In the healthy eye, flow of aqueous humor against resistance generates an intraocular pressure (IOP) of approximately 15 mmHg, necessary for the proper shape and optical properties of the globe. The circulating aqueous nourishes the cornea and lens, structures which must be transparent and therefore devoid of blood vessels as well as the trabecular meshwork (TM). The aqueous also provides a transparent and colorless medium of refractive index 1.33332 between the cornea and lens, thus constituting an important component of the eye’s optical system. The basic anatomy of the primate anterior ocular segment and the normal pathways of aqueous humor flow are illustrated schematically in Figures 11.1 and 11.2 .


Aqueous humor is secreted by the ciliary epithelium lining the ciliary processes (as a consequence of active ionic transport across the ciliary epithelium and hydrostatic and osmotic gradients between the posterior chamber and the ciliary process vasculature and stroma), and enters the posterior chamber. It then flows around the lens and through the pupil into the anterior chamber. There is convection flow of aqueous in the anterior chamber – downward close to the cornea where the temperature is cooler, upward near the lens where the temperature is warmer (seen clinically when there is particulate matter in the anterior chamber – e.g. cells). Aqueous leaves the eye by passive bulk flow mainly via two pathways at the anterior chamber angle:
- (1)
through the TM, across the inner wall of Schlemm’s canal into its lumen, and thence into collector channels, aqueous veins, and the episcleral venous circulation – the trabecular or conventional route;
- (2)
across the iris root, uveal meshwork, and the anterior face of the ciliary muscle, through the connective tissue between the muscle bundles, the suprachoroidal space, and thence out through the sclera – the uveoscleral, posterior, or unconventional route.
Whether or not there is a net osmotic resorption of some of the aqueous passing through the uvea by the uveal venous circulation has not been verified. , In young individuals of various monkey species, total aqueous drainage is relatively evenly divided between the two pathways. In the human eye, the uveoscleral pathway has more recently been shown to play a more prominent role in aqueous outflow than was originally suggested by earlier studies. , By using indirect methods, such as fluorophotometry, uveoscleral outflow accounts for approximately 35–55 percent of total aqueous drainage in younger humans. , In normal healthy subjects 60 years of age or older, uveoscleral outflow is significantly reduced compared to that of 20–30-year-olds (mean ± SD = 1.10 ± 0.81 vs 1.52 ± 0.81 µl/min; p<0.009). Similarly, in rhesus monkeys >25 years of age (human equivalent >62 years of age) uveoscleral outflow is also dramatically reduced (0.33 ± 0.08 vs 0.63 ± 0.07 µl/min, mean ± SEM). There is no significant net fluid movement across the cornea, iris vasculature, or vitreoretinal interface, although ion fluxes exist. ,
Due to the autonomic innervation and receptors of the relevant structures, adrenergic and cholinergic mechanisms play major roles in aqueous humor formation and drainage in terms of both normal physiology and glaucoma therapeutics, while evidence for other mechanisms, including serotonergic, dopaminergic, adenosinergic, nitrergic, cannabinergic, prostaglandin(PG)ergic, , and cytoskeletal (see review ), has been established.
Aqueous humor formation
Physiology of aqueous humor formation
Until the early twentieth century, aqueous humor was regarded as a stagnant fluid. Since that time, however, it has been shown to be continuously formed and drained and the associated anatomic drainage portals (Schlemm’s canal, collector channels, aqueous veins, ciliary muscle interstices) have been described. ,
Three physiologic processes contribute to the formation and chemical composition of the aqueous humor: diffusion, ultrafiltration (and the related dialysis), and active secretion. Diffusion and ultrafiltration are responsible for the formation of the “reservoir” of the plasma ultrafiltrate in the stroma, from which the posterior chamber aqueous is derived, via active secretion across the ciliary epithelium. Energy-dependent active transport of sodium into the posterior chamber by the non-pigmented ciliary epithelium ( Fig. 11.3 ) results in water movement from the stromal pool into the posterior chamber. It seems fairly certain that under normal conditions active secretion accounts for perhaps 80–90 percent of total aqueous humor formation. The observation that moderate alterations in systemic blood pressure and ciliary process blood flow have little effect on aqueous formation rate supports this notion. ,

Furthermore, Bill noted that the hydrostatic and oncotic forces that exist across the ciliary epithelium–posterior aqueous interface favor resorption, not ultrafiltration, of aqueous humor. Active secretion is essentially pressure-insensitive at near-physiologic IOP. However, the ultrafiltration component of aqueous humor formation is sensitive to changes in IOP, decreasing with increasing IOP. This phenomenon is quantifiable and is termed facility of inflow, or pseudofacility, the latter because a pressure-induced decrease in inflow will appear as an increase in outflow when techniques such as tonography and constant-pressure perfusion are used to measure outflow facility. Although measurements vary, pseudofacility in the non-inflamed monkey and human eye constitute a very small percentage of total facility. Recently it has been argued that fluid transport rates exhibited by the ciliary epithelia are insufficient to account for the rate of aqueous humor formation and that there may be some contribution from fluid directly entering the anterior chamber across the anterior surface of the iris. ,
In most mammalian species, the turnover constant of the anterior chamber aqueous humor is ~0.01 to 0.015 × min −1 , that is, the rate of aqueous humor formation and drainage is ~1.0 to 1.5 percent of the anterior chamber volume per minute. This is true also in the normal human eye, in which the aqueous formation rate is ~2.5 µl × min −1 . ,
More comprehensive theoretic analyses of the fluid mechanics of aqueous production can be found elsewhere.
