of the iris reveals a decreased number of vessels, neovascularization, and leakage from the vessels that remain.

The treatment of glaucoma associated with exfoliation syndrome is similar to that of POAG. Intraocular pressure is usually higher in exfoliation syndrome, however, and the response to medical treatment is less favorable. Argon laser trabeculoplasty has its greatest pressure-lowering effect in exfoliation syndrome. Somewhat paradoxically, however, the ultimate success rate of ALT in patients with exfoliation syndrome may be lower than that in other conditions because the initial pressures are higher and because adjunctive medical therapy is less effective. Further, in many successfully treated eyes, the IOP rises again in 12–36 months. Filtering surgery has a high rate of success in this condition. Jacobi and colleagues have suggested trabecular aspiration, a non-filtering procedure performed with a suction canula, to clear debris from the trabecular meshwork in cases of exfoliation syndrome. Short-term follow-up has been encouraging, but longer term follow-up has not been encouraging; a larger multicenter trial will be necessary to determine if this procedure is a viable alternative to intensive conventional therapy. Several authors reported that eyes with exfoliation syndrome have fragile zonules and a greater incidence of zonular rupture and vitreous loss during cataract surgery.
On microscopy the exfoliation material appears as a fibrillar protein arranged in an irregular meshwork. Evidence of exfoliation syndrome is widely distributed in the ocular tissues and may affect the rigidity of the lamina cribrosa. This material has been compared to zonules, basement membrane, microtubular constituents, and amyloid. At one time, many thought that all of the exfoliation material came from the lens capsule and epithelium. However, the material has a multifocal origin and has been found in the iris, ciliary epithelium, and conjunctiva as well as in ocular and orbital blood vessels. Exfoliative material has been found in the skin of the eyelid in patients with the syndrome.
Schlotzer-Schredhardt and associates found an abnormal relationship between matrix metalloproteinases (MMPs) and their endogenous inhibitors, tissue inhibitor of metalloproteinases (TIMPs). They postulate that this abnormal relationship leads to the development and precipitation of the exfoliative material in exfoliation syndrome. Pseudoexfoliation syndrome with glaucoma appears to be a secondary glaucoma in which exfoliation material and pigment obstruct the trabecular meshwork. Ho and colleagues confirmed the finding of inhibitors of matrix metalloproteinases in the aqueous humor of eyes with exfoliation syndrome. They conclude that there is downregulation of proteolytic activity in the trabecular meshwork leading to clogging. A few authorities have postulated an underlying defect of the outflow channels and supported this theory by finding a few patients with unilateral exfoliation and bilateral glaucoma. However, many patients with apparent unilateral disease have bilateral pseudoexfoliation that can be demonstrated by conjunctival biopsy. In addition, most patients with unilateral pseudoexfoliation syndrome have more abnormal aqueous humor dynamics in the affected eye. Finally, patients with exfoliation syndrome and glaucoma resemble the normal population rather than patients with POAG in their corticosteroid responsiveness. One investigator has postulated a developmental defect in eyes with pseudoexfoliation syndrome and glaucoma, but this has not been confirmed.
CORTICOSTEROID GLAUCOMA
Corticosteroid administration can produce a clinical picture that closely resembles that of POAG, with elevated IOP, decreased outflow facility, open angles, and eventually optic nerve cupping and visual field loss. Classic studies by Armaly and Becker indicate that 5–6% of normals develop marked IOP rises after 4–6 weeks of topical dexamethasone or betamethasone administration. However, an even higher percentage of normal individuals develop substantially increased IOPs if the glucocorticoid is administered in greater frequency, at higher doses or for a longer period. Certain groups in the population are particularly susceptible to the pressure-raising effects of glucocorticoids. These groups include patients with POAG, their first-degree relatives, diabetic patients, and highly myopic individuals.
Patients who experience a transient or sustained pressure rise after corticosteroid instillation are referred to as steroid responders; if glaucomatous damage ensues as manifested in the optic nerve or on visual field testing, then they truly can be said to have steroid glaucoma. Steroid-induced ocular hypertension would be a more accurate designation in most cases.
Most cases of corticosteroid ocular hypertension or glaucoma are caused by drops or ointments instilled in the eye for therapeutic purposes. However, glucocorticoid creams, lotions, and ointments applied to the face or eyelids may reach the eye in sufficient quantity to raise IOP, as may systemically-administered corticosteroids. This latter category includes glucocorticoids used topically on the skin that may be absorbed and produce systemic and ocular effects.
In addition, periocular corticosteroid injections, especially those involving repository or ‘depot’ preparations, are capable of raising IOP. Sometimes, the IOP rise occurs months after the injection; if not monitored, optic nerve damage could occur. If IOP elevation persists and/or optic nerve damage does occur following a periocular injection of depot corticosteroid, removal of the repository of periocular steroid often allows the IOP to return to non-dangerous levels.
Recently, intravitreal injection of triamcinolone acetonide has become popular as a treatment for diabetic macular edema, macular edema associated with retinal vein occlusion, and various posterior pole inflammatory diseases. Approximately 30% of eyes having this treatment will show a transient IOP rise. In some patients, the IOP rise persists and may require topical medication, laser trabeculoplasty or even trabeculectomy to lower the pressure and prevent optic nerve damage or progression of optic nerve damage. Patients with pre-existing glaucoma, younger age, increased dose of triamcinolone, higher baseline IOP, uveitis, and repeat injections are risk factors for elevated IOP in the post-injection period.
There have been several case reports of increased IOP following use of a corticosteroid inhaler for asthma, and we have seen at least two cases in which intractable IOP elevation followed the use of a corticosteroid nasal spray for allergic rhinitis. Corticosteroids used in nasal sprays get into the bloodstream in sufficient quantities over the long term to be associated with central serous retinopathy in susceptible patients; it is reasonable to assume that something similar happens in those susceptible to steroid-induced elevated IOP. In rare cases, glaucoma is produced by endogenous glucocorticoids associated with adrenal hyperplasia or adenoma.
The rise in IOP from corticosteroids may occur within a week of initiating treatment or may be delayed for years. Patients undergoing long-term glucocorticoid treatment must be examined periodically because no time limit exists for this problem. Tragically, many cases of corticosteroid glaucoma are produced by treatment for trivial conditions such as contact lens discomfort or red eyes. In part because of their widespread availability and general effectiveness, combination steroid–antibiotic eyedrops are prescribed routinely by the general medical community for red eyes. In many such cases, the IOP is neither measured nor followed. The overwhelming majority of patients tolerate a short course of these medications quite well, but a small percentage are unusually susceptible to steroid-induced pressure elevation. Other patients are inappropriately allowed to refill steroid drops for an extended period of time. These patients are at significant risk of developing a dangerous elevation of IOP.
There have been several reports of moderate to severe IOP elevation in patients treated with corticosteroid eyedrops following laser-assisted in-situ keratomileusis (LASIK). The elevated pressure can cause vision-threatening corneal opacity. These cases can be particularly challenging because of the difficulty in obtaining an accurate measurement of the true IOP in the postoperative period (or, in fact, anytime after LASIK because of the subsequent very thin cornea).
As stated, corticosteroid glaucoma usually resembles POAG. However, the clinical picture may be altered by the age of the patient. Infants treated with corticosteroids may develop a condition that resembles congenital glaucoma. In contrast, elderly patients who received corticosteroid treatment in the past may have normal-tension glaucoma. The clinical picture of corticosteroid glaucoma may also be confounded by the presence of other ocular diseases. For example, a patient with shallow anterior chambers and corticosteroid glaucoma may appear to have chronic angle-closure glaucoma.
