Introduction
Intraocular pressure (IOP) is an essential feature of the eye. It influences the shape and curvature of the globe, thus indirectly affecting the eye’s optical performance. The pressure is the result of the continuous entry of fluid, aqueous humor, into the eye and the hydraulic resistance that restricts its exit. The value for normal IOP is often given as approximately 15 mmHg, but it is more appropriate to consider a normal range, which in humans is from 10 to 21 mmHg. This reflects individual differences and the fact that IOP is higher in the day and lower at night. This fluctuation is primarily due to aqueous humor inflow following a circadian rhythm.
The flow of aqueous humor though the anterior eye provides nutrients to the avascular trabecular meshwork (TM), cornea, and lens. The latter two are adapted for transparency and thus optical performance. The anterior and posterior chambers are filled with clear aqueous humor and are important parts of the eye’s light path. In fact, the overall refractive power of the eye is influenced by the refractive index of the aqueous humor (1.33332), more specifically the refractive index difference at the cornea–aqueous humor interface, and the aqueous humor–lens interface . As a transparent and colorless liquid, aqueous humor is different in composition from plasma. Owing to its ultra-low protein content and cell-free composition, aqueous humor has the required optical properties of light transmittance in the visible spectrum with low scatter.
Aqueous humor is formed by the ciliary processes, which are located at the posterior of the iris root. The fluid enters the posterior chamber and flows across the anterior surface of the lens. It passes through the pupil into the anterior chamber, in which there is evidence of convective mixing that follows a pattern of downward fluid movement close to the cornea, where evaporation causes the temperature to be cooler, and upward movement near the iris, where the temperature is warmer . To exit the eye, a large fraction of aqueous humor fluid passes through the TM at the anterior chamber angle, then into Schlemm’s canal (SC), driven by the hydrostatic pressure force of IOP. Hydrostatic pressure also causes a significant amount of fluid to exit by seeping through extracellular spaces in the iris root, ciliary muscle, and other uveal structures. This is termed unconventional outflow. The pathways of aqueous humor flow are shown schematically in Figs. 10.1 and 10.2 .


Aqueous humor production is a good example of homeostasis. Whereas aqueous humor inflow is influenced by factors such as age and exercise, the rate of aqueous humor formation is remarkably stable . In a study of 300 normal volunteers aged 5 to 83 years, the age-related decline in aqueous flow was found to be minor, although a detectable decline occurs in humans over 65 years of age . There is no age-related decline in aqueous humor formation in rhesus monkeys 25 to 29 years of age (human equivalent 62–73 years) compared with those 3 to 10 years or 19 to 23 years of age .
Structure of the ciliary body and ciliary epithelium
The ciliary body is a secretory tissue, as well as the location of the ciliary muscle and the anchor for the zonules that support the lens . The surface of the ciliary body is structured in a way that provides a large surface area for fluid production. The surface that faces the aqueous humor is folded to form multiple radially oriented ridges, ciliary processes . The ridges are most pronounced anteriorly in the pars plicata region, and less well defined in the pars plana region of the ciliary body near the retina. The ridges, as well as the valleys in between, are covered with a unique epithelial formation, a bilayer made of two entirely different epithelial cell types. The nonpigmented ciliary epithelium cell layer (NPE) that faces the aqueous humor is developmentally related to the neural retina. The pigmented ciliary epithelium cell layer (PE) underneath is developmentally related to the retinal pigment epithelium. As the name indicates, PE cells contain black pigment granules. Nonpigmented cells are larger and contain a greater number of mitochondria than pigmented cells, which indicates higher metabolic activity in the nonpigmented cell layer. The architecture of the ciliary epithelium is unique. The two cell types are arranged with their apical surfaces touching. Thus, the apical-basolateral orientation of the pigmented ciliary epithelium aligns with the orientation of the retinal pigment epithelium. The basolateral surface of the NPE faces the aqueous humor and displays a high degree of invagination . This infolding of basolateral plasma membrane of the nonpigmented ciliary epithelium is another specialization that equips the ciliary body with a large surface area. Together, the ridges and valleys of the ciliary processes and the invaginations on the surface of each nonpigmented ciliary epithelial cell provide a huge surface for the ion transport mechanisms that power fluid production.
The ciliary epithelium bilayer is draped over an elaborate network of blood vessels that occupies the interior of the ciliary processes. In broad terms, the ciliary epithelium forms aqueous humor by modifying blood plasma in various ways. It follows that the rate of aqueous humor production is dependent, to an extent, on the rate of blood flow to the ciliary body. Importantly, blood flow is subject to regulation. Throughout the body, factors such as norepinephrine and certain prostaglandins cause blood vessels to constrict, while other factors such as nitric oxide bring about relaxation. Blood vessel diameter is governed by the balance between relaxing factors and constricting factors. Using a microcasting technique, investigators studying blood flow within the ciliary processes demonstrated vasoconstriction in eyes treated with catecholamines . Freddo has suggested that blood flow in the ciliary body microvasculature is under regional control because there are reports of localized constrictions . Unlike the capillaries that serve the ciliary muscle, the capillaries in the ciliary process are fenestrated and leaky. Studies using horseradish peroxidase as a tracer demonstrate the permeability of the capillaries to macromolecules, and presumably ions and water . In effect, the permeability barrier is the ciliary epithelium, not the ciliary body microvasculature.
Aqueous humor composition
The ciliary body defines the composition of aqueous humor, as well as its rate of formation. Large macromolecules in the ciliary body stroma are unable to diffuse across the ciliary epithelium. As a consequence, the most obvious difference between the composition of aqueous humor and plasma is the low protein concentration in the aqueous humor (200 times less than plasma, Table 10.1 ) ( #1071, ). There may be slight regional differences in protein concentration. For example, in the peripheral portion of the anterior chamber, close to the TM, the protein concentration may be higher than in the more central region because of protein entry directly from the peripheral iris, as demonstrated in monkey and human eyes . Owing to its contact with multiple specialized tissues in the anterior eye, proteomic analysis of aqueous humor biomarkers has the potential to provide insights into disease mechanisms . As mentioned previously, low protein concentration makes for efficient light transmission through the aqueous humor. When the protein concentration rises much above its normal 20 mg/100 mL , as in uveitis, the resultant light scattering (Tyndall effect) makes the slit-lamp beam visible as it traverses the anterior chamber (a phenomenon known as “flare”).
Rabbit | Human | ||||
---|---|---|---|---|---|
Concentration (mmol/kg H 2 O) | Anterior aqueous humor | Posterior aqueous humor | Plasma | Aqueous humor | Plasma |
Na + | 138 | 159 | 143 | 163 | 146 |
K + | 4.3 | 4.7 | 4.6 | ||
Cl − | 101 | 97 | 108 | 134 | 109 |
HCO 3 − | 30 | 34 | 25 | 20 | 28 |
Ascorbate | 1.1 | 1.4 | 0.04 | 1.06 | 0.04 |
Lactate | 9.3 | 9.9 | 10.3 | ||
Glucose | 6 | 6 | 6 | 3 | 6 |
In humans and many other species, a particularly striking feature of aqueous humor composition is its high ascorbate concentration ( Table 10.1 ). In guinea pigs the aqueous humor ascorbate concentration is almost 60 times that of plasma ; in humans the concentration is 20 times higher . The high ascorbate concentration likely protects the anterior ocular structures from ultraviolet light–induced oxidative damage . In addition to being an antioxidant, ascorbate may regulate structure and function of the TM , and may partially absorb ultraviolet radiation . Extensive and repeated oxidative stress in vivo may result in reduced TM cell adhesion, leading to TM cell loss, which is associated with glaucomatous conditions . It is noteworthy that diurnal mammals have approximately 35 times the concentration of aqueous ascorbate compared with nocturnal mammals .
The high concentration of ascorbate in aqueous humor reflects active transport by the ciliary epithelium . In mammalian cells, ascorbic acid, the reduced form, is transported by the sodium dependent L-ascorbic acid transporters SLC23A2 and SLC23A1, whereas the oxidized form, dehydro-ascorbic acid, is transported by the dehydro-ascorbic acid, is transported by the family of facilitated glucose transporters . SLC23A2 is expressed in the PE of human donor eyes but is absent in mouse, a nocturnal species . Diminished ascorbate levels in the aqueous humor have been observed in subjects with various forms of glaucoma, and a single-nucleotide polymorphism (SNP) in SLC23A2 is associated with a higher risk of primary open-angle glaucoma (POAG) .
The concentration of glucose, urea, and nonprotein nitrogen is reportedly slightly lower in aqueous humor than in plasma, while there is an excess of Cl − and certain amino acids ( Table 10.1 ). MicroRNAs are detectable in aqueous humor , likely protected by extracellular nanovesicles known as exosomes . It has been suggested that these endogenous noncoding RNAs might have a role in signaling between cells, and there is interest in whether they may also serve as disease biomarkers . Oxygen levels are low in the aqueous humor, and there are differences between pO 2 in various regions of the anterior and posterior chamber . Lactate concentration is high in the aqueous, presumably because of glycolytic activity of the lens, cornea, and other ocular structures .