Biochemistry of aqueous humor formation
The structural basis for aqueous humor secretion is the bilayered ciliary epithelium. This consists of the pigmented epithelium (PE) facing the ciliary stroma and the non-pigmented epithelium (NPE) facing the aqueous humor. The PE and NPE are connected to each other at their apical membranes through gap junctions, thereby forming a complex syncytium. Tight junctions are present between the apical borders of NPE cells where they form a barrier that restricts paracellular diffusion.
The active process of aqueous secretion is mediated via selective transport of certain ions and substances across the basolateral membrane of the non-pigmented ciliary epithelium against a concentration gradient. Two enzymes abundantly present in the non-pigmented ciliary epithelium are intimately involved in this process: sodium-potassium-activated adenosine triphosphatase (Na + -K + -ATPase) and carbonic anhydrase. Na + -K + -ATPase is found predominantly bound to the plasma membrane of the basolateral infoldings of the non-pigmented ciliary epithelium.
As a result of the primary active transport of Na + , other ions and molecules are transported over the epithelium by secondary active transport. Thus, aqueous humor in humans exhibits increased levels of ascorbate, some amino acids, and certain ions such as CI − as compared to plasma. There is also a passive transporter for HCO 3 − . , A summary of the biochemistry of aqueous secretion is shown in Figure 11.3 .
Inhibition of the ciliary process Na + -K + -ATPase by cardiac glycosides (e.g. ouabain) or vanadate (VO 3 − , VO 4 3− ) significantly reduces the rate of aqueous humor formation and IOP in experimental animals and humans, showing that the primary active transport of Na + is the primary driving force for the secretion of aqueous humor. To maintain electroneutrality, anions must accompany the actively secreted Na + , chloride can pass through chloride channels in the basolateral membrane and HCO 3 − can enter aqueous via exchange with chloride. The active transport of Na + and the accompanying anions create high osmolarity on the basolateral side of the NPE cells, which causes diffusion of water out of the cells. The movement of water is facilitated by aquaporins in NPE cells (aquaporins 1 and 4). AQP1 null mice have decreased IOP and aqueous humor production compared to normals. Sodium and chloride must continuously enter the pigmented epithelial cells for the continuous secretion of aqueous humor. This is achieved by Na + /H + and Cl − /HCO 3 − antiports and by the Na-K-2Cl cotransporter. In vivo as well as in vitro studies support the involvement of these transporters and channels in the formation of aqueous humor.
Transport of Cl − ions plays an equally important role as the active transport of Na + ions as the driving force for aqueous humor formation. In isolated bovine eyes, aqueous humor is formed mostly by processes involving active secretion and chloride transport. In rabbit preparations, the release of Cl − ions is enhanced by agonists of A3 adenosine receptors. A3 adenosine receptor-activated agonists and antagonists, respectively, increase and lower IOP in mice. Bestrophin-2, a protein which regulates Ca 2+ -activated Cl − conductance, is localized to the non-pigmented ciliary epithelium, suggesting that it may play a role in regulating aqueous humor formation. Mice deficient in bestrophin-2 have significantly diminished IOP.
In bovine eyes in vitro, 4,4′-diisothiocyanatostilbene-2,2′-disulfonic acid (DIDS, a probable inhibitor of the Cl − /HCO 3 − exchanger, the Na-HCO 3 cotransporter, and chloride channel) and 5-nitro-2-(3-phenylpropylamino)-benzoic acid (NPPB, a chloride channel blocker in non-pigmented cells) reduce aqueous humor formation by 55 percent and 25 percent respectively. In mice, topical inhibitors of Na + /H + antiports lower IOP. Bumetanide (a specific inhibitor of Na-K-2Cl cotransport) and furosemide (a nonspecific anion transport inhibitor) reduce aqueous humor formation by 35 and 45 percent in bovine eyes in vitro. Also, catecholamines such as epinephrine, norepinephrine, isoproterenol and dopamine, that stimulate aqueous humor formation in humans also stimulate Na-K-Cl cotransport. However, topical application of bumetanide to mice or monkeys has no effect on IOP.
Carbonic anhydrase (CA) is abundantly present in the basal and lateral membranes and cytoplasm of the pigmented epithelium and non-pigmented epithelium of the ciliary processes. Isoenzymes of CA (II, IV and XII) are present in the ciliary processes. ,
The conversion of CO 2 and H 2 O to carbonic acid and its subsequent dissociation to H + and HCO 3 − provides the HCO 3 − , which is essential for the active secretion of aqueous humor. Inhibition of the production of HCO 3 − also leads to an inhibition of the active transport of Na + across the non-pigmented ciliary epithelium into the first-formed aqueous, thereby reducing active aqueous humor formation. There are several hypotheses to explain the relationship between reduction in non-pigmented ciliary epithelium intracellular HCO 3 − and inhibition of Na + transport including:
- (i)
inhibition of CA causing a decrease in HCO 3 − available for transport with Na + from the cytosol of the non-pigmented ciliary epithelium to the aqueous, required to maintain electroneutrality;
- (ii)
reduction in intracellular pH inhibiting Na + -K + -ATPase, and
- (iii)
decreased availability of H + decreasing H + /Na + exchange and reducing the availability of intracellular Na + for transport into the intercellular channel.