Glucocorticoids raise IOP by lowering outflow facility through an unknown mechanism. The most common explanation for this phenomenon has been that glucocorticoids cause an accumulation of glycosaminoglycans in the trabecular meshwork, perhaps by stabilizing lysosomal membranes and inhibiting the release of catabolic enzymes. Cultured human trabecular cells secrete a wide variety of substances that contribute to the extracellular matrix. Treating cultured trabecular cells with steroid induces the secretion of elastin, which may have a role in trabecular obstruction in vivo . Other explanations for corticosteroid glaucoma include an inhibition of the phagocytosis of foreign matter by trabecular endothelial cells and decreased synthesis of prostaglandins that regulate aqueous humor outflow. Southren and co-workers and Weinstein and co-workers found abnormal glucocorticoid metabolism in trabecular tissue from patients with POAG. This finding may explain the increased susceptibility of patients with POAG to the ocular hypertensive effects of glucocorticoids. Alternatively, if steroids cause changes that would tend to result in a reduced outflow facility in most or all human eyes, those individuals with marginal or compromised outflow facility to begin with would be expected to show the greatest rise in IOP.
Cultured human trabecular meshwork cells secrete increased amounts of laminin and integrin when exposed to dexamethasone, and a similar mechanism may be operative in vivo . Other changes include thickening of the trabecular beams, alteration in F-actin architecture, increased cross-linked actin and induction of myocilin protein. Which of these changes plays a role in the corticosteroid-induced elevation of IOP is unknown.
The first step in managing corticosteroid glaucoma is to discontinue the drug. In most cases, IOP returns to normal over a few days to several weeks. During this period, antiglaucoma medications may be used to control IOP. If medication is unsuccessful in controlling IOP and the optic nerve is threatened, laser trabeculoplasty or filtering surgery should be considered. Caution should be exercised, however, before performing an irreversible procedure in what is usually a time-limited condition. It may be most appropriate to confirm progression in glaucomatous damage on maximal tolerated medical therapy before operating.
If glucocorticoid treatment is necessary for the patient’s life or well-being, therapy should be altered to the weakest possible drug at the lowest possible dose. The residual glaucoma is then treated in the same fashion as is POAG. In the cases that require topical ocular corticosteroid therapy, the patient should be treated if possible with drugs such as medrysone or fluorometholone because these drugs have less of a tendency to raise IOP.
In rare cases, IOP remains elevated months to years after the corticosteroid has been discontinued. In these situations, it may be impossible to determine whether this is a residual effect of glucocorticoid treatment or whether the patient has had underlying open-angle glaucoma unmasked by the treatment. In either case, the patient is treated in similar fashion. If IOP is elevated because of a periocular glucocorticoid injection and medical therapy is unsuccessful in controlling the pressure and protecting the optic nerve, the repository material should be excised.
Topical corticosteroids can increase IOP even in the face of a functioning filtering bleb or tube-shunt procedure (glaucoma drainage device). Therefore, if IOP rises in the mid to late postoperative period, corticosteroid use should be discontinued before assuming that the glaucoma procedure has failed.
LENS-INDUCED GLAUCOMA
There are a variety of lens-induced glaucomas. In the past, great controversy surrounded the pathogenesis and nomenclature of these disorders. This chapter uses the following classification system:
- 1.
Phacomorphic glaucoma: a swollen lens causes increased pupillary block and secondary angle closure.
- 2.
Dislocated lens: a dislocated lens causes increased pupillary block and secondary angle closure.
- 3.
Phacolytic glaucoma: lens protein leaks from an intact cataract and obstructs the trabecular meshwork.
- 4.
Lens-particle glaucoma: lens material liberated by trauma or surgery obstructs the outflow channels.
- 5.
Phacoanaphylaxis: sensitization to lens protein produces granulomatous inflammation and occasionally secondary glaucoma.
Phacomorphic glaucoma and dislocated lens are discussed in Chapter 15 . The remainder of this section is devoted to the other three conditions.
PHACOLYTIC GLAUCOMA
Lens protein is normally sequestered within the lens capsule. With age and the development of cataract, the protein composition of the lens is altered to components with heavier molecular weight. If these soluble molecules leak through what grossly appears to be an intact capsule, they can obstruct the trabecular meshwork. The lens protein also stimulates inflammation and a macrophage response. The macrophages engulf the lens protein and may further obstruct the outflow channels ( Fig. 18-7 ). Protein of heavy molecular weight is not seen in infants and children, possibly explaining the absence of phacolytic glaucoma in young patients with cataract.

Phacolytic glaucoma is usually seen in older patients, who usually have a history of poor vision in the eye for months or years. The patients we have seen with this condition have often been from areas with little access to health care such as inner cities or developing nations. The disease typically appears with an acute onset of monocular pain, redness, and perhaps a further decrease in vision. Examination reveals a severe IOP elevation, corneal edema, ciliary injection, open angles, and heavy cell and flare. The cells appear larger than white blood cells and somewhat iridescent. The cells may precipitate on the corneal endothelium, but no true keratic precipitates or hypopyon is seen. Ultrastructural analysis of aqueous humor and trabeculectomy specimens in phacolytic glaucoma have revealed melanin-laden macrophages, red blood cells (RBCs), ghost RBCs, macrophages showing erythrophagocytosis, and free cell debris in addition to the lens material-laden macrophages that are traditionally associated with this condition. The flare may be so heavy that the aqueous humor appears yellow. An important physical finding is the appearance of white particles on the anterior lens surface and in the aqueous; these particles are thought to be cellular aggregates or clumps of insoluble lens protein. Visual acuity is reduced in this condition, sometimes to the level of inaccurate light perception. The lens has a mature, hypermature, or even Morgagnian cataract ( Fig. 18-8 ). Rarely this disease is produced by an immature cataract with a zone of liquefied cortex.

On rare occasions, phacolytic glaucoma has a subacute course, with intermittent leakage of protein producing recurrent episodes of glaucoma, hyperemia, and inflammation. This appearance is more likely if the cataract has been dislocated into the vitreous. The diagnosis of phacolytic glaucoma is usually made on clinical grounds. If the diagnosis is in doubt, an anterior chamber paracentesis should be performed to detect macrophages engorged with lens material. Aqueous humor is examined by phase-contrast microscopy or Millipore filtration and staining.
Cataract extraction is the definitive treatment for phacolytic glaucoma. Before surgery, IOP and inflammation should be reduced by medical treatment, including hyperosmotic agents, topical adrenergic agents, CAIs, cycloplegic drugs, and topical corticosteroids. Traditionally most surgeons have removed these cataracts by an intracapsular technique. Microscopic examination of the lens reveals characteristic calcium oxalate crystals ( Figs 18-9 and 18-10 ). Because the lens capsule is quite fragile, a sector iridectomy and α-chymotrypsin are employed. If the capsule ruptures during delivery,the anterior chamber should be irrigated copiously to remove any residual protein. Other surgeons have used extracapsular cataract extraction in patients with this condition with good results. Because of the friability of the zonules and the brittleness of the capsule, the anterior capsulorrhexis may be performed by Vannas scissors or some other means that minimizes zonular and capsular stress. Lens delivery and residual cortical aspiration are also performed in an unusually delicate manner. In successful cases, posterior chamber IOL placement is possible and yields excellent results. As the last generation of surgeons trained in intracapsular surgery ages, the extracapsular technique will most likely replace the intracapsular approach. Regardless of approach, most patients have good visual acuity postoperatively and total remission of the glaucoma.


If phacolytic glaucoma is caused by a dislocated lens, the lens should be removed by vitrectomy instruments. Occasionally a dislocated lens can be floated into the anterior chamber with a stream of irrigation fluid and then removed through a limbal incision.
