Key points
- •
High-resolution CT of the temporal bone offers excellent visualization of the osseous anatomy of the temporal bone, but has some limitations in the evaluation of soft tissue.
- •
MRI is associated with a higher cost and probable need for sedation, but offers excellent soft tissue detail and superior identification of intracranial pathology compared to CT.
- •
For children being considered for cochlear implantation, MRI is the recommended imaging study of choice.
- •
Enlarged vestibular aqueduct is the most common imaging finding. Findings are bilateral in up to 87% of patients and associated with cochlear malformation in 84%.
Introduction
Hearing loss is a common problem within the pediatric population, with 6 in 1000 children being diagnosed by the age of 18. Over the past several decades, the universal screening of infants has been significantly expanded to evaluate 95% of newborns for hearing loss. Through the implementation of early screening, the age of diagnosis has been reduced from the previous norm of 2 to 3 years of age, to the current level of 2 to 3 months. As early detection has increased, focus has shifted to the diagnostic workup and the role of genetic, laboratory, and imaging studies. With this review, we seek to provide a guide for the use of imaging in pediatric patients with sensorineural, conductive, or mixed hearing losses. As such, we address the choice of imaging, findings, and clinical implications based on type of hearing loss at presentation. It is our hope that this format will present the most current information in a concise and more useful manner than cataloging imaging findings by Computed tomography (CT) or MRI.
Choice of Imaging
For a majority of the 20th century, temporal bone tomography was the only imaging modality available to clinicians. Using this technique, studies reported temporal bone abnormalities in up to 18% of typical deaf patients. Despite the obvious limitations, plain films were used to identify congenital aplasia of the cochlea, glomus tumors, and temporal bone fractures. Despite some utility, the use of plain films has been supplanted by more advanced (higher resolution) imaging.
The development of high-resolution CT of the temporal bone has allowed clinicians to dramatically augment the clinical history and physical examination and to arrive at more accurate and precise diagnoses. CT offers excellent visualization of the osseous anatomy of the temporal bone, but has limitations in the evaluation of soft tissues. These limitations are best exemplified by the lower sensitivity/specificity for the detection of acoustic neuromas compared with MRI, the inability to distinguish between soft tissue inflammation versus cholesteatoma consistently, and the inability to detect specific soft tissue lesions (eg, facial neuromas). In practical terms, CT offers the advantage of short image acquisition times and the lack of need for contrast in the vast majority of clinical indications. These characteristics are important in the pediatric population, with the short study duration reducing the need for sedation and the associated potential complications.
MRI of the internal auditory canal (IAC) and temporal bone offers excellent soft tissue detail not afforded by CT. In the 1980s, contrast-enhanced MRI became the standard imaging modality for the detection of lesions of the IAC. More recently, noncontrast fast spin-echo T2-weighted MRI and CISS (constructive interference) imaging protocols have been shown to possess adequate sensitivity and specificity to allow for IAC screening purposes. As such, children with sensorineural hearing loss (SNHL), without suspicion of neoplastic, infectious or inflammatory processes, do not necessarily require intravenous contrast. Published data suggest that MRI in the setting of pediatric SNHL workup should be extended to capture images that include the entire brain. Up to 20% of patients with SNHL have detectable intracranial findings, including gliosis, cortical dysplasia, brainstem hypoplasia, and cerebellar tumors. As a balancing factor, the time required for MRI represents a relative disadvantage compared with CT. With MR studies requiring approximately 20 minutes to complete, sedation is required frequently in younger patients.
Although the imaging modality used should be dictated primarily by clinical needs, it is important to acknowledge that other factors may influence decision making. Although the cost of MRI has decreased over recent years, it remains significantly more expensive than CT. For example, at our tertiary pediatric institution, a typical CT of the temporal bones (without contrast) incurs a charge of $1952. MRI is associated with a charge of $3178 without contrast and $3577 with contrast. In an increasingly cost-conscience health care environment, it has been suggested that CT may be a better initial choice in certain patient populations. However, patient safety is another highly relevant (if not more important) factor to consider. Recently, there has been a concerted effort to reduce the radiation exposure of children secondary to imaging studies. In a landmark study, Pearce and colleagues followed 178,604 patients who underwent CT scans over a 24-year period in Great Britain. Use of CT in children delivering a dose of 50 mGy tripled the risk of leukemia, whereas CT in children with a dose of 60 mGy tripled the risk of brain cancer. These exposures resulted in 1 additional case of leukemia and 1 additional case of brain cancer per 10,000 CT scans. It should be noted that because these neoplasms are rare entities, the absolute risk remains low. Nevertheless, it must be remembered that the clinical information obtained via temporal bone CT comes with a very small but real risk of inducing neoplasm. As a result of this and other research, The Alliance for Radiation Safety in Pediatric Imaging has introduced the Image Gently campaign to reduce the radiation exposure in pediatric patients ( http://www.imagegently.org ). Clinicians should consider global and long-term health risks before ordering imaging and should make every attempt to reduce the exposure of their patients to radiation.