In addition, inhibition of renal and erythrocyte CA leads to a systemic acidosis which promotes inhibition of aqueous humor formation.
CA inhibitors given systemically can reduce secretion by up to 50 percent and have been in use for clinical glaucoma therapy for over 50 years. It was once thought that the drug concentration at the ciliary epithelium required to produce nearly continuous, total ciliary CA inhibition necessary to achieve adequate and sustained reduction in aqueous humor formation (over 99 percent of the ciliary enzyme must be inhibited to achieve significant secretory suppression) might never be attainable via the eyedrop route. Fortunately, there are now available topically effective CA inhibitors such as dorzolamide and brinzolamide which may achieve substantial (albeit usually not quite as much) reduction in IOP as the earlier oral CA inhibitors but without their systemic side-effects.
Aqueous humor composition
The composition of aqueous humor differs from that of plasma as a result of two important physiological characteristics of the anterior segment: a mechanical epithelial/endothelial blood–aqueous barrier, and active transport of various organic and inorganic substances by the ciliary epithelium. The greatest differences are the low protein and high ascorbate concentrations in the aqueous relative to plasma (200 times less and 20 times greater, respectively).
The protein concentration in the peripheral portion of the anterior chamber, close to the meshwork, may be much higher than in the more central region because of protein entry directly from the peripheral iris, as demonstrated in monkey and human eyes. When the aqueous protein concentration rises much above its normal 20 mg/100 mL, as in uveitis, the resultant light scattering (Tyndall effect) makes the slit-lamp beam visible as it traverses the anterior chamber (a phenomenon known as “flare”).
The high ascorbate concentration may help protect the anterior ocular structures from ultraviolet light-induced oxidative damage. Ascorbate functions as an antioxidant, regulates the sol–gel balance of mucopolysaccharides in the TM, or partially absorbs ultraviolet radiation, as diurnal mammals have approximately 35 times the concentration of aqueous ascorbate compared to nocturnal mammals. Extensive and repeated oxidative stress in vivo may result in reduced TM cell adhesion, leading to TM cell loss which is associated with glaucomatous conditions. ,
Lactate is also normally in excess in the aqueous, presumably as a result of glycolytic activity of the lens, cornea, and other ocular structures. , Other compounds or ions in excess in the aqueous relative to plasma are Cl − and certain amino acids. Glucose, urea, and non-protein nitrogen concentrations are slightly less than in plasma. , Oxygen is also present in the aqueous humor, at a tension determined to lie between 13 to 80 mmHg, depending upon the method of measurement. ,
Blood–aqueous barrier
The blood–aqueous barrier is a functional concept, rather than a discrete structure, invoked to explain the degree to which various solutes are relatively restricted in travel from the ocular vasculature into the aqueous humor. The capillaries of the ciliary processes and choroid are fenestrated, but the interdigitating surfaces of the retinal pigment epithelia and the ciliary process non-pigmented epithelia respectively are joined to each other by tight junctions (zonulae occludens) and constitute an effective barrier to intermediate- and high-molecular-weight substances, such as proteins. The endothelia of the inner wall of Schlemm’s canal are similarly joined, , preventing retrograde movement of solutes and fluid from the canal lumen into the TM and anterior chamber. Tight junctions are also present in the iridial vascular endothelium as well as between the iris epithelia. For present purposes, one may say that the blood–aqueous barrier comprises the tight junctions of the ciliary process non-pigmented epithelium, the inner wall endothelium of Schlemm’s canal, and the iris vasculature, and the outward-directed active transport systems of the ciliary processes (see below). A more universal concept of the blood–aqueous barrier must deal with the movement of smaller molecules, lipid-soluble substances, and water into the eye.