In the rare situation when phacolytic glaucoma is caused by an immature cataract and the eye has good vision, attempts should be made to control IOP and inflammation by medical means. If this fails, the lens should be removed.
LENS-PARTICLE GLAUCOMA
Disruption of the lens capsule by penetrating trauma or surgery liberates lens material, which can obstruct the trabecular meshwork. The resulting glaucoma depends on the amount of lens material liberated, the inflammatory response of the eye, and the ability of the trabecular meshwork to clear the foreign matter. Generally the glaucoma has its onset a few days after the precipitating event. In rare cases, the lens material can be released long after surgery or trauma.
Patients with lens-particle glaucoma usually have significant pain, redness, and decreased vision. Examination reveals corneal edema, elevated IOP, open angles, heavy cell and flare, and chunky white particles in the aqueous humor. A hypopyon may be present, as may fluffy cortical material. If the condition has existed for some time, peripheral anterior synechiae and posterior synechiae may be present.
Generally the diagnosis of lens-particle glaucoma is suggested by the sequence of events. It is more difficult to diagnose delayed cases or cases with spontaneous rupture of the lens capsule, which may be confused with phacolytic glaucoma, phacoanaphylactic glaucoma, or other conditions. Anterior chamber paracentesis in this condition reveals macrophages and free lens material.
The elevated IOP and inflammation associated with lens-particle glaucoma are treated medically in the same fashion as phacolytic glaucoma. If this is not adequate, the residual lens material should be removed. Although fluffy cortical material can be aspirated easily, it is more difficult to remove solid lens material trapped in membranes behind the iris. Sometimes the entire lens mass can be teased from the eye after intracameral infusion of α-chymotrypsin. Lens particles in the vitreous can be removed with vitrectomy instruments. Surgery should not be delayed unduly in this disease or complications may occur, including posterior synechiae, peripheral anterior synechiae, cystoid macular edema, retinal detachment, and corneal decompensation.
PHACOANAPHYLAXIS
Phacoanaphylaxis is an uncommon condition that is thought to occur when patients become sensitized to their own lens protein. Phacoanaphylaxis typically develops after penetrating trauma or extracapsular cataract extraction. Histopathologically, these eyes have a granulomatous inflammation of the lens with polymorphonuclear leukocytes, lymphocytes, epithelioid cells, and giant cells. Occasionally the inflammation involves the trabecular meshwork and leads to a rise in IOP.
Phacoanaphylaxis is treated with medication to reduce inflammation and control IOP. If this is unsuccessful, residual lens material should be removed.
GLAUCOMA AFTER CATARACT SURGERY
Elevated IOP often occurs after cataract surgery through a variety of mechanisms ( Box 18-1 ). Many of these clinical problems are presented elsewhere, so the discussion here is restricted to the entities that are peculiar to cataract surgery.
- I.
Open-angle glaucoma
- A.
Early onset (within first postoperative week)
- 1.
Pre-existing chronic open-angle glaucoma
- 2.
α-Chymotrypsin-induced glaucoma
- 3.
Hyphema/debris
- 4.
Viscoelastic material
- 5.
Idiopathic pressure elevation
- 1.
- B.
Intermediate onset (after first postoperative week)
- 1.
Pre-existing chronic open-angle glaucoma
- 2.
Vitreous in the anterior chamber
- 3.
Hyphema
- 4.
Inflammation
- 5.
Lens particle glaucoma
- 6.
Corticosteroid-induced glaucoma
- 7.
Ghost-cell glaucoma
- 1.
- C.
Late onset (more than 2 months postoperatively)
- 1.
Pre-existing chronic open-angle glaucoma
- 2.
Ghost-cell glaucoma
- 3.
Neodymium:yttrium-aluminum-garnet (Nd:YAG) laser capsulotomy
- 4.
Vitreous in the anterior chamber
- 5.
Late-occurring hemorrhage
- 6.
Chronic inflammation
- 1.
- A.
- II.
Angle-closure glaucoma
- A.
With pupillary block
- 1.
Anterior hyaloid face
- 2.
Posterior lens capsule
- 3.
Intraocular lens
- 4.
Posterior synechiae
- 5.
Silicone oil
- 1.
- B.
Aqueous misdirection (malignant glaucoma)
- C.
Without pupillary block
- 1.
Pre-existing angle-closure glaucoma
- 2.
Inflammation/hyphema
- 3.
Prolonged anterior chamber shallowing
- 4.
Iris incarceration in cataract incision
- 5.
Intraocular lens haptics
- 6.
Neovascular glaucoma
- 7.
Epithelial ingrowth
- 8.
Fibrous ingrowth
- 9.
Endothelial proliferation
- 10.
Proliferation of iris melanocytes across the trabecular meshwork
- 1.
- A.
Modified from Tomey KF, Traverso CE: Glaucoma associated with aphakia and pseudophakia. In: Ritch R, Shields MB, Krupin T: The glaucomas, 2nd edn, St Louis, Mosby, 1996.
A transient rise in IOP has been reported in 33% to almost 100% of eyes after cataract extraction, depending on the method of extraction and the surgeon involved. This pressure rise may be undetected because it occurs several hours after surgery, and the pressure may return to near-normal levels by the next morning or whenever the patient is seen for the first postoperative visit. The ocular hypertension may be sufficient to cause pain, nausea and vomiting, corneal edema, and optic nerve damage, especially in patients with pre-existing glaucoma. The elevated IOP usually abates spontaneously over 2–4 days. During this period the patients are treated with topical and systemic antiglaucoma medications, including hyperosmotic agents as needed to control IOP. The mechanism of the IOP rise appears to be complex and includes the following:
- 1.
Inflammation with the release of active substances, including prostaglandins and the formation of secondary aqueous humor.
- 2.
A watertight wound closure with multiple fine sutures limiting the ‘safety valve’ leak of aqueous humor.
- 3.
Deformation of the limbal area, reducing trabecular outflow. On gonioscopy, Kirsch and co-workers noted a white ridge internal to limbal cataract wounds. This ridge, attributed to tight sutures and to operative edema and swelling, is associated with reduced trabecular function.
- 4.
Obstruction of the trabecular meshwork by pigment, blood, lens particles, inflammatory cells, and viscoelastic substances.
α-CHYMOTRYPSIN GLAUCOMA
α-Chymotrypsin fragments zonules and was used widely to facilitate intracapsular cataract extraction. However, elevated IOP often occurred within 1–5 days after using this drug. When examined, these eyes had open angles, decreased outflow facility, and increased IOP, which could be sufficient to cause corneal edema, wound disruption, and optic nerve damage. This entity is self-limited, lasting for 2–4 days and leaving no permanent abnormalities of aqueous humor dynamics.
The generally accepted mechanism for α-chymotrypsin glaucoma is that zonular fragments obstruct the outflow channels. This theory is supported by scanning electron microscopy, which shows zonular fragments in the trabecular meshwork, and by experimental studies in monkeys ( Fig. 18-11 ). A few investigators have proposed alternative mechanisms for the glaucoma, including inflammation or a direct toxic effect on the meshwork, but direct infusion of α-chymotrypsin into the anterior chamber of monkeys actually produced an increase in outflow facility. During the period of elevated IOP, patients are treated with topical and systemic glaucoma medications as needed. Patients with pre-existing optic nerve damage must be watched very closely because the pressure may rise to extremely high levels. The incidence and severity of the pressure rise can be reduced by using a lesser concentration of the drug (1:10 000 instead of 1:5000) in a lower volume (0.25–0.5 ml instead of 2 ml). The anterior chamber should be irrigated before lens extraction to remove zonular fragments. Prophylactic treatment with a variety of agents has generally failed to block this IOP rise, although one group found prophylactic timolol and acetazolamide useful in this situation. α-Chymotrypsin-associated IOP elevation is seen quite rarely today because intracapsular cataract extraction with α-chymotrypsin is rarely performed.