Physiology of aqueous humor formation
Kiel and colleagues eloquently summarized aqueous humor formation as a three-stage process: (1) delivery of water, ions, proteins, and metabolic fuel by the ciliary circulation, (2) ultrafiltration and diffusion from the capillaries into the stroma under the influence of oncotic pressure, hydrostatic pressure, and concentration gradients, and (3) active ion transport across the ciliary epithelium followed by water movement down the resultant osmotic gradient into the posterior chamber. The hydrostatic pressure driving force for ultrafiltration across the ciliary epithelium is diminished by an opposing oncotic pressure gradient due to the relative lack of protein in aqueous humor compared with the ciliary process stroma . Put simply, aqueous humor production involves diffusion and ultrafiltration but is fundamentally an active process requiring the expenditure of metabolic energy . Active transport of sodium ions into the posterior chamber by the NPE results in water movement from the stromal pool into the posterior chamber. There is general agreement that under normal conditions active secretion accounts for perhaps 80% to 90% of total aqueous humor formation . The observation that moderate alterations in systemic blood pressure and ciliary process blood flow have relatively little effect on aqueous humor formation rate supports this notion . Furthermore, Bill noted that the hydrostatic and oncotic forces that exist across the ciliary epithelium (between the posterior aqueous humor and the ciliary body stroma) favor resorption, not ultrafiltration-mediated production, of aqueous humor. Active secretion is essentially pressure-insensitive at near-physiologic IOP. However, the ultrafiltration component of aqueous humor formation is sensitive to changes in IOP, decreasing with increasing IOP. This phenomenon is quantifiable and is termed facility of inflow, or pseudofacility, the latter because a pressure-induced decrease in inflow will appear as an increase in outflow when techniques such as tonography and constant-pressure perfusion are used to measure outflow facility ( #1072). Although measurements vary, pseudofacility in the noninflamed monkey and human eye constitute a very small percentage of total facility . Recently it has been argued that fluid transport rates exhibited by the ciliary epithelia may not be sufficient to account for the rate of aqueous humor formation and there may be some contribution from fluid directly entering the anterior chamber across the anterior surface of the iris .
In most mammalian species, the turnover number for aqueous humor in the anterior chamber is approximately 0.01 to 0.015 per minute, that is, the rate of aqueous humor formation and drainage is approximately 1% to 1.5% of the anterior chamber volume per minute . This is true also in the normal human eye, in which the aqueous formation rate is approximately 2.5 µL per minute . Several studies have provided comprehensive theoretical analyses of the fluid mechanics of aqueous production and can be found elsewhere .
Aqueous humor as a secretion
If aqueous humor was formed simply by ultrafiltration of plasma, the concentrations of ions in aqueous humor would fit the predictions of a Gibbs-Donnan equilibrium, but this is not the case . In fact, aqueous humor contains a slight excess of sodium, as active fluid secretion is powered by Na,K-ATPase in the ciliary epithelium ( Table 10.1 ). Na,K-ATPase transports Na + ions from the NPE into the posterior chamber and establishes ion gradients that support the coordinated activity of several different ion transporters and channels arranged at specific locations in the two cell layers of the bilayer (detailed further in chapter).
The architecture of the ciliary epithelium is unique . The bilayer consists of the PE facing the ciliary stroma and the NPE facing the aqueous humor. The cells are extensively coupled via connexin (Cx) gap junctions, forming a syncytium-like structure. In rabbit and rat, Cx50 is found in the NPE layer while Cx43 is abundant in the region where the NPE-PE apical surfaces touch , although other connexins might also be expressed . Neighboring cells in each layer are coupled and the two cell layers are connected to each other at their apical membranes . Tight junctions are present between the apical borders of NPE cells, where they form a barrier that restricts paracellular diffusion . It should be kept in mind that the functional properties of tight junctions, as well as gap junctions, are subject to dynamic regulation. The ciliary epithelium is a living barrier that can sense and respond to external stimuli.
The process of fluid secretion is mediated via active transport of ions across the bilayer . Two enzymes abundantly present in the NPE are intimately involved in this process: Na,K-ATPase and carbonic anhydrase (CA) . Na,K-ATPase is found predominantly bound to the plasma membrane of the basolateral infoldings of the NPE .
Primary active transport by Na,K-ATPase establishes a Na + concentration gradient across the plasma membrane of the coupled NPE and PE cells. The NPE is particularly rich in Na,K-ATPase and is one of few cell types that expresses the α1, α2, α3, β1, and β2 isoforms . The pattern of Na,K-ATPase isoform expression suggests functional differences between the pars plana and pars plicata . Other ions and molecules are transported by secondary active transport mechanisms that use the Na + gradient as a driving force. Thus, aqueous humor in humans exhibits increased levels of Na + and C − compared with plasma . The bilayered epithelium has Na + -coupled transporters for some amino acids, L-ascorbic acid, glucose transporters, and Cl − /HCO 3 − exchange transporters . Another ATPase, an H + -ATPase proton pump, has also been detected in the NPE basolateral surface , and other solute transporters have been detected by single cell–RNA sequencing analysis . To maintain electroneutrality, anions must accompany the actively secreted Na + . It is thought that Cl − passes through chloride channels in the basolateral membrane and HCO 3 − can enter aqueous via exchange with Cl − . Gap junctions permit diffusion between the cell layers, enabling transporters in the NPE and PE to operate in a coordinated manner to pass solutes across the bilayer. The principal function of the ciliary epithelium, net transport of solutes and water in a blood to aqueous direction, is dependent on the specific localization of transporters and channels on either the stromal-facing or aqueous humor–facing surfaces of the bilayer. A simplified scheme is shown in Fig. 10.3 . Based on studies in the rabbit, it has been proposed that the arrangement of transporters and channels in the anterior of the ciliary processes is different and this part of the ciliary body might absorb solutes and fluid . If this is the case, stimulation of reabsorption would have the effect of reducing the rate of net aqueous humor formation, and thereby IOP.

Selective inhibition of Na + -K + -ATPase activity by cardiac glycosides (e.g., ouabain) significantly reduces the rate of aqueous humor formation and IOP in experimental animals and humans , consistent with the notion that active transport of Na + is the primary driving force for the secretion of aqueous humor. Vanadate, a different Na,K-ATPase inhibitor was also found to reduce aqueous humor formation although later studies suggest it might inhibit additional mechanisms . The activity of Na,K-ATPase activity in the NPE may be subject to endogenous regulation by protein kinase signaling pathways that are activated by nitric oxide, cAMP, cytoplasmic calcium, insulin, and other factors .
The metabolically driven, ATP-dependent active transport of Na + by Na,K-ATPase, and the accompanying movement of anions, creates an osmotic force that drives the osmotic movement of water across the invaginated basolateral surface of the NPE cells . The theoretical basis for coupling the flow of ions and water is described elsewhere . The movement of water is facilitated by water channels, aquaporins AQP1 and AQP4, in NPE cells . AQP1 null mice have decreased IOP and aqueous humor production compared to normal . Na + and Cl − enter the bilayer via the basolateral surface of the PE cells. This involves transport by Na + /H + and Cl − /HCO 3 − exchange transporters and the Na-K-2Cl cotransporter. It follows that inhibition or activation of the various transporters and channels in the ciliary epithelium bilayer can alter the rate of formation of aqueous humor . The contribution of anion transport, particularly HCO 3 − , is critical but far from straightforward because it is not the same in all species . HCO 3 − transport is intimately linked to CA enzymes . Transport of another anion, Cl − , also plays an important role in the aqueous humor formation . In isolated bovine eyes, the principal mechanisms responsible for aqueous humor formation are Na,K-ATPase-mediated active transport and chloride transport . In rabbit preparations, the transfer of Cl − ions is enhanced by agonists of A3 adenosine receptors . A3 adenosine receptor agonists and antagonists, respectively, increase and lower IOP in mice . Bestrophin-2, a protein that regulates Ca 2+ -activated Cl − conductance, is localized to the NPE, suggesting that it may play a role in regulating aqueous humor formation. Mice deficient in bestrophin-2 have significantly diminished IOP .
In bovine eyes in vitro, 4,4′-diisothiocyanatostilbene-2,2′-disulfonic acid (DIDS, an inhibitor of the Cl − /HCO 3 − exchanger, Na-HCO 3 cotransporter, and chloride channels) and 5-nitro-2-(3-phenylpropylamino)-benzoic acid (NPPB, a chloride channel blocker) reduce aqueous humor formation by 55% and 25%, respectively . In mice, topical inhibitors of Na +- H + exchange lower IOP . Bumetanide (a specific inhibitor of Na-K-Cl cotransport) and furosemide (a nonspecific anion transport inhibitor) reduce aqueous humor formation by 35% and 45% in bovine eyes in vitro . These transport inhibitors may have both direct and indirect effects on aqueous humor formation as DIDS and Na-H exchange inhibitors are known to activate protein kinase signaling pathways that change Na,K-ATPase activity . Because the Na-K-Cl cotransporter can be stimulated by catecholamines (such as epinephrine, norepinephrine, isoproterenol, and dopamine), there was interest in Na-K-Cl inhibition as a strategy to reduce aqueous humor formation . However, topical application of bumetanide to mice or monkeys has no effect on IOP. Because aqueous secretion is an active process, it is not surprising that metabolic inhibitors such as dinitrophenol decrease aqueous humor formation, but none of these has yet been found useful for glaucoma therapy .