With disease-, drug-, or trauma-induced breakdown of the blood–aqueous barrier ( Table 11.1 ), plasma components enter the aqueous humor. Net fluid movement from blood to aqueous increases, but so does its IOP dependence (pseudofacility). Total facility, as measured by IOP-altering techniques cannot distinguish pseudofacility from total outflow facility (Ctot) and therefore erroneously record the pseudofacility component as increased Ctot (hence the term, “pseudofacility”) and therefore underestimate the extent to which the outflow pathways have been compromised by the insult. Under these circumstances, increased pseudofacility provides some protection against a precipitous rise in IOP; as IOP rises, aqueous inflow by ultrafiltration is partly suppressed, blunting (but not completely suppressing) , further IOP elevation. Additionally, the inflammatory process which occurs during blood–aqueous barrier breakdown leads to a reduction in active secretion of aqueous humor, possibly via interference with active transport mechanisms. , This in turn may actually produce ocular hypotony, despite compromised conventional outflow pathways (due to plasma protein blockage of the TM). Prostaglandin release during inflammation may contribute to the hypotony by increasing aqueous outflow via the uveoscleral route. , When the noxious stimulus is removed, however, the ciliary body may recover before the TM, and the resulting normalization of the aqueous humor formation and uveoscleral outflow rates in the face of still compromised conventional outflow pathways leads to elevated IOP, as seen from the modified Goldmann equation:
IOP = [ ( AHF − F u ) / C trab ] + P e
I. Traumatic | II. Pathophysiologic | III. Pharmacologic |
---|---|---|
A. Mechanical | A. Vasodilation | A. Melanoycte-stimulating hormone |
1. Paracentesis | 1. Histamine | B. Nitrogen mustard |
2. Corneal abrasion | 2. Sympathectomy | C. Cholinergic drugs, especially cholinesterase inhibitors |
3. Blunt trauma | B. Corneal and intraocular infections | D. Plasma hyperosmolality |
4. Intraocular surgery | C. Intraocular inflammation | |
5. Stroking of the iris | D. Prostaglandins (varies with type, dose and species) | |
B. Physical | E. Anterior segment ischemia | |
1. Radiotherapy | ||
2. Nuclear radiation | ||
C. Chemical | ||
1. Alkali | ||
2. Irritants (e.g. nitrogen mustard) |
Active transport
The ciliary processes possess the ability to actively transport a variety of organic and inorganic compounds and ions out of, or to exclude them from, the eye, that is, to move them from the aqueous or vitreous to the blood against a concentration gradient. Para-aminohippurate (PAH), diodrast, and penicillin are examples of large anions that are actively transported out of the eye. These systems are similar to those in the renal tubules and satisfy all the criteria for active transport: saturability, energy and temperature dependence, Michaelis Menten kinetics, inhibition by ouabain and probenecid, etc. In addition, another system can actively excrete injected iodide from the aqueous, resembling iodide transport in the thyroid and salivary glands. The physiologic role of these outward-directed systems is unknown. With the discovery that prostaglandins may be actively transported out of the eye, , some workers have suggested that such outward-directed mechanisms may rid the eye of biologically active substances which are no longer needed or may even be detrimental. The various efflux transporters in ocular tissues are discussed in more detail in Chapter 17 .
Pharmacology and regulation of aqueous humor formation and composition ( Box 11.1 )
IOP displays a circadian rhythm in animals and humans which is under the control of endogenous pacemaker structures including the suprachiasmic nucleus, which control the activity of sympathetic and parasympathetic ocular innervation. Sympathetic and parasympathetic nerve terminals are present in the ciliary body arising from branches of the long and short posterior ciliary nerves. These nerve fibers are of both the myelinated and non-myelinated variety. Parasympathetic fibers originate in the Edinger–Westphal nucleus of the third cranial nerve, run with the inferior division of this nerve in the orbit, and synapse in the ciliary ganglion. Vasodilatory parasympathetic nerve fibers originating in the pterygopalatine ganglion are likely to release nitric oxide and vasoactive intestinal peptide (VIP) in addition to acetylcholine (see Chapter 10 ). Nerves displaying VIP immunoreactivity are also detected in the ciliary processes, posterior third of the ciliary muscle and around small to medium-sized blood vessels in the posterior uvea of the cat. Only a few VIP-positive fibers are found in the ciliary processes of humans and monkeys. In the cat eye, pituitary adenylate cyclase-activating peptide (PACAP)-containing nerve fibers are detected in the iris, ciliary body and conjunctiva. PACAP immunoreactivity co-localizes with VIP in the sphenopalatine ganglion and with calcitonin gene-related peptide (CGRP) in the trigeminal ganglion. Sympathetic fibers synapse in the superior cervical ganglion and distribute to the muscles and blood vessels of the ciliary body. Stimulation of the cervical sympathetic nerves in vervet monkeys significantly increases the rate of aqueous humor formation. Numerous unmyelinated nerve fibers surround the stromal vessels of the ciliary processes; these are most likely noradrenergic and subserve vasomotion.
