Protection of the Upper Airway
The Nose
As air is inspired through the nose, it immediately encounters the first of many protective features of the sinonasal cavity: the vibrissae. The vibrissae are coarse hairs whose follicles are located just within the nasal meatus. They filter large aerosolized particulate matter from inspired air, and they are aided in expelling the debris by the sweat and sebaceous glands in the stratified squamous epithelium of the anterior nares, the only squamous epithelium of the sinonasal cavity. Vibrissae are present in most mammals, but their innervation and role in sensory function appears to be less pronounced in humans.
Just beyond the meatus of the anterior nares and vibrissae, inspired airflow is regulated by the nasal valves, the next tier of sinonasal defense. The external nasal valve is defined by the angle between the lateral crus and the medial crus of the lower lateral cartilage, the columella, and the nasal sill. The internal nasal valve—the narrowest segment of the upper airway—is defined by the angle between the caudal upper lateral cartilage, the septum, the anterior face of the inferior turbinate, and the nasal floor. Both valves are dynamic such that they limit airflow at variable rates. When the velocity of inspired air increases with forceful inspiration, the pressure of the air passing through the nasal valves decreases relative to the ambient air outside the nasal valve in accordance with the Bernoulli principle of fluid dynamics. This pressure differential causes the nasal valve to decrease in aperture, varying with respect to the individual size, shape, and soft tissue compliance of a particular nose. During times of labored breathing or exercise, the alar muscles contract to dilate the nares to oppose the effect of this air pressure differential. This mechanism allows the nasal valves to ensure that air is not inspired faster than it can be warmed, humidified, and cleaned.
The Turbinates
Inspired air then encounters the turbinates of the nasal cavity. The three shelflike structures project from the lateral wall of the nasal cavity toward the septum and provide a crucial protective function of the sinonasal cavity. The superior and middle turbinates are extensions of the ethmoid bone, whereas the inferior turbinate is an independent osseous structure. Most air passes between the middle and inferior turbinates, with a maximum velocity just posterior to the internal nasal valve.1 By increasing the total surface area of the nasal cavity, the turbinates significantly contribute to warming and humidifying inspired air. At respiratory rates of up to 7 L per minute, the nasal airway can warm air to 37°C from ambient temperatures as low as 25°C and maintain the humidity of inspired air at ~85% within a wide range of ambient environmental humidity.2 This controlled temperature and humidity of air entering the lungs facilitates pulmonary alveolar gas exchange.
The orientation and shape of the turbinates streamlines inspired air posteriorly toward the nasopharynx while providing sufficient obstructive resistance to change the airflow from a laminar to a transitional pattern. Additionally, the cross-sectional area of the nasal airway increases substantially just past the nasal valves, leading to a drop in airflow velocity just before the air reaches the turbinates; this too contributes to the loss of laminar airflow.2 Transitional airflow is neither completely laminar nor completely turbulent, and its dynamics are protective in several ways. The element of turbulence causes much of the aerosolized debris, including pathogens and dust, to precipitate from inspired air and land in the mucus layer of the epithelium. The change in airflow pattern also exposes more air molecules to the warm and moist mucosa of the large turbinates, whereas a laminar flow would shield a central column of air from the mucosa. However, a completely turbulent flow with no laminar component would be undesirable because it would generate higher resistance and lower velocity of the airflow in the nasal passage. Such turbulence becomes useful only during the sniff, the mechanism of olfactory detection whereby quick high-flow (>300 mL/s per nostril) inhalation generates enough turbulence to expose more odorants to the olfactory mucosa, tucked posterosuperior to the superior turbinate.3 Therefore, the transitional airflow pattern of normal breathing balances the protective features of turbulent flow with the lower resistance of laminar flow.
When nasal resistance is too low, however, patients may paradoxically report subjective complaints of obstruction or difficulty breathing, known as “empty nose syndrome.” The turbinates may provide up to 50% of total airway resistance when one breathes nasally. Thus, after excessive surgical turbinectomy, the altered velocity differentials and resistance patterns throughout the upper airway may result in a paradoxical nasal obstruction, with patients reporting shortness of breath and unsatisfying breathing.4 These clinical sequelae demonstrate the functional importance of turbinates to effectively create a unique transitional airflow pattern that is necessary to protect the upper airway while allowing for normal and comfortable breathing.