CA is abundant in the ciliary epithelium and is present both in the cytoplasm and basolateral plasma membranes of the NPE and PE . There are multiple CA isoenzymes, and CAII, CAIV, and Carbonic anhydrase XII (CAXII) all are known to be present in the ciliary body . CAs catalyze the hydration of CO 2 , speeding the conversion of CO 2 and H 2 O to carbonic acid and its subsequent dissociation to H + and HCO 3 − . This provides the HCO 3 − , which is essential for the active secretion of aqueous humor. CA inhibitors (CAIs) reduce the rate of aqueous humor formation in multiple species, regardless of whether they concentrate HCO 3 − into the aqueous humor. It is likely that inhibition of CA causes a decrease in HCO 3 − anions necessary for outward transport from the NPE along with Na + in order to maintain electroneutrality . However, CAIs reduce intracellular pH and this may inhibit Na + -K + -ATPase activity in the NPE , and decrease the driving force for Na-H exchange to import Na + from the stroma into the PE . In the case of orally administered CAIs, there is also an impact of systemic acidosis on inhibition of aqueous humor formation . CA inhibitors given systemically can reduce aqueous humor secretion by up to 50% and have been in use for clinical glaucoma therapy for over 50 years . For CAIs to be effective, over 99% of the CA activity must be inhibited and it was once thought that the topical CA inhibitor penetration to the ciliary epithelium would not be able to produce the required degree of CA inhibition. Fortunately, there are now available topically effective CA inhibitors such as dorzolamide and brinzolamide that achieve substantial (albeit usually not quite as much) reduction in IOP as the earlier oral CA inhibitors but without their systemic side effects
Coordination between the pigmented and nonpigmented epithelium
The NPE and PE work in a coordinated manner to form aqueous humor. The PE is specialized for solute entry while the NPE is specialized for solute exit in terms of transporter expression . Solute and water move through the two cells in the bilayer, and Brubaker calculated that a ciliary epithelial cell transports the equivalent of 30% of its own volume every minute . Clearly, the cells are at risk of swelling or shrinkage if there were to be a mismatch between the entry and exit of water that flows through the bilayer. It has been proposed that in corneal endothelium, as well as ciliary epithelium, transepithelial fluid transport might be associated with cell volume oscillation with pulsatile water entry and exit . It is possible that certain mechanosensitive ion channels, TRPV4 and TRPV1, have a role in maintenance of cell volume homeostasis in the bilayer . Jo and coworkers observed swelling-induced calcium responses in NPE but not PE. TRPV4 inhibition prevented the swelling-induced calcium responses, and they were absent in TRPV4 knockout mice. Some responses to TRPV4 channel activation are thought to involve the opening of hemichannels formed by unpaired connexins and there is evidence for such hemichannels at the aqueous humor–facing surface of the ciliary epithelium bilayer . The TRPV4 agonist GSK1016790A reduces IOP and TRPV4 knockout mice have elevated IOP . However, IOP is normal in TRPV4 knockout mice . Interpretation of IOP responses is far from straightforward because TRPV4 activation has an influence on both aqueous formation and drainage. TRPV4 has a role in regulating function of the TM and SC . In studies on the different IOP responses to systemic and topical administration of a TRPV4 antagonist, HC067047, a mechanistic link between TRPV4 and the primary cilium in TM cells has been considered important .
Blood-aqueous barrier
Tight junctions between adjacent NPE cells make the ciliary epithelium bilayer a physical barrier. The blood-aqueous barrier is a broader functional concept invoked to explain the very limited ability of large molecules such as proteins to pass from the circulation into the aqueous humor. For present purposes, the blood-aqueous barrier comprises the tight junctions of the ciliary process NPE, the inner wall endothelium of SC, the iris vasculature, and the outward directed–active transport systems of the ciliary processes . Tight junctions prevent plasma protein leakage across the iridial vascular endothelium although there may be limited diffusion into the iris stroma from the ciliary body . Tight junctions in the posterior iris epithelium prevent protein diffusing from the iris to the posterior chamber . The capillaries of the ciliary processes and choroid are fenestrated and thus leaky, but tight junctions in the NPE constitute an effective barrier to diffusion . The endothelia of the inner wall of SC prevent retrograde movement of solutes and fluid from the canal lumen into the TM and anterior chamber .
Trauma, certain pathologic conditions, and some drugs, cause breakdown of the blood-aqueous barrier ( Table 10.2 ) . This allows plasma components to enter the aqueous humor. Net fluid movement from blood to aqueous increases, but so does its IOP dependence (pseudofacility) . Total facility, as measured by IOP-altering techniques, cannot distinguish pseudofacility from total outflow facility (C tot ) and therefore erroneously records the pseudofacility component as increased C tot , therefore underestimating the extent to which the outflow pathways have been compromised by the insult. Under these circumstances, increased pseudofacility provides some protection against a precipitous rise in IOP; as IOP rises, aqueous inflow by ultrafiltration is partly suppressed, blunting (but not completely suppressing) further IOP elevation . Additionally, the inflammatory process that occurs during blood–aqueous barrier breakdown leads to a reduction in active secretion of aqueous humor, possibly via interference with active transport mechanisms . This in turn may actually produce ocular hypotony, despite compromised conventional outflow pathways (due to plasma protein blockage of the TM). Prostaglandin release during inflammation may contribute to the hypotony by increasing aqueous outflow via the unconventional route . When the noxious stimulus is removed, however, the ciliary body may recover before the TM, and the resulting normalization of the aqueous humor formation and unconventional outflow rates in the face of still-compromised conventional outflow pathways leads to elevated IOP, as seen from the modified Goldmann equation (Eq. 10.1):
IOP=[(AHF−Fu)/Ctrab]+Pe
I. Traumatic | II. Pathophysiologic | III. Pharmacologic |
---|---|---|
|
|
|
|
|
|
|
|
|
|
|
|
|
| |
|
| |
|
| |
| ||
| ||
| ||
| ||
|
where IOP = intraocular pressure, AHF = aqueous humor formation rate, F u = unconventional outflow, C trab = facility of outflow from the anterior chamber via the TM and SC, and P e = episcleral venous pressure (the pressure against which fluid leaving the anterior chamber via the TM and SC must drain) .
Active transport of organic molecules
Xenobiotic transporters evolved as part of a mechanism to clear potentially harmful organic molecules from tissues into the bloodstream for removal by the liver or kidneys. Problems arise because the transporters also carry therapeutic drugs. The aqueous humor compartment, along with other protected sites such as the brain or the placenta, excludes many organic molecules. The ciliary body transports organic molecules from aqueous humor to blood. This is a two-step process driven by multiple xenobiotic transporters that include uptake transporters OAT1, OAT3, NADC3, and Multidrug resistance protein (MRP) 4, as well as efflux transporters MRP2, p-glycoprotein, and BCRP . These mechanisms have exceptionally wide substrate specificity and pose challenges to ocular drug delivery because they extrude therapeutic molecules, as well as toxic molecules, from the eye . For example, penicillin and other antibiotics are actively transported out of the eye. It has been known for some time that prostaglandins are actively transported out of the eye and the prostaglandin transporter OATP2A1, also known as PGT, that operates in the ciliary epithelium is able to carry latanoprost, the prostaglandin F 2 α analog used for glaucoma therapy . The xenobiotic transport mechanisms in the ciliary epithelium bear a striking resemblance to those in the renal tubules and blood-brain barrier . Because of their obvious influence on drug distribution, their transport kinetics have been extensively characterized . However, more research is needed before we understand the normal day-to-day physiologic role of these, and other outwardly-directed transport mechanisms such as the sodium-iodide symporter . The various efflux transporters in ocular tissues are discussed in more detail in Chapter 17 .
Regulation of aqueous humor formation
The rate of aqueous humor formation is not static ( Box 10.1 ). IOP displays a circadian rhythm that is attributed to diminished production of aqueous humor during sleep . The reduction of flow during sleep is roughly comparable to the reduction achieved by CAI and β-adrenergic antagonist glaucoma drugs . The nighttime reduction in secretion is related, in large part, to the level of circulating catecholamines and possibly glucocorticoids . The understanding that the rate of aqueous humor production is subject to regulation, over the years guided development of several useful IOP-lowering drugs (see Aqueous humor drainage). Circadian patterns are synchronized by the suprachiasmatic nucleus, which resets peripheral clocks by mechanisms that include adrenal glucocorticoids and sympathetic nerves . Circadian changes of adenylate cyclase activity are detectable in the ciliary processes . Autonomic regulation of the ciliary epithelium function and, to a lesser extent, blood flow within the ciliary body, influence aqueous humor formation. Sympathetic and parasympathetic nerve terminals in the ciliary body arise from branches of the long and short posterior ciliary nerves. These nerve fibers are of both the myelinated and nonmyelinated variety. Parasympathetic fibers originate in the Edinger-Westphal nucleus of the third cranial nerve, run with the inferior division of this nerve in the orbit, and synapse in the ciliary ganglion ( Fig. 10.4 ) . Vasodilatory parasympathetic nerve fibers originating in the pterygopalatine ganglion are likely to release nitric oxide and vasoactive intestinal peptide (VIP) in addition to acetylcholine (see Chapter 6 ). Nerves displaying VIP immunoreactivity are also detected in the ciliary processes, posterior third of the ciliary muscle and around small to medium-sized blood vessels in the posterior uvea of the cat . Relatively few VIP-positive fibers are found in the ciliary processes of humans and monkeys . In the cat eye, nerve fibers containing pituitary adenylate cyclase–activating peptide (PACAP) are detected in the iris, ciliary body, and conjunctiva. PACAP immunoreactivity colocalizes with VIP in the sphenopalatine ganglion and with calcitonin gene–related peptide (CGRP) in the trigeminal ganglion . Sympathetic fibers synapse in the superior cervical ganglion and distribute to the muscles and blood vessels of the ciliary body. Stimulation of the cervical sympathetic nerves in vervet monkeys significantly increases the rate of aqueous humor formation . Numerous unmyelinated nerve fibers surround the blood vessels in the stroma of the ciliary processes. These are likely noradrenergic and control vascular tone .
- •
Aqueous humor production involves diffusion and ultrafiltration but is fundamentally an active process requiring the expenditure of metabolic energy.
- •
β-Adrenergic antagonists, such as timolol, that lower intraocular pressure (IOP) by decreasing aqueous humor formation, continue to be a mainstay in clinical glaucoma therapy.
- •
IOP displays a circadian rhythm that is attributed to diminished production of aqueous humor during sleep. There can be differences between the nocturnal and diurnal effectiveness of an IOP-lowering drug.
- •
α2-Adrenergic agonists such as apraclonidine and brimonidine are powerful ocular hypotensive agents, lowering IOP primarily by decreasing aqueous humor formation.
- •
In subjects treated with drugs that decrease outflow resistance (e.g., latanoprost), it is possible to obtain additional reduction of daytime IOP by adding carbonic anhydrase isoenzymes (CAIs) and β-adrenergic antagonists that reduce aqueous humor formation.