- •
β-adrenergic antagonists such as timolol, that lower IOP by decreasing aqueous humor formation, continue to be a mainstay in clinical glaucoma therapy
- •
Nocturnal IOP elevation in glaucomatous eyes may not be effectively eliminated with adrenergic-based glaucoma therapies
- •
α2-adrenergic agonists such as apraclonidine and brimonidine, are powerful ocular hypotensive agents, lowering IOP primarily by decreasing aqueous humor formation
- •
Long-term suppression of aqueous humor formation and redirection of aqueous drainage from the trabecular to the uveoscleral pathway could lead to underperfusion of the trabecular meshwork with detrimental morphologic and functional effects
- •
Brinzolamide or timolol added to latanoprost have similar ocular hypotensive effects and safety in POAG, NTG, or OHT. The small difference in efficacy between the secretory suppressants is buried beneath the bigger IOP effect of latanoprost. This has to do with the physics of IOP; at a lower pressure, it is harder to get the same magnitude of effect
Sympathetic denervation in monkeys does not alter resting aqueous humor formation and marginally affects the aqueous humor formation response to timolol or epinephrine. In humans with unilateral Horner’s syndrome, IOP, daytime aqueous humor formation, tonographic outflow facility and the flow and IOP response to timolol are similar in both eyes. However, eyes with Horner’s syndrome show a decrease in aqueous humor formation in response to epinephrine instead of the normal increase. Isoproterenol, on the other hand, increases flow during sleep in both normal and Horner’s syndrome eyes but has no significant effect on flow in either during the day. Chemical sympathectomy with guanethidine sulfate in glaucomatous humans lowers IOP, presumably by reducing aqueous humor production measured indirectly by tonography. VIP stimulated aqueous formation following intravenous administration in monkeys is secondary to activation of the sympathetic nervous system while the effect of intracameral administration of VIP is a direct effect on the ciliary epithelium. PACAP appear to be slightly more potent than VIP as a stimulator of aqueous humor flow in the monkey. The role of sympathetic innervation in mediating aqueous inflow and outflow responses to pharmacologic agents remains largely uncertain. Also the role of sympathetic innervation in mediating inflow responses can vary depending on the species used, i.e. rabbits vs monkeys, as is found, for example, with opioid agonists. ,
Cholinergic mechanisms
The effects of cholinergic drugs on aqueous humor formation and composition and on the blood–aqueous barrier are unclear. Overall, cholinomimetics have little effect on the volumetric rate of aqueous humor formation. In general, cholinergic drugs cause vasodilation in the anterior segment resulting in increased blood flow to the choroid, iris, ciliary processes, and ciliary muscle (see Chapter 10 ). However cholinergic drugs may also promote vasoconstriction in the rabbit eye. Congestion in the iris and ciliary body is a well-recognized clinical side-effect of topical cholinomimetics, especially the anticholinesterases. The presence of flare and cells in the aqueous humor by biomicroscopy indicates that these agents can cause breakdown of the blood–aqueous barrier and perhaps frank inflammation. Pilocarpine increases blood–aqueous barrier permeability to iodide and inulin. Cholinergic drugs may alter the aqueous humor concentration of inorganic ions and the movement of certain amino acids from the blood into the aqueous humor and may also influence the outward-directed transport systems of the ciliary processes. , Cholinergic agents can disrupt the coupling and Na + currents between pigmented and non-pigmented epithelial cells in vitro, suggesting a possible inhibitory effect on aqueous secretion , although this is likely to be minimal as stated below.
Under certain conditions, pilocarpine may increase pseudofacility. , Using a variety of species, conditions, and experimental techniques, cholinergic agents or parasympathetic nerve stimulation is reported to increase, decrease, or not alter the aqueous humor formation rate and to increase slightly the episcleral venous pressure. These apparently confusing results may indicate that cholinergic drug effects on these parameters are extremely dependent on species and technique-related factors and on the ambient neurovascular milieu. In any event, the effects on the rate of aqueous humor formation and episcleral venous pressure are surely minor in most instances, and not responsible for the drug-induced decrease in IOP that forms the basis of the therapeutic efficacy of pilocarpine in chronic glaucoma; the latter resides in its ability to decrease outflow resistance via its effect on the ciliary muscle and perhaps, to a much lesser extent, directly on the TM (see Aqueous Humor Drainage ).
Adrenergic mechanisms
The precise role and receptor specificity of adrenergic mechanisms in regulating the rate of aqueous humor formation are unclear. At one time it was generally believed that long-term topical administration of epinephrine, a combined α 1 , α 2, β 1 , β 2 -adrenergic agonist, would decrease the rate of aqueous humor formation. This effect was thought to be mediated by β-adrenergic receptors in the non-pigmented ciliary epithelium, via activation of a membrane adenylate cyclase. Further, forskolin, which directly and irreversibly activates intracellular adenylate cyclase, in some, , albeit not all, studies decreases the rate of aqueous humor formation when given topically or intravitreally.
Fluorophotometric studies show that short-term topical administration of epinephrine increases aqueous humor formation; studies with other adrenergic agonists, including salbutamol, isoproterenol (isoprenaline), and terbutaline, support this finding and are consistent with many studies showing that β-adrenergic antagonists unequivocally decrease aqueous humor formation.
The ocular hypotensive action of β-antagonists led to their becoming mainstays of clinical glaucoma therapy: the non-selective β 1 , β 2 antagonists timolol, levobunolol, and metipranolol, the non-selective β 1 , β 2 partial agonist carteolol, , and the relatively β 1 selective antagonist betaxolol. Adrenergic receptors in the ciliary epithelium are of the β 2 subtype, but antagonists that are relatively selective for β 1 receptors (e.g. betaxolol) are effective (although less potent) in suppressing aqueous humor formation. However, the apparent β 1 efficacy may be related to a sufficiently high concentration reaching the ciliary epithelium so that non-selective blockade of β 2 receptors may occur.
It is questioned whether β-adrenergic antagonists suppress aqueous humor formation via their effect on ciliary epithelial β-adrenergic receptors. There is evidence that classical β-adrenergic receptor blockade may not be involved, and that other receptor types such as 5HT 1A , may be relevant (see Other Agents ). Furthermore, Na/K/2Cl cotransport stimulated by epinephrine and isoproterenol can be inhibited by β 2 -adrenergic receptor antagonists in human fetal non-pigmented ciliary epithelial cells.