GLAUCOMA FROM VISCOELASTIC SUBSTANCES
Viscoelastic substances often are employed in cataract surgery to protect the corneal endothelium and to facilitate intraocular lens (IOL) insertion. Sodium hyaluronate, the agent with perhaps the widest clinical use, frequently causes marked postoperative IOP elevations. When examined, these eyes demonstrate elevated IOP, deep anterior chambers, and corneal edema. Cellular and particulate matter in the aqueous humor may appear suspended and almost immobile if large amounts of viscoelastic remain in the anterior chamber, but IOP elevations may occur even in the absence of clinically detectable viscoelastic. It is sometimes possible to see tiny ruby-like globs of hemorrhage on the iris surface or suspended in the anterior chamber. These isolated blood droplets indicate the presence of retained viscoelastic. The IOP reaches a maximum in 12–16 hours and then abates spontaneously over the next 72 hours. During this period, patients are treated with topical and systemic glaucoma medications and hyperosmotic agents as needed to control IOP. The postoperative IOP rise can apparently be limited by removing as much of the sodium hyaluronate as possible at the end of the surgery, although some studies have failed to show a significant difference in IOP between eyes that have had the viscoelastic removed and those in which it was left in place at the end of surgery. Because it is difficult to standardize all of the variables that can affect the pressure rise in a given patient, it has been difficult to determine the effectiveness of various prophylactic treatment regimens on preventing the postoperative IOP rise seen after viscoelastic use. Some of these variables include the viscoelastic agent used, the surgeon, the surgical technique, the completeness of viscoelastic removal at the end of the procedure, and the rate at which the patient’s eye clears itself of retained viscoelastic.
It is postulated that viscoelastic substances obstruct the trabecular meshwork. This theory is supported by experiments showing that sodium hyaluronate causes elevated IOP in animal eyes and reduces outflow facility in enucleated human eyes. The latter is reversed by intracameral infusion of hyaluronidase. Hyaluronidase is normally present in the anterior segment of the eye but not in sufficient quantity to metabolize the large volume of sodium hyaluronate infused at surgery, at least in the short haul. Obstruction of the trabecular meshwork depends on more than the drug’s viscosity because low-viscosity sodium hyaluronate produces a greater rise in IOP in monkey eyes than does high-viscosity sodium hyaluronate.
Other viscoelastic substances have been evaluated for cataract surgery. Viscoat (a mixture of sodium hyaluronate and sodium chondroitin sulfate) produced similar IOP increases in one study, and marginally higher pressures in another. Chondroitin sulfate and methylcellulose may be less likely to elevate IOP.
GLAUCOMA WITH PIGMENT DISPERSION FROM INTRAOCULAR LENSES
Pigment dispersion and elevated IOP have been described with posterior chamber IOLs and, in a few cases, with iris fixation lenses. In most instances, the lenses have been decentered, tilted, excessively mobile, too small, or reversed in position, creating excess friction between the optic or haptic and the iris pigment epithelium. The pigment epithelial loss is geographic, and the iris may have a grey atrophic appearance. The pigment particles rubbed off the iris accumulate in the trabecular meshwork, where they obstruct aqueous humor outflow in a manner analogous to pigmentary glaucoma. Intraocular pressure rises days to months after cataract surgery but may improve spontaneously.
Patients with this entity are responsive to standard medical treatment for glaucoma and to ALT. In some cases, pupillary dilation or constriction may reduce the pigment dispersion. If IOP cannot be controlled, the IOL should be replaced or stabilized. This entity can be distinguished from pigmentary glaucoma by the temporal relation to cataract surgery, the unilateral state, and the absence of a typical Krukenberg’s spindle and radial transillumination defects.
UVEITIS-GLAUCOMA-HYPHEMA SYNDROME
The uveitis-glaucoma-hyphema (UGH) syndrome has been described with iris-supported, with posterior chamber, and most often with anterior chamber IOLs. The patients affected by this syndrome often have a history of uncomplicated cataract extraction but then develop elevated IOP, iridocyclitis, and recurrent hyphemas weeks to months after surgery ( Fig. 18-12 ). Ultrasonic biomicroscopy and aqueous humor analysis can aid in confirming the diagnosis. The patients may complain of recurrent episodes of extreme blurring (whiteout), presumably related to the hyphemas. The UGH syndrome is caused by excessive chafing of the iris by the pseudophakos because the lenses are too mobile, are poorly designed, or have poor finishing characteristics. Most of the lenses removed from these eyes show sharp or serrated edges capable of traumatizing iris tissue. Although some patients with a mild form of UGH syndrome respond to corticosteroid treatment and standard antiglaucoma therapy, in most cases the lens should be removed to prevent corneal decompensation and optic nerve damage. In a report of 101 IOLs explanted for a variety of reasons, patients with UGH syndrome had relatively poor visual outcomes, with 7 of 9 patients achieving a final visual acuity of less than 20/200. However, explantation did result in a decrease in pain and inflammation and better glaucoma control.


GLAUCOMA AFTER NEODYMIUM:YTTRIUM-ALUMINUM-GARNET LASER POSTERIOR CAPSULOTOMY
Intraocular pressure often rises after neodymium:yttrium-aluminum-garnet (Nd:YAG) laser posterior capsulotomy and can reach levels of 60–80 mmHg. The IOP elevation usually occurs within 2–4 hours of the laser treatment and then abates spontaneously over the next 24 hours. However, in some patients, the pressure elevations can be delayed, can last for days to weeks, or can be severe enough to produce visual loss. Extreme IOP elevations are seen more often in eyes with pre-existing glaucoma and in eyes that do not have posterior chamber IOLs. There is some controversy about whether higher energy levels and larger capsulotomies are more likely to produce pressure rises, but most studies find little correlation. Intraocular pressure measurements should be taken 2–4 hours, 1 day, and 7 days after Nd:YAG laser capsulotomy. Pretreatment with apraclonidine 1% 1 hour prior to surgery and one drop 1 hour after surgery has been shown to decrease the number and severity of postoperative pressure spikes. The duration of the IOP rise may exceed the duration of action of the medication in some cases, and the pressure may be elevated the next day even after being measured as normal an hour or two after surgery. We generally give our patients an additional dose of apraclonidine to take just before bedtime on the night of surgery. This will theoretically help protect against a dangerous pressure rise in the very early morning hours. Patients who still have a substantial rise in IOP should be treated with a topical β-adrenergic antagonist, topical, or, if necessary, a systemic CAI, an α-adrenergic agonist, and hyperosmotic agents as needed to control IOP. Topical corticosteroids may be administered to reduce inflammation. There have been sporadic cases reported of Latanoprost-associated cystoid macular edema in pseudophakic eyes following posterior capsulotomy, but these are rare occurrences and the causal relationship remains obscure.
The pressure rise occurring after Nd:YAG laser capsulotomy is usually associated with particulate debris clogging the trabecular meshwork. Altamirano and co-workers used a flare-cell meter to prospectively measure the level of flare and the amount of particulate matter in the anterior chamber following Nd:YAG laser capsulotomy in 65 eyes of 58 patients. They found that the amount of particulate matter correlated strongly ( P <0.001) with postoperative pressure rises. The amount of flare also correlated with pressure rises, but not as strongly ( P <0.037). Others have found that following Nd:YAG laser capsulotomy in experimental animals, the aqueous humor contains fibrin, lens material, inflammatory cells, macrophages, and RBCs in sufficient quantity to obstruct the trabecular meshwork.