Various investigators have studied the consequences of eliminating autonomic input, and the results illustrate a degree of complexity in the control of aqueous formation. Experimental sympathetic denervation in monkeys does not alter resting aqueous humor formation and marginally affects the aqueous humor formation response to timolol or epinephrine . Studies have been done on individuals with Horner’s syndrome who have an interruption of sympathetic nerve supply to the eye. In human subjects with unilateral Horner’s syndrome, the rate of daytime aqueous humor formation, IOP, tonographic outflow facility, and the flow and IOP response to the β-adrenergic antagonist timolol are similar in both eyes . However, eyes with Horner syndrome show a decrease in aqueous humor formation in response to epinephrine, a combined α- and β-adrenergic agonist, instead of the normal increase. The β-adrenergic agonist isoproterenol, on the other hand, increases flow during sleep in both normal and Horner’s syndrome eyes but has no significant effect on flow in either during the day . Chemical sympathectomy with guanethidine sulfate in glaucomatous humans lowers IOP, presumably by reducing aqueous humor production measured indirectly by tonography .
The rate of aqueous humor secretion is increased by VIP and PACAP, two structurally related neuropeptides. The stimulation of aqueous formation following intravenous VIP administration in monkeys occurs secondary to activation of the sympathetic nervous system, while the effect of intracameral administration of VIP to the eye is a direct effect on the ciliary epithelium . PACAP appears to be slightly more potent than VIP as a stimulator of aqueous humor flow in the monkey . There are still unanswered questions about the role of sympathetic innervation in mediating aqueous inflow responses to pharmacologic agents, and species differences add to the challenge.
Cholinergic control of aqueous humor formation
The effects of cholinergic drugs on the rate of aqueous humor formation, its composition, and on the blood-aqueous barrier, are unclear. Overall, cholinomimetics have little effect on the volumetric rate of aqueous humor formation. In general, cholinergic drugs cause vasodilation in the anterior segment resulting in increased blood flow to the choroid, iris, ciliary processes, and ciliary muscle (see Chapter 11 ). However cholinergic drugs may also promote vasoconstriction in the rabbit eye . Congestion in the iris and ciliary body is a well-recognized clinical side effect of topical cholinomimetics, especially the anticholinesterases . The presence of flare and cells in the aqueous humor by biomicroscopy indicates that these agents can cause breakdown of the blood-aqueous barrier and perhaps frank inflammation . Pilocarpine increases blood-aqueous barrier permeability to iodide and inulin . Cholinergic drugs may alter the aqueous humor concentration of inorganic ions and the movement of certain amino acids from the blood into the aqueous humor, and may also influence the outward-directed transport systems of the ciliary processes . Studies in vitro indicate acetylcholine and carbachol can disrupt the gap junctions that couple PE and NPE cells . Because aqueous humor secretion requires the NPE and PE to work together in a coordinated manner, diminished coupling would be likely to contribute to inhibition of aqueous secretion. However, the cholinergic effect on aqueous humor formation appears minor and not entirely consistent across various species. Cholinergic agents or parasympathetic nerve stimulation is reported to elicit a range of responses that include increasing, decreasing, or not altering the aqueous humor formation rate . Under certain conditions, pilocarpine may increase pseudofacility . In short, the effects on the rate of aqueous humor formation is likely not a major factor in the drug-induced decrease in IOP that forms the basis of the therapeutic efficacy of pilocarpine in chronic glaucoma; the latter resides in its ability to decrease outflow resistance via its effect on the ciliary muscle (see Aqueous humor drainage).
Adrenergic control of aqueous humor formation
There is still much to be learned regarding the precise role and receptor specificity of adrenergic mechanisms in regulating the rate of aqueous humor formation. At one time it was generally thought that long-term topical administration of epinephrine, a combined α1, α2, β1, β2-adrenergic agonist, decreased the rate of aqueous humor formation . This effect was thought to be mediated by β-adrenergic receptors in the nonpigmented ciliary epithelium, via activation of a membrane adenylate cyclase . This line of thinking was supported by studies with forskolin, which directly and irreversibly activates intracellular adenylate cyclase. Forskolin was found to decrease the rate of aqueous humor formation when given topically or intravitreally . However, fluorophotometric studies show that short-term topical administration of epinephrine increases the rate of aqueous humor formation as did other adrenergic agonists, including salbutamol , isoproterenol (isoprenaline), and terbutaline . These results are consistent with studies showing that β-adrenergic antagonists alter aqueous humor formation .
The ocular hypotensive action of β-antagonists led to the development of drugs that became mainstays of clinical glaucoma therapy. Effective drugs include the nonselective β 1 , β 2 antagonists timolol , levobunolol , and metipranolol ,the nonselective β 1 , β 2 partial agonist carteolol , and the relatively β 1 -selective antagonist betaxolol . However, their pharmacology is not easy to explain. Adrenergic receptors in the ciliary epithelium are of the β 2 subtype , but antagonists that are relatively selective for β 1 receptors such as betaxolol are effective (although less potent) in suppressing aqueous humor formation . Nevertheless, the apparent β 1 efficacy may be related to a sufficiently high concentration reaching the ciliary epithelium so that nonselective blockade of β 2 receptors may occur . There has been some debate as to whether β-adrenergic antagonists suppress aqueous humor formation via their effect on β-adrenergic receptors in the ciliary epithelium. Some studies suggest that classical β-adrenergic receptor blockade may not be involved, and that other receptor types such as 5HT 1A , may be relevant . The reduced rate of aqueous humor formation during sleep has been linked to the β-arrestin–mediated regulation of the β-adrenergic G-protein–coupled receptors on the ciliary epithelium. β-antagonists produce little additional reduction in aqueous humor formation during sleep or in pentobarbital-anesthetized monkeys .
Sympathetic fibers synapse in the superior cervical ganglion and distribute to the muscles and blood vessels of the ciliary body, regulating blood flow to the ciliary process vasculature ( Fig. 10.4 ) (see Chapter 16 ). Catecholaminergic and Neuropeptide Y (NPY)-ergic nerve fibers preferentially supply the vasculature and epithelium of the monkey anterior ciliary processes, suggesting that they assist in the precise regulation of aqueous humor formation . Stimulation of the cervical sympathetic nerves in vervet monkeys significantly increases the rate of aqueous humor formation . However, the adrenergic tone of the ciliary epithelium may be more influenced by circulating catecholamines than sympathetic innervation. Two major hormones of the adrenal gland, epinephrine and cortisol, can regulate aqueous formation . Moreover, the pattern of aqueous flow in human subjects during sleep and wakefulness correlates with circulating catecholamine levels . Autonomic regulation of the ciliary epithelium function is consistent with the observation that ciliary epithelial cells express adrenergic receptors . Coupling between the NPE and PE cell layers likely allows coordination of signal transduction responses . Moreover, adrenergic agents are capable of changing the activity of ion transporters and gap junctions that support fluid secretion across the bilayer . Adrenergic drugs also may exert their effect by causing localized constriction in the arterioles that supply the ciliary processes .
Topically applied α1-adrenergic agonists and antagonists appear to have little effect on fluorophotometrically determined aqueous humor formation in the normal intact human eye . Clonidine, which has both α1-antagonist and α2-agonist properties, decreases aqueous humor formation and ocular blood flow . Therefore, epinephrine may have a dual effect on aqueous humor formation: stimulation via β-adrenoceptors, and inhibition via α2-adrenoceptors . α2-Adrenergic agonists, such as apraclonidine and brimonidine, are powerful ocular hypotensive agents when applied topically. Drugs such as brimonidine (Alphagan), and apraclonidine (Iopidine) lower IOP primarily by decreasing aqueous humor formation , although there may be effects on unconventional outflow, and responses in sleeping subject may be different . α2-Adrenergic agonists have been found effective when used alone, as well as with other IOP-lowering drugs in fixed combination formulations . For example, a fixed combination of two inflow-reducing drugs, brimonidine and a CAI brinzolamide, was found to have an additive IOP-lowering effect compared with a prostaglandin analog alone .
Carbonic anhydrase inhibitor effects on aqueous humor formation
As detailed previously, the physiology of the ciliary epithelium is highly dependent on CA, and CAIs have been used for many decades to reduce the rate of aqueous humor formation and lower IOP. The use of CAIs in the treatment of glaucoma was greatly increased by the development of topically effective CAIs, such as dorzolamide and brinzolamide , and the ability to use them in combination therapies with β-blockers and α2-agonists that have different mechanisms of action . Thus, in subjects treated with prostaglandin analog drugs like latanoprost that increase outflow, it is possible to obtain additional reduction of daytime IOP by adding CAIs and β-adrenergic antagonists that reduce aqueous humor formation .
Other pharmacological strategies
The rate of aqueous humor formation can also be reduced by a wide variety of agents from different drug categories ( Table 10.3 ) . This comprises guanylate cyclase activators, natriuretic peptides including atrial natriuretic peptide , neuropeptides , opioid receptor agonists , and the nitrovasodilators sodium nitroprusside , sodium azide , and nitroglycerin . Agents that increase cyclic GMP tend to reduce aqueous humor formation. This includes nitric oxide, atrial natriuretic peptide (ANP) , brain natriuretic peptide (BNP), and C-type natriuretic peptide (CNP), as well as peptide analogs more suitable for topical administration . It has been proposed that endogenous neuropeptides regulate fluid secretion 8-Bromo cyclic GMP also reduces the aqueous humor formation rate by 15% to 20% in the monkey . Interactions between pathways are likely. For example, ANP levels are elevated in the aqueous humor of rabbits following application of kappa opioid agonists to reduce aqueous humor formation suppression . Nitric oxide may be involved in mediating the IOP-lowering response to mu3 opioid agonists in rabbits .