Nasal air filtration proves to be remarkably efficient. Humans inspire between 10,000 and 20,000 L of air per day. About 80% of particles 3 to 5 µm in diameter and 60% of particles 2 µm in diameter are filtered by nasal filtration, with the vast majority of this filtration process occurring anteriorly. Studies with radiolabeled water have found that ~95% of pollen-sized particles are trapped in the anterior nasal cavity.5 However, despite this powerful filtration system, countless contaminants including particulate debris and infectious pathogens pass through to the upper airway and become trapped in the mucus layer of the sinonasal epithelium, generally allowing only particles less than 1 µm to pass into the lungs. Mucociliary clearance is the process by which the mucus layer containing such debris is transported to the gastrointestinal tract for elimination, and it is the definitive mode of defense for both the upper and lower airways.
The Nasal Cycle
In the anatomically normal nasal airway, most people experience asymmetric airflow through the nose. At any time, one nasal passage is typically more widely patent to airflow and has increased secretions from both serous and mucus glands, whereas the other passage is more congested with reduced secretions.2 This feature of the nasal airway, known as the “nasal cycle,” is tightly regulated by a combination of neural control and vasomotor input that results in alternating engorgement and constriction of the arterioles, precapillary sphincters, and most significantly, the venous sinusoids within the erectile mucosa of the nasal passage. The mechanisms governing these vascular changes are not completely understood, but vascular tone is influenced by factors including local metabolic and vasoactive substances, neurotransmitters, and neuropeptides ( Table 2.1 ).
This erectile tissue is most fully developed along the anterior nasal septum and on the inferior turbinates, but is distributed throughout much of the nasal airway. At peak congestion, the mucosal edge of the inferior turbinate may protrude anteriorly as far as 5 mm beyond its baseline de-congested position.6 Sympathetic adrenergic input leads to constriction of the vasculature and a patent nasal passage, which plays an additional role in increasing systemic oxygenation in the fight-or-flight response. Parasympathetic input may contribute to vasodilatation and engorgement of the distensible mucosa but appears to play a much smaller role in this process. Rather, parasympathetic input to the sinonasal cavity functions largely to stimulate glandular secretions.7
Vasodilators | Vasoconstrictors |
Histamine | Epinephrine |
Vasoactive intestinal peptide | Norepinephrine |
Substance P | |
Prostaglandin E2 | |
Bradykinin | |
Nitric oxide | |
Estrogen |
The nasal cycle has been described as far back as 1895, when Kayser found that patients had varying airway resistance in each nasal passage, yet a constant total resistance of the whole nasal airway.8 Some investigators have found using rhinomanometry that ~80% of normal people undergo this recurring process, and that the patent and congested sides alternate every 2 to 7 hours on average.7 However, the term “nasal cycle” has been called into question, as there is little data to confirm that a consistent rhythmic periodicity exists that predicts the alternating patency and congestion of a nasal passage. Rather, it is now commonly accepted that most people exhibit an irregularly alternating patency and congestion governed by many factors including posture, infection, mucosal irritants, hormones, temperature, and drugs. Kayser was still correct in his original estimation that, despite changing resistance of individual nasal passages, the total resistance of the combined nasal cavities remains largely unchanged,8 and because there is often no sensation of obstruction from the congested side, the cycle goes unnoticed by most people.
The functions of the nasal cycle are not entirely understood, but it most likely serves both mechanical and immunologic roles. The cycle′s mechanical functions include augmenting the efficacy of the turbinates to humidify and warm inspired air. During inspiration of cold or dry air, the blood is directed through the capillaries and venous sinusoids leading to engorgement of the erectile tissue and swelling of the nasal lining. Additionally, the nasal cycle may play a role in controlling nasal secretions, whereby the alternating congestion and decongestion creates a pump-like mechanism for plasma transudate, an important component of these secretions. During the period of decongestion, the increased sympathetic tone that constricts the venous sinusoids of the erectile tissue may raise their intravascular hydrostatic pressure, resulting in increased plasma filtration through the endothelium of the distensible venous sinusoids in the nasal lining.9 This plasma transudate, as a component of nasal secretions, has been suggested to play a major role in the first line of defense against pathogenic organisms that enter the nose as the primary site of infection. Indeed, studies have confirmed that the amplitude of the nasal cycle as measured in unilateral nasal resistance is increased during acute viral upper respiratory infections,8 supporting the immunological role of the nasal cycle.