In a few patients, the vitreous moves forward and causes pupillary block. In other cases, Nd:YAG laser surgery causes sufficient bleeding or inflammation to obstruct outflow. More often, however, the outflow facility is reduced in the absence of severe inflammation or hemorrhage. Some have proposed that Nd:YAG laser surgery releases a dialyzable factor from the vitreous that blocks the outflow channels.
GLAUCOMA FROM VITREOUS IN THE ANTERIOR CHAMBER
Vitreous in the anterior chamber is a rare cause of open-angle glaucoma in aphakic eyes. The glaucoma usually begins within weeks after cataract surgery but may be delayed for months. In most cases, the vitreous reaches the anterior chamber after a spontaneous rupture of the hyaloid face or after an extensive posterior vitreous detachment. In a few cases, vitreous is left in the anterior chamber at the time of cataract extraction. When examined, these eyes demonstrate elevated IOP and open angles. Vitreous fills the anterior chamber and appears to be in contact with the trabecular meshwork. It should be emphasized that in many cases, confirming this contact by clinical examination is impossible.
The mechanism of the glaucoma in this entity is not clear because vitreous is present in the anterior chamber of many aphakic eyes without causing problems. It is postulated that glaucoma occurs when a large bolus of vitreous comes into contact with a major portion of the trabecular meshwork and obstructs the outflow system. Grant infused vitreous into enucleated human eyes and found a diminished outflow facility that was reversed by hyaluronidase. In some cases, the mechanism of the glaucoma is more complicated and includes inflammation, pupillary block, and the formation of peripheral anterior synechiae.
Glaucoma caused by vitreous in the anterior chamber is usually a self-limited condition. The vitreous often retracts with time, and then IOP decreases. During this period, topical and systemic pressure-lowering medications are employed to control IOP. The response to miotics is unpredictable in this condition, with some patients helped and others not. Many patients respond better to cycloplegic drugs than to miotics. In unresponsive patients in whom the optic nerve is threatened, vitrectomy should be considered.
GLAUCOMA AFTER TRAUMA
CHEMICAL BURNS
Chemical burns are often associated with a complex pattern of IOP alterations that include an immediate IOP rise followed by a period of hypotony, which in turn is followed by an elevation in the intermediate or late phases of the disease process. Glaucoma is more common after alkali burns but can also be seen after severe acid burns. The diagnosis of glaucoma is often difficult in patients with chemical injuries because opacities of the media may interfere with optic nerve and visual field assessment. Also, external swelling, scarring, and corneal irregularity may interfere with standard methods of tonometry. Intraocular pressure measurements may be more accurate with the pneumatic or MacKay-Marg tonometers than with the Goldmann applanation tonometer.
Intraocular pressure elevations in the early phase of disease are caused by scleral shrinkage and release of active substances, including prostaglandins. This situation is managed by topical and systemic medications, including β-adrenergic antagonists, α-adrenergic agonists, CAIs, and hyperosmotic agents as needed.
Elevated IOP in the intermediate phase is usually caused by inflammation. These eyes are treated with aqueous suppressants, hyperosmotic agents, and cycloplegic drugs. Topical and systemic corticosteroids are also used, but the patients must be monitored closely to avoid corneal melting. At times, sufficient posterior synechiae form to produce pupillary block, which requires vigorous pupillary dilation and/or iridectomy. Acute lens swelling can also produce pupillary block.
Late elevations of IOP are usually caused by trabecular damage and formation of peripheral anterior synechiae or other intraocular scarring. This situation is usually managed by standard medical therapy. Filtering surgery may be required but can be technically difficult if there is extensive scarring of the conjunctiva and episcleral tissues. If extensive scarring is present, a cyclodestructive procedure or a glaucoma drainage device procedure such as an Ahmed or Molteno valve should be considered.
ELECTRIC SHOCK
Transient IOP elevations have been reported after electric injury, cardioversion, and electroshock therapy. Various investigators have attributed the pressure rise to venous dilation, contraction of the extraocular muscles, and pigment dispersion. Because the IOP elevation is usually transient, no therapy is administered.
RADIATION
Radiation can cause elevated IOP through a variety of mechanisms, including neovascularization, open-angle glaucoma associated with diffuse conjunctival telangiectasia, and ghost-cell glaucoma associated with radiation retinopathy and vitreous hemorrhage. In many cases, the widespread ocular disease and radiation damage indicate a poor prognosis.
PENETRATING INJURIES
Penetrating injuries can produce elevated IOP through various mechanisms, including flat anterior chamber with formation of peripheral anterior synechiae; inflammation, including sympathetic ophthalmia; intraocular hemorrhage, including hyphema and ghost-cell glaucoma; lens swelling with pupillary block; lens subluxation with pupillary block; lens-particle glaucoma; phacoanaphylaxis; posterior synechiae with pupillary block; epithelial downgrowth, and fibrous ingrowth. When penetrating trauma includes retained organic material, severe inflammation and secondary glaucoma often follow. When blunt and penetrating trauma are combined, angle recession or other forms of direct trabecular damage may produce elevated IOP.
Retained metallic foreign bodies can produce open-angle glaucoma months to years after the injury. It is postulated that iron released from the foreign body is toxic to several ocular tissues, including the retina and the trabecular meshwork. This condition, known as siderosis , is similar to hemosiderosis, which results from the release of iron from blood. When examined, eyes with siderosis demonstrate heterochromia, mydriasis, elevated IOP, decreased outflow facility, and a diminished electroretinogram. The deep cornea, trabecular meshwork, and anterior subcapsular region of the lens all have a rust-brown color. In some eyes, a previous corneal scar and an iris transillumination defect indicate the path of injury.
Prevention is the key to treating ocular siderosis. Traumatized eyes must be examined carefully with indirect ophthalmoscopy, gonioscopy, ultrasound, radiography, and computed tomography (CT) studies to detect metallic foreign bodies. Whenever possible, the foreign bodies should be removed to prevent this occurrence. Once glaucoma is present, the foreign body may be so encapsulated that standard extraction techniques may not be possible. Furthermore, at this stage, the prognosis may be limited by extensive retinal damage. Standard medical and surgical means are used to treat the glaucoma. Glaucoma and retinal changes are also seen in chalcosis, which is caused by copper-containing foreign bodies.
CONTUSION INJURIES
Contusion injuries occur most frequently in young men and can cause hyphema, iridocyclitis, iris sphincter tears, iridodialysis, cyclodialysis, lens subluxation, retinal tear or dialysis, retinal detachment, vitreous hemorrhage, choroidal rupture, and glaucoma. Postcontusion glaucoma can occur immediately after the injury or be delayed for months to years. Uveal effusion and angle closure can occur rarely after blunt trauma.
The pressure rise that occurs immediately after non-penetrating trauma can be severe but is usually limited in duration to days or weeks. During this period, patients are treated with topical β-adrenergic antagonists, CAIs, and hyperosmotic agents as needed to control IOP. The cause of the early pressure elevation is often complex and includes trauma to the trabecular meshwork as well as obstruction of the outflow channels by RBCs, leukocytes, pigment, and inflammatory debris. Tears in the trabecular meshwork typically occur after contusions but often are not recognized because gonioscopy is not performed routinely ( Fig. 18-13 ). The tears have the appearance of a hinged flap, with the cut edge posterior to Schwalbe’s line. The tear in the meshwork heals within several weeks to months, leaving an area of scarring that is sometimes combined with peripheral anterior synechiae.

In some cases, IOP is low after trauma and then becomes elevated weeks to months later. The likely explanation for this phenomenon is that both outflow facility and aqueous production are reduced immediately after trauma, and then aqueous production recovers first. An alternative explanation is the closing of a tear in the trabecular meshwork or a traumatic cyclodialysis cleft. The IOP elevation that occurs during this period can be very severe and may require filtering surgery if medical treatment fails.