I.General | II.Systemic | III.Local | IV.Pharmacologic | V.Surgical |
---|---|---|---|---|
A.Age | A.Artificial reduction in internal carotid arterial blood flow | A.Increased IOP (pseudofacility) |
| A.Cyclodialysis |
B.Diurnal cycle | B.Diencephalic stimulation | B.Uveitis (especially iridocyclitis) |
| B.Cyclocryothermy |
C.Exercise | C.Hypothermia | C.Retinal detachment |
| C.Cyclodiathermy |
D.Acidosis | D.Retrobulbar anesthesia |
| D.Cyclophotocoagulation | |
E.General anesthesia | E.Choroidal detachment |
| ||
| ||||
| ||||
| ||||
| ||||
| ||||
| ||||
| ||||
|
Serotonin (5-hydroxytryptamine (5-HT)) receptors are expressed in the ciliary epithelium and 5-HT agonists have been proposed as IOP-lowering agents . The serotonergic antagonist ketanserin reduces the aqueous humor formation rate in rabbits, cats, and monkeys . Serotonergic receptors of a 5-HT l A -like subtype are reported to exist in the iris-ciliary body of rabbits and humans . It may be significant that these receptors seem to be activated by timolol and other β-blockers . The 5-HT lA agonist 8-OH-DPAT dose-dependently decreases IOP in normotensive rabbits during light and dark cycles . The more selective 5-HT lA agonist flesinoxan also decreases IOP in rabbits . However, the precise nature of the putative 5-HT lA -like receptor subtype in the ciliary epithelium is still in question. Also, IOP and aqueous flow suppression of 5-HT lA receptor agonists in nonhuman primates is variable .
The ability of cannabinoids and endocannabinoids to reduce aqueous humor formation has received considerable interest . It has been known for some time that an active component of marijuana (cannabis), ∆ 9 -tetrahydrocannabinol, reduces secretion of aqueous in human volunteers when injected intravenously or when inhaled via marijuana smoking. In contrast, topical ∆ 9 -tetrahydrocannabinol has no effect on the human eye . Studies demonstrate the presence of functional CB1 cannabinoid receptors, as well as endogenous cannabinoids, in the ciliary processes and TM of human and animal tissue . Topical application of the cannabinoid receptor agonist, WIN-55-212-2, significantly reduces aqueous humor formation in normal and glaucomatous cynomolgus monkeys .
Aqueous humor drainage
Fluid mechanics
The tissues of the anterior chamber angle of the eye normally generate resistance to aqueous humor outflow. In response to the inflow and accumulation of aqueous humor, IOP builds up to the level sufficient to drive fluid across that resistance at the same rate it is produced by the ciliary body; this is the steady-state IOP. In the glaucomatous eye, this resistance is often unusually high, causing elevated IOP. Results from four major clinical trials confirm the value of reducing IOP in patients with ocular hypertension or glaucoma to prevent the onset of glaucoma in the former case, and the progression of disease in the latter . Understanding the factors governing normal and abnormal aqueous humor formation, aqueous humor outflow, IOP, and their interrelationships and manipulation is vital in understanding and treating glaucoma.
The factors can be mathematically represented as follows:
- •
F = flow (µL/min)
- •
F in = total aqueous humor inflow: human = approximately 2.5 µL per minute .
- •
F S = inflow from active secretion
- •
F f = inflow from ultrafiltration
- •
F out = total aqueous humor outflow
- •
F trab = outflow via the conventional (trabecular) pathway
- •
F u = outflow via the unconventional pathway (includes uveoscleral and uveovortex pathways), appears to decrease with age; with older eyes ranging from 0.3 to 1.16 µL/min and younger eyes at 1.64 µL/minute
- •
P = pressure (mmHg)
- •
P i = IOP: humans = 16 mmHg
- •
P e = episcleral venous pressure: human = approximately 9 mmHg
- •
R = resistance to flow (mmHg × min/µL)
- •
C = facility or conductance of flow (µL/min per mmHg) = 1/R
- •
C tot = total aqueous humor outflow facility: measurements in healthy humans 0.19 to 0.28 µL/min per mmHg
- •
C trab = facility of outflow via trabecular pathway: measurements in healthy humans range from 0.21 to 0.27 µl/min per mmHg
- •
C ps = facility of inflow, also known as pseudofacility, which reflects the decrease of aqueous humor flow in response to an increase in IOP. In healthy humans, measurement ranges from 0.06 to 0.08 µL/min per mmHg . These values for C ps are most likely overestimates: under normal circumstances in a noninflamed eye the phenomenon is negligible ; values for the normal monkey measured by a more precise tracer technique are <0.02 µL/min per mmHg .
Then (Eq. 10.2):
Fin=Fs+Ff
Fout=Ftrab+Fu
Ctot=Ctrab+Cps
At steady state (Eq. 10.3):
F=Fin+Fout
The simplest hydraulic model, represented by the classic Goldmann equation, views aqueous flow as passive nonenergy-dependent bulk fluid movement down a pressure gradient, with aqueous humor leaving the eye only via the trabecular route, where ∆P = P i − P e , so that F = C trab (P i − P e ). This relationship is generally correct, but it is vastly oversimplified. For example, there is good evidence that maintaining outflow resistance is an active process that requires energy such as the maintenance of contractile tone. Wiederholt et al., ($year$) Moreover, because there is no complete endothelial layer covering the anterior surface of the ciliary body and no delimitation of the spaces between the trabecular beams and the spaces between the ciliary muscle bundles , fluid can pass from the chamber angle into the tissue spaces within the ciliary muscle. These spaces in turn open into the suprachoroid, from which fluid can pass through the sclera or the perivascular/perineural scleral spaces into the episcleral tissues. Some fluid is also drawn osmotically into the vortex veins by the high protein content in the blood of these vessels . Along these uveal routes, the fluid mixes with tissue fluid from the ciliary muscle, ciliary processes, and choroid. Thus, this flow pathway may be analogous to lymphatic drainage of tissue fluid in other organs, providing an important means of ridding the eye of potentially toxic tissue metabolites . The eye has long been considered to be devoid of lymphatics, but expression of lymphatic markers are present in the ciliary body and muscle , having apparent lymphatic flow ; however their contribution to aqueous humor drainage is unclear.
Flow from the anterior chamber across the TM into SC is pressure-dependent, but drainage via the unconventional pathway is virtually independent of pressure . The reasons for the pressure-independence of the unconventional pathway are not entirely clear but might be consequent to the complex nature of the pressure and resistance relationships between the various fluid compartments within the soft intraocular tissues along the route . For instance, pressure in the potential suprachoroidal space (Ps) is directly dependent on IOP, such that at any IOP level, Ps is considerably but constantly less than IOP . Because the pressure gradient between the anterior chamber and suprachoroid is independent of IOP, bulk fluid flow between these compartments will also be IOP-independent. Intraorbital pressure is such that under normal circumstances there is always a positive pressure gradient between the suprachoroidal and intraorbital spaces . Fluid and solutes, including large protein molecules, can easily exit the eye by passing through the spaces surrounding the neural or vascular scleral emissaria or through the scleral substance itself . At very low IOP levels, the net pressure gradient across the unconventional pathways is apparently so low that unconventional drainage decreases .
The absence of an outflow gradient from the suprachoroid may contribute to the development of choroidal detachments seen during the ocular hypotony that sometimes follows intraocular surgery . However, other investigators find unconventional outflow to be more pressure-sensitive under certain circumstances . For example, unconventional outflow may become more sensitive to pressure following prostaglandin treatment . Based on calculations of diffusional transport properties, there is no need for a pressure gradient to drive tracer across the sclera because it can diffuse across on its own . Direct evidence for a uveovortex pathway is demonstrated after perfusion of the anterior chamber with fluorescein, and finding the fluorescein concentration in the vortex veins is higher than in the general circulation. Also, flow across the sclera is pressure-dependent . Uveovortex flow explains the relative insensitivity of the flow to pressure because most of the driving force is the colloidal osmotic pressure of the blood that draws the fluid into these vessels . Clearly, more needs to be learned about this flow pathway. As under normal steady-state conditions, C ps is low compared with C trab , the hydraulics of aqueous dynamics may be reasonably approximated for clinical purposes by (Eq. 10.4):
Fin=Fout=Ctrab(Pi−Pe)+Fu
or rearranged (Eq. 10.5):
Pi=Pe(Fin−Fu)/Ctrab
Clinically significant increases in inflow occur only in situations involving breakdown of the blood-aqueous barrier. The pressure sensitivity of the ultrafiltration component of aqueous secretion blunts the tendency for IOP to rise under such conditions, that is, C ps is increased. Elevated episcleral venous pressure, such as may occur with arteriovenous communications resulting from congenital malformations or trauma and perhaps, as shown for the first time in one study, in POAG and normal-tension glaucoma , causes a nearly mmHg for every mmHg increase in IOP, consistent with the Goldmann equation. Clinically relevant reductions in IOP are produced by decreasing F in or by increasing C trab and F u . Pharmacologic agents, particularly vasodilators, also appear to exert a small, but clinically significant effect on P e .
Anatomy of the conventional outflow pathway
Depending upon age, 70% to 90% of the aqueous in humans leaves the eye through the pressure-dependent conventional outflow pathway, which includes the TM, SC, and distal vasculature (aqueous veins, collector channels, and intrascleral venous plexus) . SC and the TM reside within the internal scleral groove spanning the scleral spur to the ring of Schwalbe’s line at the termination of Descemet’s membrane. An anterior nonfiltering portion (also called the “insert” region) presents minimal resistance to fluid outflow and can be distinguished from a posterior filtering portion of the meshwork because it does not overlie SC and houses resident TM stem cells. The TM itself consists of three functionally and structurally different parts: the iridic and uveal part, which represents the innermost portion of the TM; the corneoscleral part, which extends between the scleral spur and the cornea; and the juxtacanalicular tissue (JCT, also called the cribriform layer), which lies adjacent to the inner wall of SC ( Fig. 10.5 ) .