The system of neural and humoral factors that control the nasal mucosa is complex and includes adrenergic and cholinergic fibers as well as vasoactive intestinal peptide and nitric oxide, where parasympathetic input causes engorgement and congestion and sympathetic input causes constriction and patency ( Table 2.1 ). Most nasal decongestants are selective alpha agonists that cause precapillary vasoconstriction with selective shunting of blood through the arteriovenous anastomoses, leading to decreased nasal edema.
The Sneeze Reflex
The sneeze reflex is a protective mechanism that causes a forceful nasal expulsion of air and secretions in response to an irritating or noxious stimulus. It protects the upper airway in a manner analogous to the cough reflex of the lower airway. The function of this reflex is to expel the offending irritant from the nasal cavity. The velocity of exhaled air associated with a sneeze may reach in excess of 100 miles per hour.10 Thus, the sneeze is a highly effective neuromuscular response involving both cranial and spinal nerves. Although the exact neural arcs are unknown, certain neurological deficits have been described that lead to an impaired sneeze reflex.
While the olfactory nerve conveys the specialty olfactory senses (and is discussed in detail in Chapter 4), the trigeminal nerve conveys thermal, noxious, and mechanical stimuli from the nasal cavity. When an irritant is present in the nose, the sneeze reflex is initiated through activation of the afferent fibers of the trigeminal nerve (V1 and V2). The efferent parasympathetic fibers, carried from the pterygopalatine ganglion, then stimulate the corresponding mucosa to increase local secretions, the initial effort to flush or dislodge the irritant. Simultaneously, the phrenic nerve stimulates the diaphragm to activate inspiration. Then, the anterior abdominal wall muscles are stimulated to contract, generating a powerful exhalation. Concurrently, the glossopharyngeal and vagus nerves elevate the palate and contract the superior pharyngeal constrictor, causing a brief Valsalva maneuver, before forcing the pressurized exhalation through the nasal cavity.
In cats, a “sneeze center” has been localized to the lateral medulla, the brainstem region containing the glossopharyngeal and vagus nerve nuclei.11 It has been suggested that an analogous region exists in humans. Wallenberg syndrome is a relatively common manifestation of ischemic insult to the lateral medulla caused by vertebral or posterior inferior cerebellar artery occlusion. Indeed, there have been several case reports of patients with Wallenberg syndrome who have an impaired sneeze reflex.12 Such patients describe the sensation of the urge to sneeze, but no spontaneous forced nasal expulsion ensues. This complaint corresponds to their neurological deficit of impaired motor function from the glossopharyngeal and vagus nerve nuclei despite intact sensory function of the trigeminal nerve and nucleus, providing evidence that humans may have a localized brainstem center for the sneeze reflex as well.
The Mucosa, Mucus, Cilia, and Mucociliary Clearance
The Mucosa
When aerosolized pathogens and debris pass through the initial tiers of nasal defense and are greater than 0.5 to 1 µm, they become trapped in the mucus layer of the sinonasal mucosa. The sinonasal mucosa protects the cavities and upper airway from inhaled pathogens, toxins, and debris by mucociliary clearance, a specialized function of the unique protective epithelium of the airway. Mucociliary clearance, which comprises mucus production and mucus transport, removes both healthy secretions and pathological debris from the sinonasal airway. Although the cough and sneeze mechanisms may supplement mucus clearance from the lung and nose respectively, mucociliary clearance remains the principal mode of defense of the respiratory system, especially of the paranasal sinuses.
The mucosa consists of a superficial layer of epithelium with variable numbers of goblet cells, an acellular basement membrane, a thick lamina propria containing vascular and glandular layers, and the periosteum ( Fig. 2.1 ). The epithelium of each part of the nasal cavity is specialized to suit its particular region. The anterior margin of the nasal vestibule is composed of stratified squamous epithelium. Containing sebaceous glands, sweat glands, vibrissae, and finer hair, the stratified squamous epithelium provides a protective barrier much like the skin of the rest of the face. Around the area of the nasal valves, the stratified squamous epithelium transitions to the pseudostratified columnar ciliated epithelium found throughout the rest of the nasal cavity (with the exception of the olfactory epithelium). This region of epithelial transition, which is 1 to 2 mm in thickness, overlies dense arterial anastomoses. The anastomoses lead to a plexus of capillaries derived from the anterior ethmoid, greater palatine, sphenopalatine, and superior labial arteries. This plexus, known as Kiesselbach′s or Little′s area, is believed to be the source of 80% of epistaxis, owing to its rich blood supply and anterior location.13
The transitional area between stratified squamous epithelium and pseudostratified columnar ciliated epithelium enlarges as a person ages. As the most anteroinferior columnar epithelium is exposed to years of desiccation from inspired airflow, it may undergo squamous metaplasia, a common adaptive mechanism of stressed epithelium. The total surface area of intranasal squamous epithelium thus often increases over the course of one′s life.