Introduction
Hearing loss is a common problem within the pediatric population, with 6 in 1000 children being diagnosed by the age of 18. Over the past several decades, the universal screening of infants has been significantly expanded to evaluate 95% of newborns for hearing loss. Through the implementation of early screening, the age of diagnosis has been reduced from the previous norm of 2 to 3 years of age, to the current level of 2 to 3 months. As early detection has increased, focus has shifted to the diagnostic workup and the role of genetic, laboratory, and imaging studies. With this review, we seek to provide a guide for the use of imaging in pediatric patients with sensorineural, conductive, or mixed hearing losses. As such, we address the choice of imaging, findings, and clinical implications based on type of hearing loss at presentation. It is our hope that this format will present the most current information in a concise and more useful manner than cataloging imaging findings by Computed tomography (CT) or MRI.
Choice of Imaging
For a majority of the 20th century, temporal bone tomography was the only imaging modality available to clinicians. Using this technique, studies reported temporal bone abnormalities in up to 18% of typical deaf patients. Despite the obvious limitations, plain films were used to identify congenital aplasia of the cochlea, glomus tumors, and temporal bone fractures. Despite some utility, the use of plain films has been supplanted by more advanced (higher resolution) imaging.
The development of high-resolution CT of the temporal bone has allowed clinicians to dramatically augment the clinical history and physical examination and to arrive at more accurate and precise diagnoses. CT offers excellent visualization of the osseous anatomy of the temporal bone, but has limitations in the evaluation of soft tissues. These limitations are best exemplified by the lower sensitivity/specificity for the detection of acoustic neuromas compared with MRI, the inability to distinguish between soft tissue inflammation versus cholesteatoma consistently, and the inability to detect specific soft tissue lesions (eg, facial neuromas). In practical terms, CT offers the advantage of short image acquisition times and the lack of need for contrast in the vast majority of clinical indications. These characteristics are important in the pediatric population, with the short study duration reducing the need for sedation and the associated potential complications.
MRI of the internal auditory canal (IAC) and temporal bone offers excellent soft tissue detail not afforded by CT. In the 1980s, contrast-enhanced MRI became the standard imaging modality for the detection of lesions of the IAC. More recently, noncontrast fast spin-echo T2-weighted MRI and CISS (constructive interference) imaging protocols have been shown to possess adequate sensitivity and specificity to allow for IAC screening purposes. As such, children with sensorineural hearing loss (SNHL), without suspicion of neoplastic, infectious or inflammatory processes, do not necessarily require intravenous contrast. Published data suggest that MRI in the setting of pediatric SNHL workup should be extended to capture images that include the entire brain. Up to 20% of patients with SNHL have detectable intracranial findings, including gliosis, cortical dysplasia, brainstem hypoplasia, and cerebellar tumors. As a balancing factor, the time required for MRI represents a relative disadvantage compared with CT. With MR studies requiring approximately 20 minutes to complete, sedation is required frequently in younger patients.
Although the imaging modality used should be dictated primarily by clinical needs, it is important to acknowledge that other factors may influence decision making. Although the cost of MRI has decreased over recent years, it remains significantly more expensive than CT. For example, at our tertiary pediatric institution, a typical CT of the temporal bones (without contrast) incurs a charge of $1952. MRI is associated with a charge of $3178 without contrast and $3577 with contrast. In an increasingly cost-conscience health care environment, it has been suggested that CT may be a better initial choice in certain patient populations. However, patient safety is another highly relevant (if not more important) factor to consider. Recently, there has been a concerted effort to reduce the radiation exposure of children secondary to imaging studies. In a landmark study, Pearce and colleagues followed 178,604 patients who underwent CT scans over a 24-year period in Great Britain. Use of CT in children delivering a dose of 50 mGy tripled the risk of leukemia, whereas CT in children with a dose of 60 mGy tripled the risk of brain cancer. These exposures resulted in 1 additional case of leukemia and 1 additional case of brain cancer per 10,000 CT scans. It should be noted that because these neoplasms are rare entities, the absolute risk remains low. Nevertheless, it must be remembered that the clinical information obtained via temporal bone CT comes with a very small but real risk of inducing neoplasm. As a result of this and other research, The Alliance for Radiation Safety in Pediatric Imaging has introduced the Image Gently campaign to reduce the radiation exposure in pediatric patients ( http://www.imagegently.org ). Clinicians should consider global and long-term health risks before ordering imaging and should make every attempt to reduce the exposure of their patients to radiation.