With age, the TM changes from being a long, wedge-shaped tissue to having a shorter, rhomboid shape . The scleral spur appears more prominent in older eyes, with the inner TM being more compact. The trabeculae become progressively thickened and extracellular materials appear more abundant and different in appearance, by transmission electron microscopy. Some of these changes are likely a result of decreased cellularity observed in older eyes. Hence, TM cell loss declines in a linear fashion with age and is more severe in the filtration region . These structural changes correspond to a decline in outflow facility with age .
The JCT is thought to be the major site of hydraulic resistance , the outermost part of the TM consisting of several layers of cells embedded in a ground substance comprising a wide variety of macromolecules, including hyaluronic acid, other glycosaminoglycans, collagen, fibronectin, and other glycoproteins presumably produced by the resident cells . The JCT is supported by an elastic-like fiber network and fine collagen fiber bundles ( Fig. 10.6 ) , extending from the anterior tendons of the longitudinal ciliary muscle bundles, and having the same orientation as the elastic-like network in the central core of the trabecular lamellae. This network is connected on the inner wall endothelium of SC by fine, bent, connecting fibrils. This elastic plexus is far more extensive in the JCT of human eyes than other species, perhaps contributing to the lack of “washout” in human eyes ex vivo . Washout is the phenomenon by which outflow facility increases over time of perfusion with artificial aqueous humor. Interestingly, rodents do not appear to wash out, and also have an extensive cribiform plexus like humans . The washout-associated increase in outflow facility in nonhuman species correlates with the extent of physical separation between the JCT and the inner wall endothelium lining SC or, in some species, the angular aqueous plexus (anatomical equivalent of SC in many nonprimates) .

A contributor to outflow resistance in the JCT appears to be the inner wall itself and its discontinuous basement membrane of the inner wall endothelial cells. Separation of the basal lamina cells lining of the inner wall of SC from the underlying JCT, as seen using quick-freeze/deep-etch electron microscopy, reveals apparent flow pathways . Under normal conditions, the inner wall of SC and JCT cells are likely in a contracted state (having contractile tone), which limits the routes available for fluid flow as demonstrated with gold particle infusion studies in nonhuman primates ( Fig. 10.7 ) . Expansion of the area available for fluid drainage increases the rate of fluid outflow ; although the accompanying loss of extracellular material does not appear to be responsible for the decrease in resistance to fluid outflow .

The inner wall of SC has two primary roles—to maintain the blood-aqueous barrier and to facilitate the movement of aqueous humor out of the eye in the basal to apical direction. To accomplish these apparently opposing tasks, SC cells are a hybrid endothelia, part blood vascular and part lymphatic . Fluid movement across the inner SC wall endothelium is predominantly via pressure-dependent transcellular and paracellular pathways including giant vacuole formation ( Fig. 10.8 ) especially near collector channels . Pore formation is often associated with the giant vacuoles, but may also be found in thin, flat regions in the inner wall . Calculations of the number and size of pores and openings in the inner wall endothelium of SC are too large to account for most of the outflow resistance . These and other findings lead to the hypothesis that the main resistance to aqueous outflow is located internal to the endothelial lining, within the subendothelial tissue or JCT .

Although the pores are large, and likely contribute negligible flow resistance, they force the fluid to “funnel” through confined regions of the cribriform TM tissues nearest the pores ( Fig. 10.7 ). Thus, their number and size can greatly influence the effective outflow resistance of the cribriform tissue . Two studies failed to find a correlation between outflow facility and inner wall pore density as would be expected if the funneling effect contributed to aqueous outflow resistance ; however, other studies report a significant linear relationship between pore density/size and outflow facility . The best evidence of funneling is provided by a perfusion study with gold particles showing exclusion of large segments of the conventional outflow pathway and punctate decoration of SC ( Fig. 10.7 ). Significantly, disruption of funneling by relaxing inner wall cells and JCT cells expands the JCT to increase fluid drainage with rho kinase inhibitors has been exploited therapeutically to lower outflow resistance (described in detail further in the chapter) .
Experimental studies of the transendothelial passage of ferritin particles in monkey eyes indicate that ferritin also traverses tortuous paracellular routes that lie between the endothelial cells of SC . The functional significance of these paracellular routes for aqueous outflow is not known. However, tight junctions between endothelial cells of SC become less complex with increasing pressure, suggesting that the paracellular pathway into SC in the normal eye may be sensitive to modulation within a range of physiologically relevant pressures .
Active involvement of the conventional outflow pathway in IOP homeostasis
Mounting evidence portrays the TM as a living, active, and reactive organ, rather than just a passive mélange of tissue components. Long-term homeostasis in response to physical stress requires modulation by cellular constituents of the conventional outflow pathway, including the cellular lining trabecular lamellae, JCT cells, SC endothelia, and cells of the distal venous vessels. Extracellular load-bearing constituents include the extracellular matrix (ECM) of the trabecular lamellae and the cribiform elastin network, which suspend the TM between two fluid compartments (anterior chamber and SC) at different pressures. The TM likely “senses” this pressure differential by “monitoring” stretch, deformation, shear stress, etc., and strives to maintain these parameters within a homeostatic range. A visual example of the pressure gradient experienced by conventional outflow tissues is provided by the formation of giant vacuoles, where SC cells are extensively stretched into its lumen ( Fig. 10.8 ); or the pressure-dependent narrowing of SC, which as a result increases shear stress experienced by SC endothelial cells to levels approaching arterial vessels . This narrowing of the SC lumen corresponds to an expansion and stretching of the TM and its resident cells . Among other mechanosensors such as ion channels . ATP release mechanisms , and caveoli , these cellular deformations activate integrins , which in turn mediate regulation of ECM deposition and relaxation/contractility of the cells themselves . ECM rigidity in turn modulates cytoskeletal structures, protein expression patterns, signal transduction, and fibronectin deposition in TM cells .
IOP is not stagnant. Overlaid on the mean IOP gradient experienced by cells of the conventional outflow pathway are IOP fluctuations, primarily owing to the filling and draining of the choroid during cycles of the heartbeat, resulting in the ocular pulse . These approximately 3-mmHg fluctuations in IOP cause the TM and inner wall of SC to oscillate ( Fig. 10.9 ). Such TM movements can be visualized via a specialized imaging technique called phase-sensitive optical coherence tomography , and induction of ocular pulse in perfused eyes increases outflow facility . Thus, the TM and inner wall of SC are constantly moving, sensing changes in IOP, and changing outflow resistance, and this movement is depressed in glaucoma , likely due to increased conventional outflow tissue stiffness .

In addition to responding to IOP-induced stretch by altering its contractile tone and ECM, TM cells secrete dozens of soluble mediators . These substances appear to act in both an autocrine and paracrine fashion, some traveling with aqueous flow to impact SC cells downstream. For example, stretching of TM cells triggers the release of vascular endothelial growth factor (VEGF), which increases outflow facility owing to activation of VEGF receptor 2 in SC cells . In contrast, chronic injection of antibodies against VEGF in patients with neovascular age-related macular degeneration results in decreased outflow facility and elevated IOP .
Another paracrine signaling relationship between TM and SC involves the angiopoietin/Tie2 pathway. Here, angiopoetin-1 is expressed by TM cells and its receptor, Tie2 is expressed by SC cells. Thus, conditional knock out of angiopoetin-1 or Tie2 in adult mice results in ocular hypertension . Alternatively, directly activating Tie2 with antibodies or indirectly activating Tie2 by inhibiting its phosphatase decreases IOP by increasing outflow facility . Importantly, this phosphatase inhibitor, AKB-9778 was the first SC-selective drug to be evaluated in human clinical trials for the treatment of ocular hypertension. Although safe and significantly lowering IOP, it unfortunately failed to meet clinical end points.
The oscillatory movements owing to ocular pulse also impact SC by amplifying the shear stress experienced by SC cells during IOP elevations that narrow its lumen . Similar to other blood vessels, shear stress in a narrow SC lumen results in the production of nitric oxide by endothelial nitric oxide synthase (NOS) ( Fig. 10.10 ). Owing to its unique properties as a gas, nitric oxide can diffuse upstream of aqueous humor flow and relax JCT cells or diffuse downstream and change the permeability of SC inner wall or dilate distal veins . The net effect is increased outflow facility to oppose increased IOP. Importantly, the time scale of these homeostatic effects is dynamic and rapid (milliseconds to seconds), and complement the slow homeostasis mediated by ECM turnover, which occurs over hours to days. Emphasizing the role of nitric oxide produced by SC cells in IOP homeostasis, knockout of endothelial NOS results in elevated IOP owing to decreased outflow facility, and transgenic mice overexpressing nitric oxide have lower IOP because of increased outflow facility .

Nitric oxide synthase–immunoreactive nerve fibers are present in the primate TM, especially in the cribriform region adjacent to the inner wall of SC . NOS released from nerve terminals could relax JCT cells and increase outflow facility independent from the ciliary muscle. In human glaucoma eyes there are dramatic reductions in staining indicative of NOS activity in ciliary muscle, TM, and SC, compared with control eyes , that are unrelated to the use of multiple glaucoma therapies, or the severity of the disease . Staining for NOS activity is likely due to nitric oxide produced by all three subtypes of NOS: neuronal NOS in ciliary muscle and TM, inducible NOS from macrophages throughout the conventional outflow pathway, and endothelial NOS in SC. Hence, nitric oxide–mimicking nitrovasodilators, delivered systemically or locally to the eye, decrease IOP by altering outflow resistance, without apparent effects on the ciliary muscle . Drug companies have capitalized on the impact of nitric oxide on outflow physiology by developing several nitric oxide–donating compounds , one of which (latanoprostene bunod) has been approved for use in humans .