Aqueous humor formation is reduced by nearly 50 percent during sleep, due to the β-arrestin/cAMP cascade regulation of signal transduction from the β-adrenergic receptor to the ciliary epithelium. This results in a circadian fluctuation of IOP. However it is suggested that IOP may actually increase in humans at night and that technical limitations may be responsible for previously reported IOP decreases. , Nocturnal IOP elevation may play a more important role in glaucomatous progression than previously appreciated. β-Antagonists produce little additional reduction in aqueous humor formation during sleep or in pentobarbital-anesthetized monkeys. Therefore adrenergic-based glaucoma therapies which target aqueous humor formation reduction may be less efficacious for combating the progression of glaucomatous damage than therapies targeting other mechanisms.
Sympathetic fibers synapse in the superior cervical ganglion and distribute to the muscles and blood vessels of the ciliary body, regulating blood flow to the ciliary process vasculature (see Chapter 10 ). Catecholaminergic and NPY-ergic nerve fibers preferentially supply the vasculature and epithelium of the monkey anterior ciliary processes, suggesting that they assist in the precise regulation of aqueous humor formation. Stimulation of the cervical sympathetic nerves in vervet monkeys significantly increases the rate of aqueous humor formation. However adrenergic tone to the ciliary epithelium may be humoral catecholaminergic rather than sympathetic innervational. Two major hormones of the adrenal gland, epinephrine and cortisol can regulate aqueous formation. Aqueous flow in human subjects correlates with circulating catecholamine levels during sleep and wakefulness. Adrenergic drugs may exert their effect by causing localized constriction in the arterioles that supply the ciliary processes.
Topically applied α 1 -adrenergic agonists and antagonists appear to have little effect on fluorophotometrically determined aqueous humor formation in the normal intact human eye. , Clonidine, which has both α 1 -antagonist and α 2 -agonist properties, decreases aqueous humor formation and ocular blood flow. Therefore epinephrine may have a dual effect on aqueous humor formation: stimulation via β-adrenoceptors, and inhibition via α 2 -adrenoceptors. α 2 -Adrenergic agonists, such as apraclonidine and brimonidine, are powerful ocular hypotensive agents when applied topically, and lower IOP primarily by decreasing aqueous humor formation. , However, one study in humans suggests that brimonidine suppresses aqueous humor formation early on, but after 8–29 days of treatment the predominant effect is on uveoscleral outflow.
Other agents
There are many other ways in which aqueous humor formation can be reduced pharmacologically ( Table 11.2 ). Effective compounds include: the guanylate cyclase activators atrial natriuretic factor , and the nitrovasodilators sodium nitroprusside, , sodium azide, and nitroglycerin. 8-Bromo cyclic GMP also reduces the aqueous humor formation rate by 15–20 percent in the monkey. Atrial natriuretic factor injected intravitreally reduces IOP and aqueous humor formation in rabbits and monkeys. However, intracameral and intravenous administration of atrial natriuretic factor to monkeys increases aqueous humor formation. Atrial natriuretic factor levels are elevated in aqueous humor of rabbits following topical treatment with kappa opioid agonists, which results in IOP reduction and aqueous humor formation suppression. However, non-kappa opioid mechanisms are responsible for aqueous humor formation and IOP reductions in monkeys. Nitric oxide may be involved in mediating the IOP lowering response to mu3 opioid agonists in rabbits.
I. General | II. Systemic | III. Local | IV. Pharmacologic | V. Surgical |
---|---|---|---|---|
A. Age | A. Artificial reduction in internal carotid arterial blood flow | A. Increased IOP (pseudofacility) | A. β-Adrenoceptor antagonists (e.g. timolol, betaxolol, levobunolol , carteolol, metipranolol) | A. Cyclodialysis |
B. Diurnal cycle | B. Diencephalic stimulation | B. Uveitis (especially iridocyclitis) | B. Carbonic anhydrase inhibitors | B. Cyclocryothermy |
C. Exercise | C. Hypothermia | C. Retinal detachment | C. Nitrovasodilators; atrial natriuretic factor (route and species dependent) | C. Cyclodiathermy |
D. Acidosis | D. Retrobulbar anesthesia | D. 5-HT 1A antagonists (e.g. ketanserin) | D. Cyclophotocoagulation | |
E. General anesthesia | E. Chroroidal detachment | E. DA 2 agonists (e.g. pergolide, lergotrile, bromocriptine) | ||
F. α 2 -Adrenoceptor agonists (e.g. apraclonidine, brimonidine) | ||||
G. Opioid agonists | ||||
H. Δ9-Tetrahydrocannabinol (Δ9 – THC) | ||||
I. Metabolic inhibitors (e.g. DNP, fluorocetamide) | ||||
J. Cardiac glycosides (e.g. ouabain, digoxin) | ||||
K. Spironolactone | ||||
L. Plasma hyperosmolality | ||||
M. cGMP |
The serotonergic antagonist ketanserin reduces the aqueous humor formation rate in rabbits, cats, and monkeys. Serotonergic receptors of a 5-HT l A -like subtype are reported to exist in the iris-ciliary body of rabbits and humans. These receptors may be antagonized by timolol and other β-blockers. The 5-HT lA agonist 8-OH-DPAT dose-dependently decreases IOP in normotensive rabbits during light and dark cycles. , The more selective 5-HT lA agonist flesinoxan also decreases IOP in rabbits. However, the precise nature of the putative 5-HT lA -like receptor subtype in the ciliary epithelium is still in question. Also, IOP and aqueous flow suppression of 5-HT lA receptor agonists in non-human primates is variable. A selective 5-HT2 agonist increases aqueous humor formation following topical treatment in monkeys but lowers IOP by increasing uveoscleral outflow.