Sensorineural hearing loss
SNHL will be diagnosed in 1 to 2 newborns per 1000 births in the United States each year. The presentation of SNHL in children is variable ranging from profound congenital bilateral deafness to mild adolescent unilateral hearing loss. As such, the diagnostic workup and results will be influenced by a myriad of factors including onset, severity, patient characteristics, genetic or syndromic factors, and laterality.
Imaging Protocols
Bilateral sensorineural hearing loss
Before embarking on a discussion of the workup of bilateral SNHL in children, it is necessary to take a slight tangent and mention the impact of molecular genetic testing and the recognition of the significance of genetic hearing loss as it pertains to contemporary imaging diagnostic algorithms. First described in 1997, mutations of the Gap Junction Beta 2 (GJB2) gene result in defects of the connexin 26 protein. This autosomal-recessive form of hearing loss has been implicated as the cause of hearing loss in up to 40% of children with severe to profound congenital SNHL. It is has been argued that the diagnostic workup in patients with confirmed autosomal-recessive hearing loss can, and should, be limited to only truly necessary studies. To support this proposal, Preciado and associates evaluated the relevance of GJB2 screening on diagnostic workup. Overall, 18% of patients with SNHL were found to be positive for biallelic GJB2 mutations. This detection rate increased to 37% in the subset of patients with severe to profound hearing loss. In patients found to be GJB2 positive, only 1 patient was noted to have an enlarged vestibular aqueduct (EVA), representing a rate of less than 4% in this specific population. Given the low diagnostic yield of imaging in GJB2-positive patients, the authors recommended an updated diagnostic algorithm. This algorithm seeks to avoid unnecessary cost and risk to patients by integrating genetic screening with imaging, in an algorithm that reaches the final diagnoses with the least number of tests and the lowest incurred costs. As depicted in Fig. 1 , patients with bilateral SNHL and screening negative for deafness-causing mutations would proceed to diagnostic imaging (eg, MRI). The diagnostic yield of imaging studies in these patients is significantly influenced by the severity of hearing loss. In 1 study of patients with bilateral SNHL undergoing MRI, an increase in yield was noted from mild to moderate (21.2%) to moderate to severe (24.7%) and to severe to profound (29.9%). Other authors have demonstrated an even more dramatic difference in diagnostic yield between mild to moderate loss (29%) and severe to profound hearing loss (48%). Accordingly, these data may be useful in identifying patients most likely to have relevant imaging findings in the setting of limited resources.
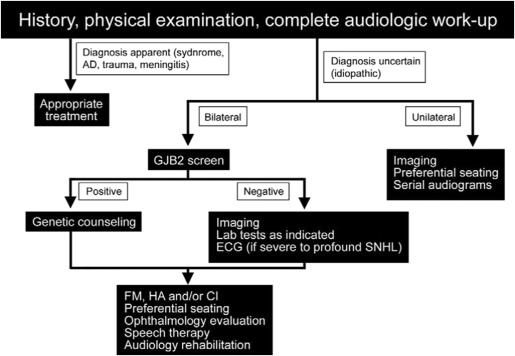
Unilateral sensorineural hearing loss
For patients with unilateral SNHL, temporal bone imaging offers the highest diagnostic yield rate of all available testing. Approximately 35% of studies (MRI or CT imaging ) will provide a definitive diagnosis as to the etiology of a child’s unilateral SNHL. Interestingly, it is not uncommon for patients with unilateral SNHL to have bilateral findings on imaging. Using CT, Song and colleagues demonstrated bilateral findings in 5.6% of patients, with EVA and Mondini malformation representing the most common abnormalities in the otherwise normal hearing ear. This finding is supported by previous audiometric studies showing that 11% of patients with unilateral SNHL will eventually demonstrate bilateral disease. As a result, imaging in unilateral hearing loss is essential to provide diagnoses as well as to evaluate the contralateral ear, and perhaps, provide some prognostic information regarding the child’s hearing.