Glaucoma can also occur years or even decades after blunt trauma. Most patients with postcontusion glaucoma have unilateral increased IOP, decreased outflow facility, open angles, and quiet eyes. Many patients have no memory of ocular trauma, whereas others only recall the injury after being questioned by a physician. Other patients will deny a specific history of ocular trauma but fail to consider a routine sports-related injury such as that which occurs during boxing matches or other similar activities. The key finding is a previous tear in the ciliary muscle, called an angle recession ( Fig. 18-14 ). This may be present in the entire circumference of the angle or only in scattered areas. The recession is usually recognized gonioscopically as an irregular widening of the ciliary band. At times, the angle recession is very subtle. In these cases, it is only diagnosed by comparing one eye with the fellow eye or one part of the angle with the remaining parts. Other clues of angle recession include absent or torn iris processes, posterior attachment of the iris root ( Fig. 18-15 ), an anterior chamber deeper than in the fellow eye, and increased visibility and width of the scleral spur. Late glaucoma is more frequent if the recession involves three-quarters or more of the angle but can occur even with recessions of 1 clock hour or less. The angle recession is not the cause of the glaucoma, but rather it is an indicator of previous trauma. Late glaucoma occurs in an estimated 2–10% of eyes after contusion injuries.



It is thought that eyes with an underlying tendency to develop open-angle glaucoma are more likely to develop late increased IOP after blunt trauma. This theory is supported by the observation that many of the supposedly normal, untraumatized fellow eyes have spontaneous IOP elevations. Furthermore, the normal fellow eyes respond to topical corticosteroids, similar to eyes with POAG.
The unifying theory is that eyes with marginal outflow facility are more likely to develop pathologic pressure elevations with trabecular injury than are eyes with excess or reserve outflow facility. Because the outflow facility tends to be about equal in the two eyes, a patient with angle recession and glaucoma might well be expected to have poor outflow in the fellow eye.
Histopathologic study of eyes with post-traumatic glaucoma may reveal a cuticular membrane covering the trabecular meshwork. However, it is not clear whether this membrane is the cause of the glaucoma.
The treatment of late postcontusion glaucoma usually consists of the full antiglaucoma regimen, including miotics. Argon laser trabeculoplasty is often disappointing in this condition but should be considered before proceeding to filtering surgery. It should be emphasized again that the untraumatized fellow eyes should be watched closely for elevated IOP.
GLAUCOMA ASSOCIATED WITH INTRAOCULAR HEMORRHAGE
GHOST-CELL GLAUCOMA
Ghost-cell glaucoma is an uncommon condition that occurs in association with intraocular hemorrhage. In this entity, RBCs degenerate in the vitreous, migrate forward to the anterior chamber through a disrupted anterior hyaloid face, and then obstruct the trabecular meshwork. The vitreous hemorrhage is usually caused by retinal disease, trauma, or surgery; a recent case report associated ghost-cell glaucoma with snake poisoning injury. Generally the anterior hyaloid face has been disrupted by vitrectomy, cataract surgery, or trauma, but ghost-cell glaucoma has been reported in non-traumatized phakic eyes as well. The RBCs in the vitreous degenerate to tan-colored spheres (ghost cells), which appear empty except for clumps of denatured hemoglobin called Heinz bodies. The ghost cells are more rigid than are normal RBCs and thus are less able to pass through the trabecular meshwork. This proposed mechanism is supported by animal experiments in which infusion of fixed RBCs produced elevated IOP.
When examined, patients with ghost-cell glaucoma have elevated IOP, which may be sufficient to cause pain and corneal edema. Slit-lamp examination shows tiny, tan-colored cells in the vitreous, aqueous, and trabecular meshwork. Sometimes the cells in the anterior chamber are so numerous that they settle into a pseudohypopyon. The diagnosis of ghost-cell glaucoma is confirmed by anterior chamber paracentesis. The anterior chamber aspirates can be passed through a Millipore filter and then stained, or the fluid can be examined by phase-contrast microscopy.
Ghost-cell glaucoma is a transient condition that can last for weeks or months depending on the volume of blood in the vitreous and the ability of the trabecular meshwork to clear the degenerated cells. Many cases are controlled by standard medical therapy, including topical β-adrenergic antagonists, α agonists, topical CAIs (oral if necessary), and hyperosmotic agents. Resistant cases are treated with anterior chamber washouts, which can be repeated as needed. If IOP cannot be controlled by repeated anterior chamber lavage, a vitrectomy should be performed to remove as much of the blood as possible.
HEMOLYTIC GLAUCOMA
Hemolytic glaucoma is a rare form of glaucoma associated with intraocular hemorrhage. It resembles ghost-cell glaucoma, except that in hemolytic glaucoma macrophages phagocytize RBC debris and then occlude the trabecular meshwork. When examined, these eyes demonstrate elevated IOP, reddish cells in the aqueous humor, open angles, and increased pigmentation of the trabecular meshwork. The diagnosis is confirmed by anterior chamber paracentesis, which reveals pigment-containing macrophages rather than khaki-colored ghost cells. Microscopic study of eyes with hemolytic glaucoma shows the trabecular meshwork to be occluded by RBCs, debris, and macrophages laden with pigment. Hemolytic glaucoma is usually a self-limited condition that responds to management with topical and systemic pressure-lowering medications. If IOP is not controlled, an anterior chamber washout should be performed. If this is not successful, a filtration or cyclodestructive procedure may be indicated.
HEMOSIDEROSIS
Hemosiderotic glaucoma is a rare entity associated with intraocular hemorrhage. Hemosiderosis is similar to siderosis, except that the source of iron in hemosiderosis is degenerating RBCs rather than a retained foreign body. Hemoglobin released by degenerated RBCs is phagocytized by trabecular endothelial cells. The iron liberated from the hemoglobin causes siderosis and discoloration of the meshwork.
HYPHEMA
Blunt trauma to the globe can produce a tear in the ciliary face and bleeding into the anterior chamber. Traumatic hyphemas can occur in patients of any age or either gender but are typically seen in young men. Patients with hyphemas complain of redness and blurred vision; if IOP is elevated, patients may also complain of pain, nausea, and vomiting. The history of the injury reveals trauma that seems severe in some cases and trivial in others. In all cases, however, the object causing the trauma must have been small enough or sufficiently deformable to fit inside the rim of the orbit in order to strike the globe.
When examined, patients with hyphemas demonstrate diminished vision, conjunctival injection, RBCs floating in the aqueous humor, a variable amount of blood settled to the bottom of the anterior chamber, and normal or low IOPs. Children with traumatic hyphemas often appear somnolent. In most patients, the blood clears spontaneously in a few days with no immediate complications. A few weeks after the injury, these patients should have a careful dilated examination to search for retinal tears, retinal dialyses, and choroidal ruptures. They should also undergo gonioscopy to determine whether angle recession is present.
Unfortunately some patients with traumatic hyphemas have recurrent episodes of hemorrhage into the anterior chamber, or re-bleeds. Most episodes of re-bleeding occur within a few days of the trauma. It is postulated that additional bleeding occurs when the blood clot closing the vessel torn in the original injury undergoes lysis and retraction. Reports indicate that re-bleeding after traumatic hyphema occurs in 4–35% of patients. There is reasonable evidence linking aspirin use to re-bleeding. Some authorities also believe that re-bleeding is more common in blacks and in individuals with larger hyphemas and hypotony.