The respiratory mucosa of the remainder of the sinonasal cavity and turbinates consists of columnar epithelium (~80%), goblet cells (~20%), and basal cells (less than 5%). Although the nasal columnar epithelium is predominantly pseudostratified, including both ciliated and nonciliated cells, the paranasal sinus columnar epithelium is predominantly simple ciliated columnar cells.1 Both ciliated and nonciliated columnar cells have hundreds of immotile microvilli along their surface, tiny hairlike projections of actin filaments 1 to 2 µ m in length covered by the cell membrane. The microvilli tremendously increase the total surface area of the columnar cells, which likely aids the sinonasal mucosa in mucus production, secretion, and sensation.14
The goblet cells, interspersed throughout the columnar cells, contribute to mucus production via secretory granules that contain mucin—a glycoprotein essential to the viscosity and elasticity of mucus. The apical surface of a goblet cell is also covered with microvilli and has a small duct through which it releases its secretions into the nasal cavity ( Fig. 2.2 ). Basal cells, attached to the basement membrane by hemidesmosomes, are shielded from the abrasive and contaminated environment of the nasal cavity and paranasal sinuses. In addition to serving as a progenitor cell that can differentiate into goblet or ciliated columnar cells, basal cells may also aid in anchoring the overlying columnar cells to the basement membrane.15
The epithelium of the sinonasal mucosa is constructed with three types of intercellular junctions. Adherens junctions, comprised of actinlike filaments, bind epithelial cells to their basement membrane with protein structures including hemidesmosomes, macula adherents, and zonula adherents ( Fig. 2.3 ). Tight junctions surround each epithelial cell and ensure that the epithelial layer remains impermeable to water molecules, ions, and potential pathogens. Finally, gap junctions provide small windows connecting the cytoplasm of adjacent epithelial cells. They allow certain ions and charges to pass through and likely play an important role in ciliary coordination.16