MRI versus computed tomography
The choice of the optimal imaging modality remains a controversial topic. CT is the imaging modality of choice in patients with a conductive hearing loss component, suspected superior semicircular canal dehiscence, or temporal bone trauma. Likewise, MRI is selected when retrocochlear and intracranial pathology must be ruled out. Both MRI and CT can be effective in identifying common inner ear malformations, such as EVA ( Fig. 2 ). Unfortunately, few studies have directly compared the two modalities in the same patients. In fact, concurrent CT and MRI have only been evaluated in patients with unilateral SNHL. Concordance between CT and MRI in this setting has been reported as high as 76%. The weaknesses of each modality have been proposed, such as MRI failing to detect EVA, and CT occasionally missing cochlear nerve hypoplasia. Unfortunately, these studies remain limited because fewer than 50% of patients underwent both MRI and CT. This selection bias prevents any meaningful conclusions about the superiority of either modality at this time.
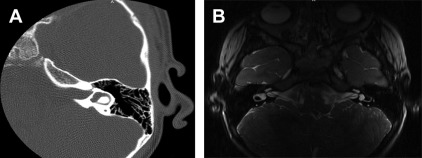
Proposed algorithm
At our institution, patients with SNHL undergo an imaging workup using a structured algorithm (see Fig. 1 ) based on diagnostic yield. Patients presenting with bilateral moderate, severe, or profound congenital SNHL first undergo genetic screening. As discussed, this screening effectively reduces the need for further imaging and laboratory studies, and offers a potential cost savings. Patients with negative genetic testing undergo a noncontrast fast spin-echo T2-weighted MRI to evaluate inner ear and central nervous system structures. In cases of unilateral SNHL, patients proceed directly to MRI regardless of other clinical factors. Despite the need for sedation, MRI has become our imaging modality of choice owing to its superior visualization of the cochlear nerve and central nervous system, its excellent resolution of the inner ear structures, the avoidance of exposure to ionizing radiation, and the ability to perform the study early in the child’s life when cochlear implantation is being considered.
For the child with unexplained conductive hearing loss, temporal bone CT imaging is the preferred modality. This imaging, however, can wait until the child is 5 to 6 years old because surgery will not be considered until at least that age, and the larger child receives less total body radiation.
Common Findings
The scope of findings on inner ear imaging in SNHL is exceptionally broad. This review emphasizes the most common findings and their clinical implications.
Enlarged vestibular aqueduct
EVA represents the most common inner ear malformation detected in patients with SNHL. This finding can be bilateral in up to 87% of patients. Although EVA is a congenital condition, hearing loss may not be evident (or even present) immediately from birth, with onset of hearing loss often occurring postlingually. Variability of vestibular aqueduct enlargement and dysmorphology are common in imaging studies ( Fig. 3 ). Diagnosis of EVA was initially defined by Valvassori and Clemens as a midpoint vestibular aqueduct greater than 1.5 mm in width. More recently, Vijayasekaran and colleagues have sought to define normative data for vestibular aqueduct size. Using the 95th percentile as a cutoff point, they defined EVAs as having a midpoint and opercular width of 1.0 and 2.0 mm, respectively. The use of these more inclusive criteria is supported by the audiology findings of Madden and colleagues. Their study of EVA patients revealed that borderline EVA (midpoint of 1.0–1.5 mm and operculum of 1.5–2.0) was associated with SNHL that is prone to progression and fluctuation.