Metabolic inhibitors such as dinitrophenol decrease aqueous humor formation as do the cardiac glycosides ouabain and digoxin, which inhibit the ciliary epithelial Na + -K + -ATPase enzyme. , None of these has yet been shown to be useful clinically as glaucoma therapeutics agents. Ibopamine exhibits activity at dopaminergic and adrenergic receptors. In humans, it can increase aqueous humor formation but can decrease or increase IOP. ,
A component of marijuana (cannabis), Δ 9 -tetrahydrocannabinol, reduces secretion of aqueous in human volunteers when injected intravenously or when inhaled via marijuana smoking. In contrast, topical Δ 9 -tetrahydrocannabinol has no effect on the human eye. , Studies demonstrate the presence of functional CB1 receptors in the ciliary processes and TM of human and animal tissue. Topical application of the cannabinoid receptor agonist, WIN-55-212-2, significantly reduces aqueous humor formation in normal and glaucomatous cynomolgus monkeys, but without increasing outflow facility. The decrease in aqueous humor formation after a single application is insufficient to explain the reduction in IOP, suggesting that other mechanisms are involved. The endocannabinoid, 2-arachidonylglyceral, increases outflow facility in a porcine organ culture system by an effect mediated through both CB1 and CB2 cannabinoid receptors and changes in the actin cytoskeleton in porcine TM cells.
Other local, systemic, and surgical factors can reduce aqueous humor formation rate, as can age and exercise ( Table 11.2 ). In a study of 300 normal volunteers, ages 5–83 years, there is an average decline in aqueous flow of 25 percent between the ages of 10–80 years. A smaller decline occurs in humans over 65 years of age. There is no age-related decline in aqueous humor formation in rhesus monkeys 25–29 years of age (human equivalent 62–73 years) compared to those 3–10 years or 19–23 years of age.
Long-term use of drugs that decrease IOP by decreasing aqueous humor formation could adversely affect the eye. During the course of several weeks of oral acetazolamide therapy in humans, IOP gradually reverts toward pretreatment values but tonographic outflow facility is reduced. Similarly, monkeys receiving prolonged treatment with timolol show a reduction in outflow facility. Underperfusion of the TM can lead to detrimental morphologic effects.
Aqueous humor drainage
Fluid mechanics
The tissues of the anterior chamber angle normally offer a certain resistance to fluid outflow. IOP builds up, in response to the inflow of aqueous humor, to the level sufficient to drive fluid across that resistance at the same rate it is produced by the ciliary body; this is the steady-state IOP. In the glaucomatous eye, this resistance is often unusually high, causing elevated IOP. Results from three major clinical trials confirm the value of reducing IOP in patients with ocular hypertension or glaucoma to prevent the onset of glaucoma in the former case and the progression of disease in the latter. Understanding the factors governing normal and abnormal aqueous humor formation, aqueous humor outflow, IOP, and their interrelationships and manipulation is vital in understanding and treating glaucoma.
Briefly, let:
- •
F = flow (µl/min)
- •
F in = total aqueous humor inflow: human = ~2.5 µl × min −1 .