Beneath the thin basement membrane lies the lamina propria, the layer containing the glands, vasculature, and nerves supplying the sinonasal mucosa. The lamina propria has a superficial glandular layer, a vascular layer, and a deep glandular layer. Anterior serous glands, present on the anterior septum and lateral nasal wall, produce a watery secretion that contributes to the moisture of the nasal cavity. Seromucinous glands are found throughout the cavity and contribute a combination of serous and mucinous secretions. Intraepithelial glands consist of several goblet cells arranged around a lumen and contribute a small amount of mucus to nasal secretions. The parasympathetic fibers that course throughout the lamina propria originate in the superior salivatory nucleus of the brainstem and are carried by the nervus intermedius branch of the facial nerve to the greater superficial petrosal nerve. The sympathetic fibers of the nasal mucosa originate in the sympathetic trunk, synapse in the superior cervical ganglion, and are carried by the deep petrosal nerve. The greater superficial petrosal and deep petrosal nerves then join to form the vidian nerve, which carries the autonomic nervous supply through the pterygopalatine ganglion, where parasympathetic fibers synapse, and the autonomic fibers are then carried to the mucosa by the trigeminal nerve (V2). The parasympathetic fibers of the lamina propria directly stimulate glandular secretions and can be blocked by atropine or other anticholinergics. The sympathetic fibers of the lamina propria, as mentioned, appear to play a more significant role in mucosal vasoconstriction and decongestion than in regulating nasal secretions.15
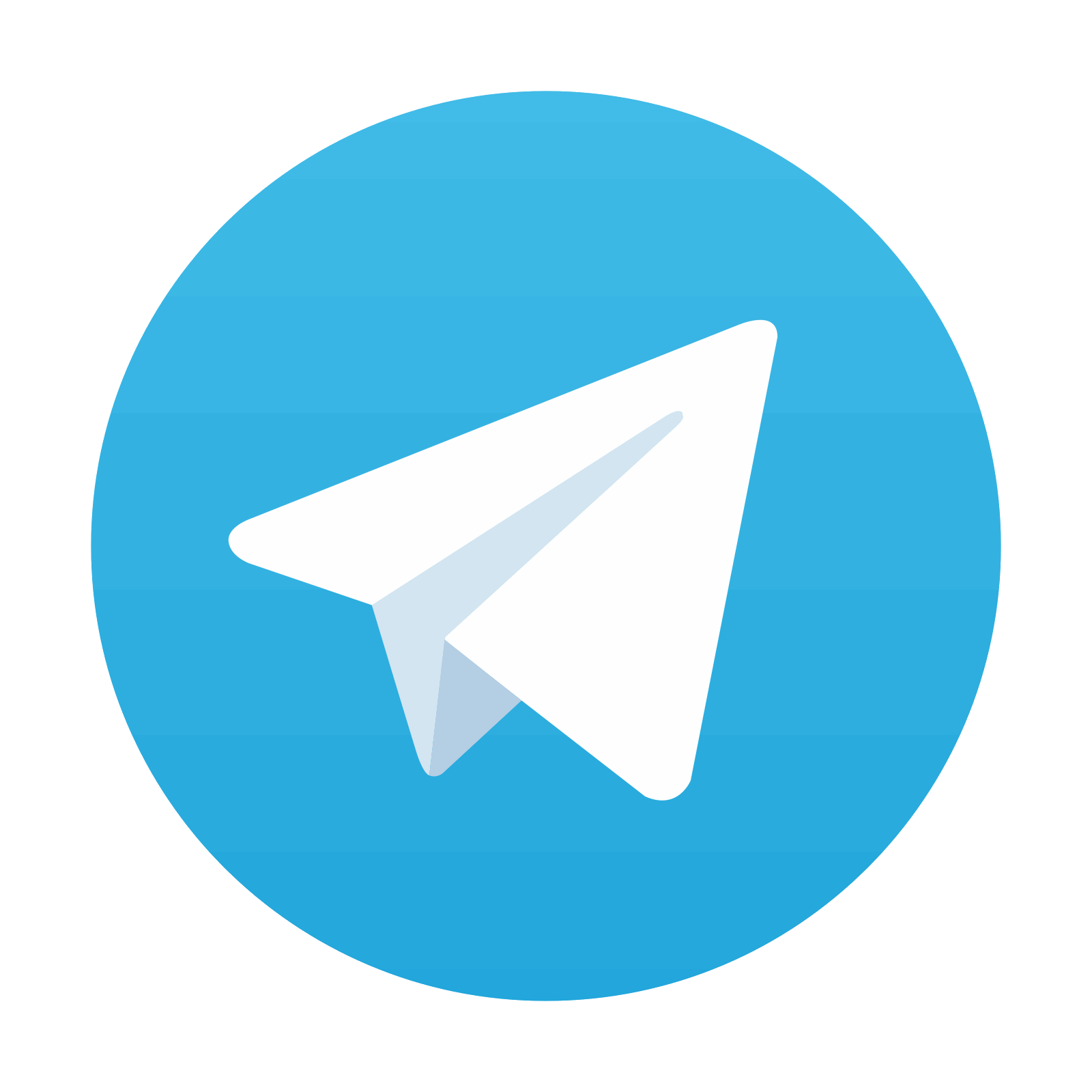
Stay updated, free articles. Join our Telegram channel

Full access? Get Clinical Tree
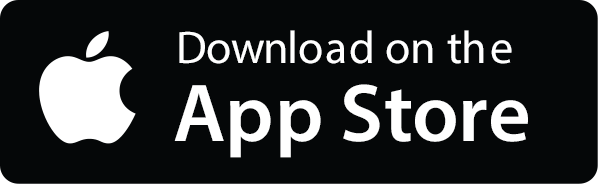
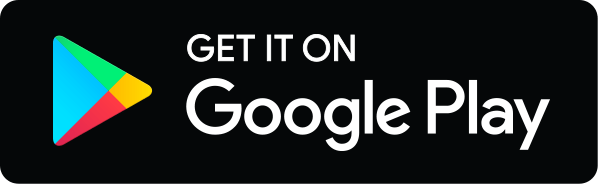