Importantly, only 50% to 75% of total outflow resistance resides in the proximal part of the conventional tract in the JCT . The remaining 25% to 50% of resistance is distal to the inner wall of SC, likely in collector channel ostia or contractile intrascleral veins. . Vasoconstriction at these sites likely explains clinical observations whereby surgical bypass or elimination of the JCT region of the conventional outflow pathway only lowers IOP to mid to high teens, instead of near episcleral venous pressure (EVP) (i.e., ~8 mmHg) as predicted by the Goldmann equation . These data suggest that the distal part of the conventional tract is actively responding to removal of upstream resistance. In fact, direct evidence for distal outflow regulation are provided by studies in human and porcine anterior segments where the TM and inner wall of SC have been removed, but outflow facility is altered pharmacologically . Both here in the distal region of the conventional outflow tract and proximally, endothelin-1 physiologically opposes nitric oxide to regulate vascular/trabecular tone and regulate IOP .
Taken together, conventional outflow tissues sense the physical, as well as the biochemical, environment in which they reside, and make modifications to their physical and conformational properties to affect their overall hydraulic conductivity and thereby allow the eye to reach a specific target pressure (discussed further in chapter). Evidence for such a homeostatic response is provided by experiments that artificially increase IOP of eyes during organ culture perfusions. In response to elevated IOP, outflow facility increases steadily over 2 to 3 days until IOP is normalized ( Fig. 10.10 ) . Responses of the TM to long-term increases in IOP can be visualized with tracer, showing that there are alterations in the distribution of preferential flow passageways . This “slow” homeostatic response is likely mediated by increased ECM turnover . Interestingly, homeostatic response to an elevated IOP challenge appears disrupted in glaucomatous eyes ( Fig. 10.10 ) .
Obstruction of outflow
Extracellular matrix accumulation
In glaucomatous eyes there is an increase in ECM beneath the inner wall of SC and in the cribriform region of the TM and thickening of the trabecular lamellae compared with age-matched normal controls . In advanced cases of POAG there is additional loss of TM cells beyond that associated with normal aging . In this condition, the inner uveal and corneoscleral lamellae appear fused together , and SC can be partly obliterated. Areas with more ECM are less perfused, presumably because of the higher resistance of the area . The origin of the increased amounts of ECM in glaucomatous eyes is still unknown. Complicating interpretation of results, most specimens of glaucomatous eyes investigated morphologically are derived from patients who have been treated for many years with antiglaucoma drugs, which can themselves induce changes in the biology of the trabecular cells by slowing or diverting flow of aqueous humor .
Transforming growth factor (TGF) β2, a component of normal aqueous humor in many mammalian eyes , influences ECM production in the TM and has been implicated in IOP elevation . Increased levels of total and active TGFβ2 are found in the aqueous humor of POAG patients compared with age-matched controls . TGFβ2 decreases the activity of matrix metalloproteinases (MMP), and thus possibly contributes to increased ECM in the TM of glaucomatous eyes . Perfusion of human anterior segments ex vivo with TGFβ2 results in decreased outflow facility and increased focal accumulation of ECM under the inner wall of SC . One of these ECM proteins, cochlin, is elevated in the TM upon TGFβ2 treatment of perfused anterior segments and in glaucomatous eyes. Overexpression in cochlin results in decreased outflow facility . In vitro treatment of cultured TM cells with TGFβ2 results in elevated production of ECM proteins such as fibronectin and plasminogen activator inhibitor (PAI-1) . In addition, interactions between bone morphogenic protein (BMP), Wnt proteins, and TGFβ represent potential checkpoints to regulate TM cell function, IOP, and glaucoma pathogenesis. For instance, the expression of secreted frizzled-related protein-1 (sFRP-1) is significantly elevated in the glaucomatous TM. Accordingly, perfusion of human eyes with sFRP-1 significantly decreased outflow facility .
How important “extra” ECM is in increasing resistance to aqueous humor outflow (and thus increasing IOP) in open-angle glaucoma is uncertain. TM cells are highly phagocytic and the normal turnover of ECM is fast, similar to that of a healing wound . This appears to be in part for outflow resistance regulation and in part for maintenance of a patent drainage pathway for aqueous humor. Thus, the TM is in effect a self-cleaning filter, and that in most of the open-angle glaucomas, the self-cleaning (i.e., phagocytic) function is deficient or at least inadequate to cope with the amount of material present . Phagocytosis, especially of particulate matter and red blood cells, is also carried out by resident macrophages of the TM, which are numerous . Macrophages appear important in clearing the anterior chamber of some inflammatory debris after laser trabeculoplasty , or a pigment shower . However, their role in normal housekeeping and bulk outflow of aqueous humor is currently unclear.
Combining the clogged-filter concept of glaucoma and the washout concept of perfusion-induced resistance decrease inevitably led to interest in compounds that might disrupt or remodel the structure of the TM and SC inner wall to enhance flow through the tissue and/or promote washout of normal and pathologic resistance–producing ECM (discussed further in chapter). For example, a rho kinase inhibitor was shown to prevent and reverse ECM accumulation in the JCT due to chronic glucocorticoid treatment . Such compounds will likely provide insights into cellular and extracellular mechanisms governing outflow resistance in normal and glaucomatous states. Additionally, if normal or pathologic ECM required many years to accumulate to the extent that IOP became elevated, perhaps a one-time washout would provide years of normalized outflow resistance and IOP .
Cells and other particulates
Normal erythrocytes are deformable and pass easily from the anterior chamber through the tortuous pathways of the TM and the inner wall of SC . However, nondeformable erythrocytes such as sickled or clastic (ghost) cells may become trapped within and obstruct the TM, elevating outflow resistance and IOP . Similarly, macrophages that are swollen after ingesting lens proteins leaking from a hypermature cataract or breakdown products from intraocular erythrocytes or pigmented tumors (or the tumor cells themselves) may produce JCT obstruction. Pigment liberated from the iris spontaneously (pigmentary dispersion syndrome) or iatrogenically (following laser iridotomy) may clog or otherwise alter the TM function temporarily, presumably without prior ingestion by wandering macrophages , as may zonular fragments following iatrogenic enzymatic zonulolysis or lens capsular fragments following laser posterior capsulotomy . Ocular amyloidosis can lead to elevated IOP as a result of blockage of the TM with amyloid particles .
Glaucoma secondary to hypermature cataract (phacolytic glaucoma) or uveitis has long been ascribed to JCT obstruction. In the former entity, the presence of protein-laden macrophages lining the chamber angle seems adequate to account for increased outflow resistance . The uveitis-related glaucomas comprise many different entities, and the etiology of the increased outflow resistance seems less clear; postulated mechanisms include TM involvement by the primary inflammatory process, JCT obstruction by inflammatory cells, or secondary alteration of TM cellular physiology by inflammatory mediators or byproducts released elsewhere in the eye.
Small amounts of purified high-molecular-weight, soluble lens proteins or serum itself , when perfused through the anterior chamber of freshly enucleated human eyes, causes an acute and marked increase in outflow resistance. Thus, it may be that specific proteins, protein subfragments, or other macromolecules are themselves capable of obstructing or altering the TM to increase outflow resistance, perhaps contributing to the elevated IOP in entities such as the phacolytic, uveitic, exfoliation , and hemolytic glaucomas. In the living eye, serum proteins constantly diffuse out from the iris stroma into the anterior chamber, equilibrating at an approximately 1% concentration and exiting via the trabecular outflow pathway . Experimental perfusion of the enucleated calf eye or monkey eye in vivo with medium containing higher serum protein concentrations than found in normal aqueous humor indeed reduces or eliminates resistance washout. Perhaps protein in the aqueous humor that passes through the TM is essential for maintenance of normal resistance either by providing resistance itself, or by signaling or modifying some property of the TM such as stimulation of focal adhesion and stress fiber formation, to enhance adhesion of TM cells to the ECM . Hyaluronate- and chondroitin sulfate–based agents used as tissue spacers during intraocular surgery (“viscoelastic agents”) may raise IOP in human eyes if not completely removed from the eye by irrigation/aspiration at the conclusion of a procedure, presumably by obstructing trabecular outflow .
Pharmacology and regulation of outflow
Cholinergic effects on conventional outflow
In primates the iris root inserts into the ciliary muscle and the uveal TM just posterior to the scleral spur, while the ciliary muscle inserts at the scleral spur and the posterior inner aspect of the TM . The anterior tendons of the longitudinal portion of the ciliary muscle insert into the outer lamellated portion of the TM and into the JCT, and via elastic elements into specialized cell surface adaptations on the inner wall of SC endothelial cells ( Fig. 10.6 ). Ciliary muscle contraction results, not only in spreading of the lamellated portion of the TM but also in an inward pulling of the cribriform elastic fiber plexus and straightening of the connecting fibrils and dilation of SC . Movement of the inner wall region affects the area and configuration of the outflow pathways and thereby outflow resistance , which in the nonaccommodating rodent eye suggest that the initial function of longitudinal ciliary muscle fibers was to regulate trabecular outflow . Voluntary accommodation (human) , electrical stimulation of the third cranial nerve (cat) , topical, intracameral, or systemically administered cholinergic agonists (monkey and human) , and, in enucleated eyes (monkey and human), pushing the lens posteriorly with a plunger through a corneal fitting all decrease outflow resistance, while ganglionic blocking agents and cholinergic antagonists increase resistance .
Furthermore, the resistance-decreasing effect of intravenous pilocarpine in monkeys is virtually instantaneous, implying that the effect is mediated by an arterially perfused structure or structures . However, not all the experimental evidence supports this strictly mechanical view of cholinergic and anticholinergic effects on TM function. For example, in monkeys, intravenous atropine rapidly reverses some, but not all of the pilocarpine-induced resistance decrease , and topical pilocarpine causes a much greater resistance decrease per diopter of induced accommodation than does systemic pilocarpine (monkey) or voluntary accommodation (human) . The inability of atropine to rapidly and completely reverse the pilocarpine-induced facility increase in normal eyes could be due to mechanical hysteresis of the TM . The variation in the relative magnitude of pilocarpine-induced accommodation and resistance decrease when the drug is administered by different routes might reflect differences in bioavailability of the drug to different regions of the muscle . However, following ciliary muscle disinsertion and total iris removal (but not iris removal alone), there is virtually no acute outflow resistance response to intravenous or intracameral pilocarpine and no response to topical pilocarpine . Thus, it seems certain that the acute resistance-decreasing action of pilocarpine, and presumably other cholinomimetics, is mediated entirely by drug-induced ciliary muscle contraction, with no direct pharmacologic effect on the TM itself.