- •
F S = inflow from active secretion
- •
F f = inflow from ultrafiltration
- •
F out = total aqueous humor outflow
- •
F trab = outflow via trabecular pathway
- •
F u = outflow via uveoscleral pathway, 0.3 µl/min, measured invasively by radioactive tracer, in primarily elderly human eyes with malignant melanoma, tumor or glaucoma; 1.64 µl/min in normal subjects age 20–30 yrs and 1.16 µl/min in subjects >60 years, measured by non-invasive fluorophotometry
- •
P = pressure (mmHg)
- •
P i = IOP: humans = 16 mmHg
- •
P e = episcleral venous pressure: human = 9 mmHg ,
- •
R = resistance to flow (mmHg × min/µl)
- •
C = facility or conductance of flow (µl/min/mmHg) = 1/R
- •
C tot = total aqueous humor outflow facility (OF): humans age 20–30 years, 0.25 µI/min/mmHg; >60 years, 0.19 µI/min/mmHg; OF = 0.54 − (0.0042 × age in years) µl/min/mmHg; 0.24 µl/min/mmHg; <40 years, 0.33; >60 years, 0.23 µl/min/mmHg; 0.28 µl/min/mmHg, all measured by indentation tonography
- •
C trab = facility of outflow via trabecular pathway: humans 20–30 years, 0.21 µl/min/mmHg; >60 years, 0.25 µl/min/mmHg (fluorophotometry); 0.22 µl/min/mmHg (tonography)
- •
C u = facility of outflow via uveoscleral pathway: humans = 0.02 µl/min/mmHg this was probably based on reported monkey values ,
- •
C ps = facility of inflow: human = 0.06 µl/min/mmHg; 0.08 µl/min/mmHg. These values for C ps are most likely overestimates: under normal circumstances in a non-inflamed eye the phenomenon is negligible; values for the normal monkey measured by a more precise tracer technique are <0.02 µl/min/mmHg ,
Then:
F in = F S + F t
F out = F trab + F u
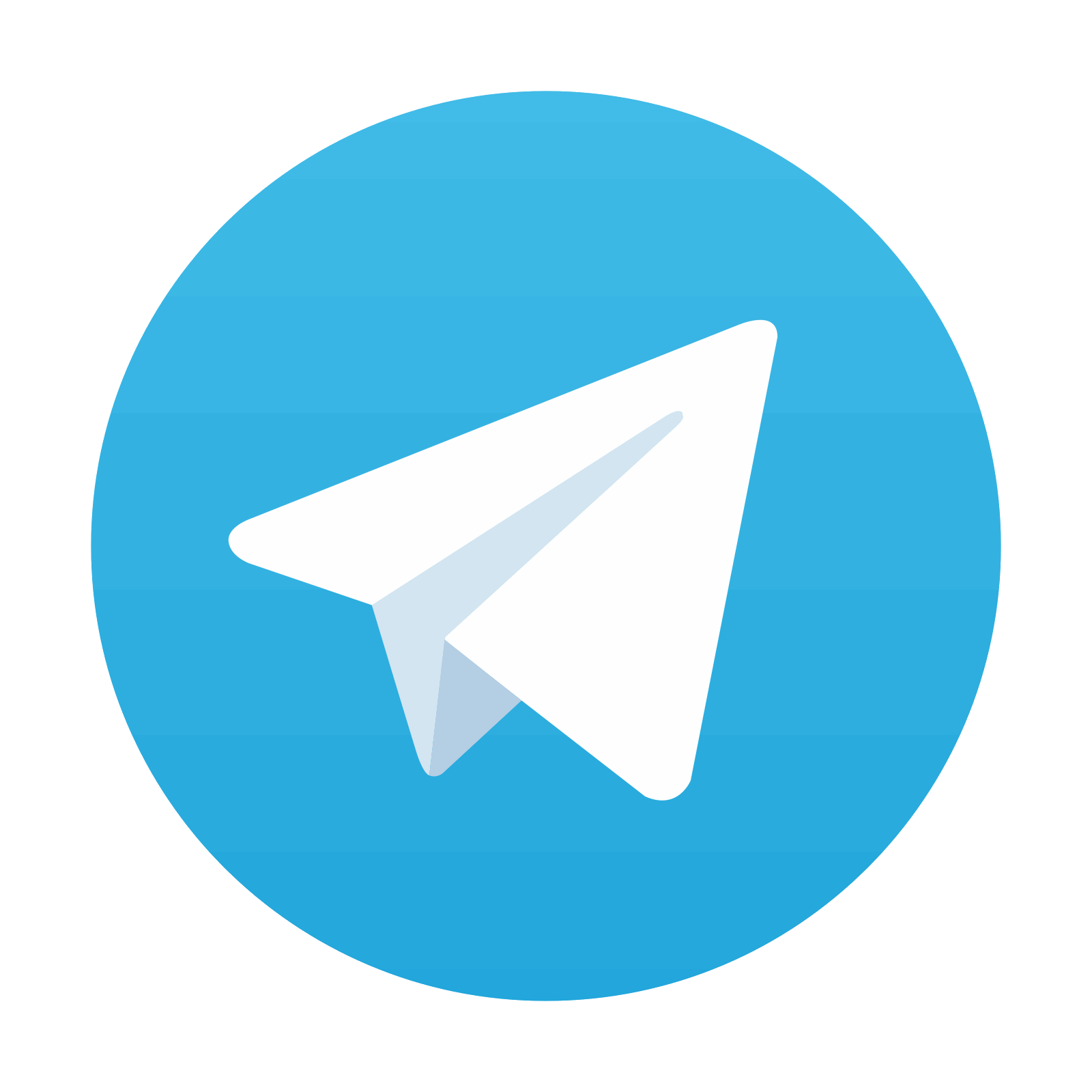
Stay updated, free articles. Join our Telegram channel

Full access? Get Clinical Tree
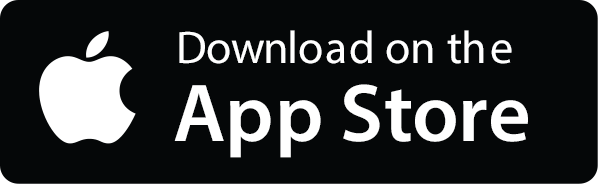
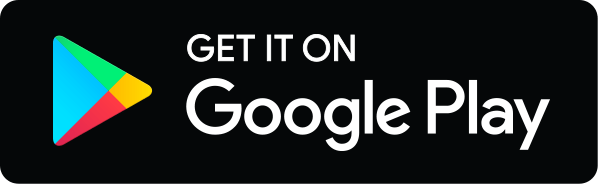