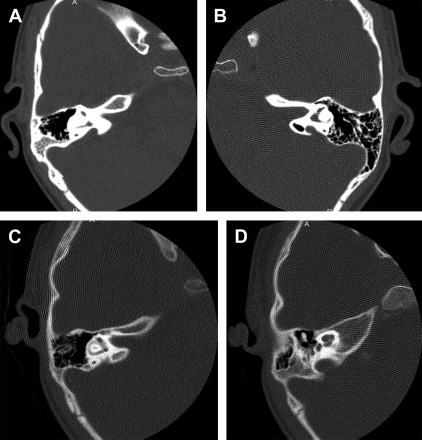
EVA is rarely found in isolation, with 84% of patients having an associated cochlear or vestibular anomaly ( Fig. 4 ). Associated cochlear anomalies can range from mild asymmetry of the modiolus to classic Mondini malformation. Although progression of hearing loss in EVA is variable, profound hearing loss is both possible and common. In particular, head trauma has been linked to progression of hearing loss in up to 4% of patients with EVA. As a result, children are urged to avoid situations where head trauma is a significant possibility or likelihood. The radiographic diagnosis of EVA at an early age allows physicians to offer prognostic information to patients and families, and to counsel those patients at risk for potentially preventable progression of hearing loss.
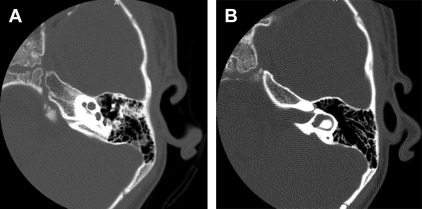
Congenital cochlear abnormalities
Congenital cochlear malformations represent a common finding on both CT and MRI evaluations. Isolated abnormalities of the cochlea have been detected in 4.1% of patients with SNHL. Mondini malformation, or type II incomplete partition, accounts for 55% of all cochlear deformities ( Fig. 5 ). This malformation was first reported by Carlo Mondini in 1791 and was originally described as having an absent interscalar septum and osseous spiral lamina. Imaging classically reveals an absent modiolus and a cochlea limited to 1.5 turns. Mondini malformations are frequently accompanied by other deformities, with 20% of patients having an additional abnormality of the vestibular aqueduct, vestibule, endolymphatic sac/duct, or semicircular canals.
Although less common, other cochlear abnormalities may also be discovered in patients with SNHL on imaging. Complete labyrinthine aplasia (Michel aplasia) represents the most severe and rare malformation of the inner ear. Imaging in these cases reveals a complete absence of inner ear structures that may be unilateral or bilateral. Other findings associated with cochlear anomalies include petrous bone aplasia, narrowed or aplastic IAC, tegmen defects, and middle ear/mastoid hypoplasia. Common cavity deformity occurs with significant frequency, representing 25% of malformations. This deformity is characterized by limited differentiation of the cochlea and vestibule, resulting in a single cavity ( Fig. 6 ). Common cavity deformity and complete labyrinthine aplasia can be distinguished by the presence of the IAC in patients with common cavity malformations.
Development of the cochlea can be arrested or perturbed at any point, resulting in deformities ranging from complete aplasia to mild hypoplasia. Cochlear aplasia is characterized by a complete absence of the cochlea in the setting of a normal, dilated, or hypoplastic vestibular system. In cochleovestibular malformation, also known as type 1 incomplete partition, the modiolus is absent with imaging studies revealing a cystic cochlea and dilated vestibule. In 15% of malformations, the cochlea will be hypoplastic, with imaging demonstrating a small cochlear bud of variable length with only 1 or a partial turn.
Recent studies have suggested that, with expanded use of MRI, cochlear abnormalities may be detected with greater frequency than even EVA. In patients with SNHL, McClay and colleagues observed mild cochlear dysplasia in 23% of patients. In this study, the mild dysplasia ranged from isolated deficiency of the modiolus to absence of the cochlear apical turn. The incidence of mild dysplasia in this study is notably greater than both previously reported CT numbers and the accepted frequency of EVA.
Cochleovestibular nerve deficiency
Cochlear nerve deficiency (CND) is defined as an absent or hypoplastic cochlear nerve and is diagnosed in 12% to 18% of patients with SNHL. Using CT, the IAC and cochlear nerve canal are defined as narrow when less than 4 mm and less than 1.8 mm in size, respectively. However, when compared with MRI these criteria have respective sensitivities of only 76% and 68% for the detection of CND. Using IAC size alone is therefore insufficient, because patients with normal caliber IAC may still have detectable CND on MRI. Suspicion for CND should be high in patients with profound congenital SNHL because the cochlear nerve is more likely to be hypoplastic compared to normal controls. In addition, patients may have bilateral nerve deficiency or hypoplasia as well as other associated inner ear abnormalities. Given the propensity for CND, evaluation of the cochlear nerve with MRI is appropriate in the workup of patients with SNHL and may offer useful information, particularly before cochlear implantation ( Fig. 7 ).