Cholinergic and nitrenergic nerve terminals that could induce contraction and relaxation of TM and scleral spur cells are present in primate TM and scleral spur. Terminals in contact with the elastic-like network of the TM and containing substance P immunoreactive fibers resemble afferent mechanoreceptor–like terminals . Afferent mechanoreceptors that measure stress or strain in the connective tissue elements of the scleral spur have also been identified . These findings raise the possibility that the TM may have some ability to self-regulate aqueous humor outflow; however, this has not been demonstrated experimentally.
Muscarinic receptors and contractile elements are present in the TM. The m3 muscarinic receptor transcript and m2 receptor protein is detected in human TM of cadaver eyes . Carbachol (CARB)-induced mobilization of Ca 2+ and phosphoinositide production in human TM cells in culture are associated with the M3 muscarinic receptor activation . Smooth muscle–specific contractile proteins are present in cells within the human TM and adjacent to the outer wall of SC and the collector channels . Isolated bovine TM strips contract isometrically in response to CARB, pilocarpine, aceclidine (ACEC), and acetylcholine and endothelin-1 . However, in the organ-cultured perfused bovine anterior segment, endothelin-1 and CARB-induced contractions result in a reduction of the outflow rate . Also, low (10 −8 to 10 −6 M) but not high (10 −4 to 10 −2 M) doses of pilocarpine, ACEC, or CARB induce increased outflow facility in human perfused anterior ocular segments, devoid of ciliary muscle . However, low doses of pilocarpine have no effect on outflow facility in living monkey eyes ; facility increases occur only at doses that also produced miosis and accommodation.
Pilocarpine-induced ciliary muscle contraction spreads the TM, perhaps with the same physiologic consequences as direct relaxation of the TM—decreased tissue density and thus decreased flow resistance due to opening of new flow pathways. Combination of threshold facility–effective doses of pilocarpine with maximal outflow facility–effective doses of H-7 (discussed further in chapter) can further enhance the outflow facility response with minimal effects on accommodation .
At least two different subtypes of muscarinic receptors, M2 and M3, are present in the ciliary muscle . The M2 receptor shows preferential localization to the longitudinal , putatively more facility-relevant portion of the ciliary muscle, but to date no functional role for this subtype has been elucidated. mRNA from the M2, M3, and M5 subtypes is strongly expressed in the longitudinal and circular portions of human ciliary muscle cells and tissue . The M3 subtype appears to mediate the outflow facility and accommodative responses to pilocarpine and aceclidine in monkeys . In monkeys, the outer longitudinal region of the ciliary muscle differs ultrastructurally and histochemically from the inner reticular and circular portions . Although differential distribution of muscarinic subtypes is probably not responsible for the outflow facility/accommodation dissociation occurring under certain conditions, functional dissociation might be produced by combinations of drugs from different classes.
Given the long-term use of cholinergic agonists in glaucoma therapy and the vital role of ciliary muscle tone in regulating outflow resistance, it is important to note that in the monkey, topical administration of the cholinesterase inhibitor echothiophate or the direct-acting agonist pilocarpine desensitizes the outflow facility and accommodative responses to pilocarpine, accompanied by decreased numbers of muscarinic receptors in the ciliary muscle . Even a single dose of pilocarpine or carbachol reduces receptor number . Because cholinergic drug therapy is not the mainstay of glaucoma therapy that it once was, the issues related to refractoriness to long-term therapy versus disease progression are no longer prominent areas of research.
Cholinergic effects on unconventional outflow
When the ciliary muscle contracts in response to exogenous pilocarpine ( Fig. 10.11 ), the spaces between the muscle bundles are substantially obstructed . Conversely, during atropine-induced ciliary muscle relaxation, the spaces are widened . If mock aqueous humor–containing albumin labeled with iodine-125 or iodine-131 (which under resting conditions leave the anterior chamber essentially by bulk flow via the conventional and unconventional drainage routes) is perfused through the anterior chamber, autoradiographs show qualitatively the distribution of the flow . In the pilocarpinized eye, radioactivity is present in the iris stroma, the iris root, the region of SC and the surrounding sclera, and the most anterior portion of the ciliary muscle. In the atropinized eye, radioactivity is found in all these tissues, as well as throughout the entire ciliary muscle and even further posteriorly in the suprachoroid/sclera . In other perfusion experiments quantifying unconventional drainage, pilocarpinized eyes demonstrate but a fraction of the unconventional flow in atropinized eyes . Thus, to generalize in the primate eye, the magnitude of the pilocarpine (and presumably all cholinergic agonists) enhancement of aqueous humor drainage via the conventional route is greater than the reduction of drainage via the unconventional route, thus resulting in a reduction in IOP.

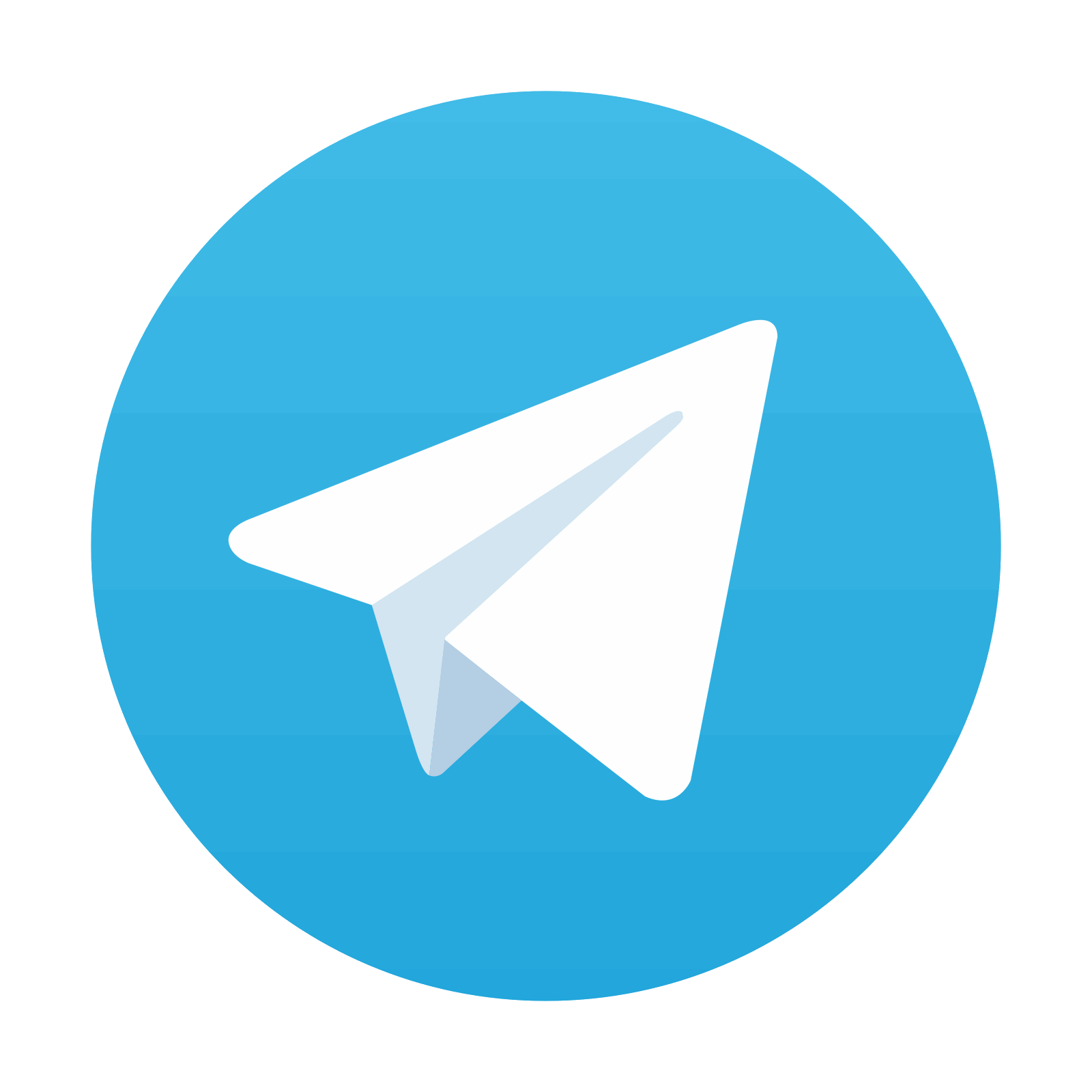
Stay updated, free articles. Join our Telegram channel

Full access? Get Clinical Tree
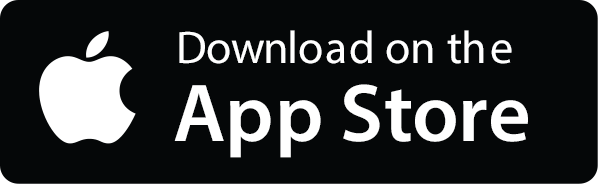
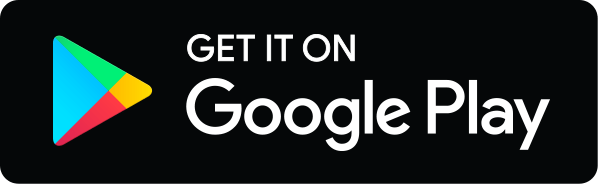