Re-bleeding is important because it is often associated with complications, including corneal blood staining ( Fig. 18-16 ), optic atrophy, and elevated IOP. In most cases, elevated IOP is caused by RBCs obstructing the trabecular meshwork. Less often, the blood clot may produce pupillary block. Glaucoma is more frequent when the hyphema is total. A total hyphema changing color from red to black ( black-ball or eightball hyphema ) is an ominous sign of impending complications. The exact reason for this is obscure, but it is assumed that the underlying injury is more severe in many eyes with eightball hyphema compared with eyes with subtotal hyphema.

The management of traumatic hyphema has been controversial. Typical practice in the past was to hospitalize all patients for bed rest, sedation, and bilateral patching. However, there is evidence that bed rest and patching are not necessary and that equally good results can be obtained by limiting activity. If there is a stable family situation, some patients can be managed at home and checked daily in the physician’s office. Despite widespread use, no evidence suggests that either cycloplegics or miotics facilitate the clearing of blood. The antifibrinolytic agents epsilon-aminocaproic acid (Amicar) and tranexamic acid have been found to decrease the incidence of rebleeding. The reported track record of tranexamic acid remains fairly good, although its use is not entirely without risk. The effectiveness of aminocaproic acid has been questioned by some investigators, who found no significant difference in re-bleed rates between patients treated with aminocaproic acid and those treated with either corticosteroids or placebo.
This has been a difficult issue to study. The re-bleed rate of the control groups in these studies ranges from less than 5% to over 25%. In several cases, the patients in the study group were collected over periods of 10 years or more, and it is difficult to be certain that identical diagnostic criteria and treatment regimens were employed. For the most part, the studies with high rates of re-bleeding in the control group found the medications helpful. Other studies with seemingly similar entry criteria and treatment plans had low re-bleed rates in the control group and showed no benefit from aminocaproic acid. Some authorities recommend that all patients with traumatic hyphemas be treated with an antifibrinolytic agent, assuming no contraindication. Other authorities use these agents only after severe trauma or in patients with large initial hyphemas. The best available data support using these agents in circumstances in which the re-bleed rate is likely to exceed about 10%. If this is the case in the experience of a particular hospital or practice, it would also seem prudent to carefully review the entire treatment regimen as well as the circumstances and extent of the injury causing the hyphema and the support services available to the patient during convalescence. A few reports indicate that systemic corticosteroids reduce the rate of rebleeding, although this was not confirmed in other studies. Again, patient demographics, the circumstances of injury, and other critical factors are difficult to compare. Elevated IOP associated with a traumatic hyphema is managed by topical and systemic glaucoma medications as needed. Topical corticosteroids are administered if the eye is significantly inflamed, independent of any potential positive effect on re-bleeding rates.
Non-clearing total or subtotal hyphema is a serious condition. In addition to other damage to the eye caused by the event that produced the hyphema, the hyphema itself can cause corneal blood staining, persistent inflammation, and dramatically elevated IOP. Tissue plasminogen activator (t-PA) is a fibrin-specific fibrinolytic agent that has shown promise in experimental and limited clinical use. Current experience remains limited, at least partially because appropriate cases are rare. Early case reports have been favorable, however.
Surgical removal of blood from the anterior chamber is indicated for persistent pain, corneal blood staining, or IOP elevations threatening the optic nerve. It is difficult to know what IOP level will be tolerated by young healthy patients who have no pre-existing optic nerve disease. One proposed guideline is that an IOP of 60 mmHg for 2 days, 50 mmHg for 5 days, or 35 mmHg for 7 days requires intervention. If possible, evacuation of blood should be delayed until the fourth day because, at this time, the clot is somewhat retracted and less adherent to the surrounding tissues. Sometimes, a simple paracentesis allows the clot to retract enough from the chamber angle to allow drainage to occur. Removal of blood also can be done as a washout through a peripheral corneal puncture using saline or a fibrinolytic agent such as urokinase, fibrinolysin, or, when available, t-PA. Liquid blood is irrigated from the eye during this maneuver; it is neither necessary nor wise to remove the entire clot. If the anterior chamber remains shallow and a large clot persists, the surgeon may consider performing an iridectomy to relieve pupillary block.
Some surgeons recommend treating the hyphema through a larger limbal incision using manual expression, ultrasonic emulsification, cryoextraction, or vitrectomy instruments. Other experts recommend a trabeculectomy procedure with or without evacuation.
Patients with sickle cell trait are at great risk of developing IOP elevations and optic nerve damage after traumatic hyphemas. The abnormal RBCs in the anterior chamber develop sickle deformity so they are less able to pass through the trabecular meshwork. Furthermore, increased IOP may reduce the perfusion pressure to the optic nerve and retina and lead to sickling of RBCs within the vessels of these tissues. Accordingly, more aggressive treatment is indicated in patients with sickle cell trait. Unfortunately patients with sickle cell trait may respond adversely to a variety of medications, including hyperosmotic agents, adrenaline (epinephrine), and acetazolamide. However, these individuals generally tolerate topical β-adrenergic antagonists and methazolamide. It has been suggested that mean IOPs greater than 25 mmHg or pressure spikes higher than 30 mmHg indicate the need for surgery in patients with sickle cell trait and traumatic hyphema. One report states that hyperbaric oxygen reduces sickling of RBCs in the anterior chamber of rabbits.
Not all hyphemas arise from contusion injuries. Intraocular bleeding can also occur with penetrating trauma, surgery, neovascularization of the iris or a previous wound, IOLs, tumors, or vascular tufts at the pupillary margin. Elevated IOP is managed with medical therapy and with washout if necessary.
RETINAL DETACHMENT AND GLAUCOMA
Retinal detachment and glaucoma are associated in a variety of ways, including the following:
- 1.
Chance occurrence: because glaucoma and retinal detachment are both common diseases, they occur together by chance.
- 2.
Genetic linkage: in one series, 4% of the eyes with retinal detachment had open-angle glaucoma. This is a much higher prevalence of glaucoma than in the general population. Patients with retinal detachments have larger cup-to-disc diameter ratios and a higher prevalence of corticosteroid responsiveness than would be expected in the general population. Myopia is also associated with glaucoma and retinal detachment. Patients with pigmentary glaucoma also have an increased incidence of retinal detachment.
- 3.
Common underlying mechanism: retinal detachment and glaucoma can be associated through a common underlying mechanism such as trauma, cataract surgery with vitreous loss, proliferative retinopathy, or retinopathy of prematurity.
- 4.
Treatment for retinal detachment may cause glaucoma: therapeutic agents and interventions such as corticosteroids, scleral buckling procedures, extensive retinal photocoagulation, and vitrectomy are all capable of producing glaucoma. In one study, the IOP was measured directly using a canula in patients undergoing scleral buckling surgery. The highest recorded IOP was 211 mmHg! The mean elevation was 112 mmHg for a mean duration of 118 seconds. Glaucoma does not interfere with the reattachment of detached retinas but may limit the visual outcome. Repair of retinal detachment may reduce scleral rigidity, so it is necessary to use applanation rather than Schiøtz tonometry for postoperative pressure measurements.
- 5.
Treatment of glaucoma may cause retinal detachment: strong miotic agents are capable of inducing retinal tears, vitreous hemorrhage, and retinal detachment. There is suggestive evidence that standard cholinergic drugs can also cause retinal detachment. Glaucoma patients should have periodic peripheral retinal examinations, especially before starting miotic therapy. Ophthalmologists should question glaucoma patients about flashes, floaters, and curtains in their vision and should be suspicious of any sudden decrease in IOP.
In most cases of newly diagnosed retinal detachment, IOP is low. However, glaucoma may become apparent later or may be detected in the fellow eye. It is postulated that retinal detachment lowers IOP by inducing inflammation and reducing aqueous humor formation. It is also possible that aqueous humor may be eliminated by flowing through the retinal hole into the subretinal space. Campbell has described an extreme example of this phenomenon, which he calls the iris retraction syndrome . In this syndrome, a patient with a rhegmatogenous retinal detachment, secluded pupil, iris bombé, and angle-closure glaucoma develops hypotony and iris retraction when aqueous formation is reduced pharmacologically. Campbell postulates that the induced reduction in aqueous formation allows most of the aqueous humor to go posteriorly through the retinal hole.
SCHWARTZ SYNDROME
In a minority of cases, retinal detachment causes a peculiar increase in IOP that is referred to as Schwartz syndrome . In this syndrome, rhegmatogenous retinal detachment is associated with elevated IOP, diminished outflow facility, open angles, and cell and flare in the aqueous humor. When the retinal detachment is repaired, theIOP and outflow facility return to normal. It has been postulated that the glaucoma is related to angle recession, inflammation, pigment granules released by the retinal pigment epithelium, and glycosaminoglycans synthesized by the photoreceptors. One additional suggestion is that photoreceptor outer segments migrate through the retinal hole and obstruct the trabecular meshwork. The IOP can vary from mildly elevated to very high, and medical treatment is rarely successful in controlling the condition. Schwartz syndrome must be distinguished from glaucoma and non-rhegmatogenous retinal detachment caused by an undetected malignant melanoma.
GLAUCOMA AFTER VITRECTOMY
Elevated IOP has been reported in 20–26% of eyes after vitrectomy. This occurs through a variety of mechanisms ( Box 18‑2 ). Because most of these entities are discussed in detail elsewhere in this book, only a few general remarks are offered here.
Pre-existing glaucoma
Angle recession
Ghost cell
Primary open-angle glaucoma
Pigmentary glaucoma
Associated with intraocular hemorrhage
Hyphema
Ghost cell
Hemolytic
Hemosiderosis
Related to lens material
Phacolytic
Lens particle
Phacoanaphylactic
Neovascular
Inflammatory
Corticosteroid induced
Intraocular gas or liquid
Air
Viscoelastic substances
Perfluorocarbons
Silicone
Modified from Wilensky JT, Goldberg MF, Alward P: Glaucoma after pars plana vitrectomy, Trans Am Acad Ophthalmol Otolaryngol 83:114, 1977.
It is important to monitor IOP postoperatively by applanation tonometry because the accuracy of indentation tonometers is affected by scleral surgery. In addition, intraocular gas may interfere with pressure measurements using a Schiøtz tonometer.
Liquid silicone is sometimes injected into the vitreous cavity in cases of complicated retinal detachment. The silicone and macrophages laden with silicone may obstruct the trabecular meshwork. The silicone may also cause pupillary block, which can be prevented by an inferior iridectomy at the time of surgery. The iridectomy has a somewhat greater chance of closing in these cases; t-PA helped keep the peripheral iridectomy open in a case reported by MacCumber and co-workers. In many cases of intravitreal silicone injection, IOP is depressed by persistent inflammation, hyposecretion, retinal detachment, or cyclitic membrane formation.
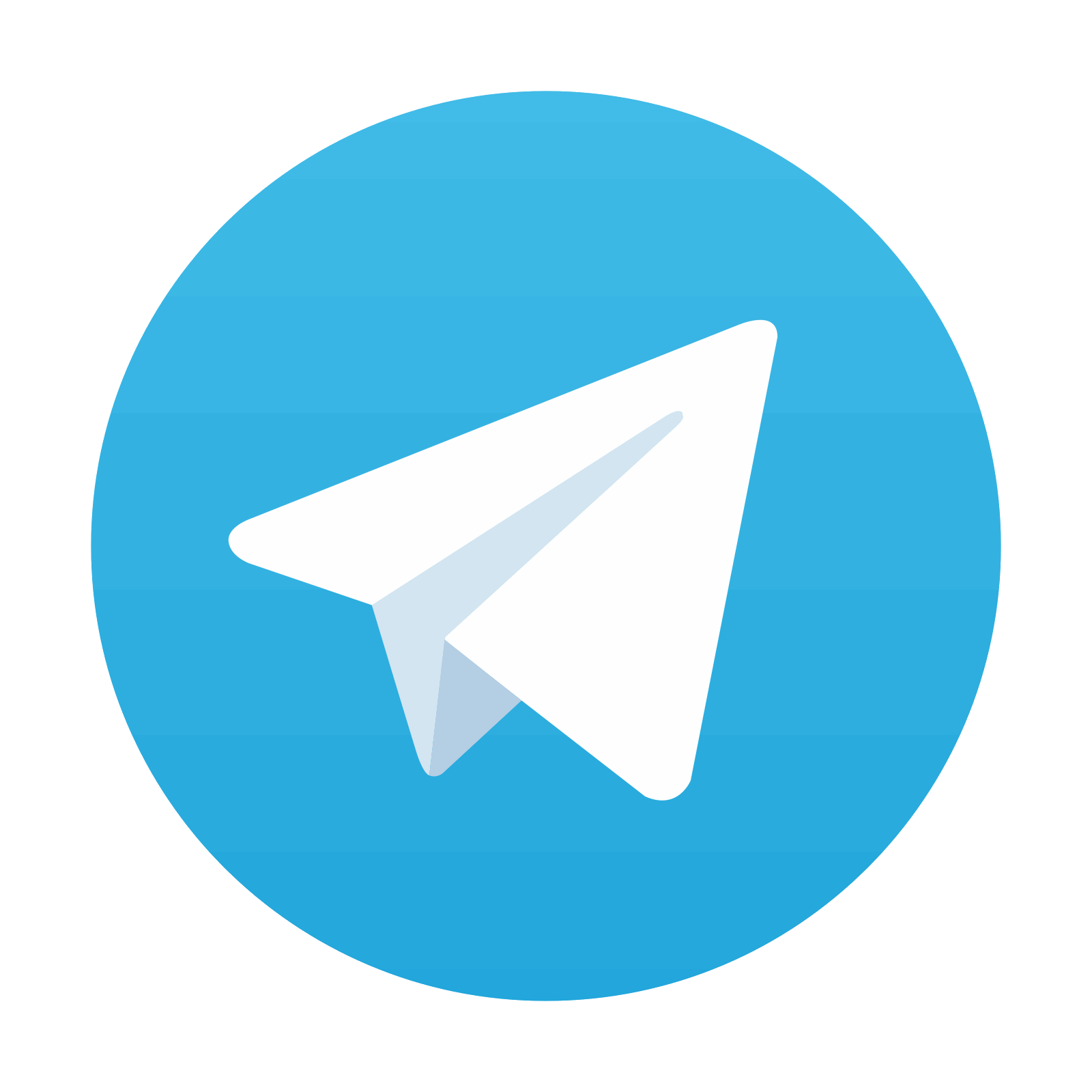
Stay updated, free articles. Join our Telegram channel

Full access? Get Clinical Tree
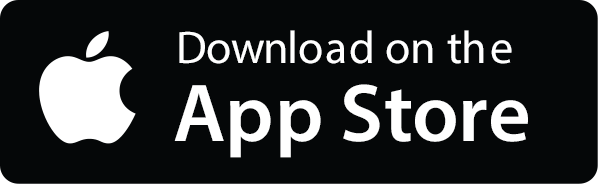
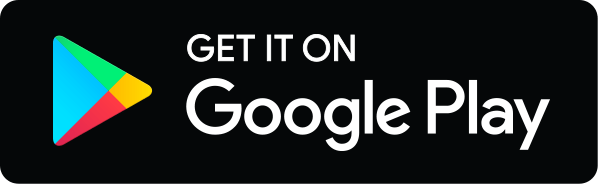