Purpose
To summarize emerging concepts regarding the onset and progression, traction effects, and complications of the early stages of age-related posterior vitreous detachment (PVD).
Design
Interpretive essay.
Methods
Review and synthesis of selected literature, with clinical illustrations, interpretation, and perspective.
Results
Imaging of the vitreoretinal interface with optical coherence tomography has shown that PVD begins in the perifoveal macula. Recent longitudinal studies have demonstrated conclusively that early PVD stages persist chronically and progress slowly over months to years. Vitreous traction forces resulting from perifoveal PVD with a small vitreofoveolar adhesion (500 μm or less) may cause localized cystoid foveal thickening or one of several macular hole conditions. Traction associated with larger adhesion zones may cause or exacerbate a separate group of macular disorders. Ultrastructural studies suggest that epiretinal membrane develops from cortical vitreous remnants left on the retinal surface after PVD and plays an important role in traction vitreomaculopathies.
Conclusions
Age-related PVD is an insidious, chronic event that begins in the perifoveal macula and evolves over a prolonged period before vitreopapillary separation. Although asymptomatic in most individuals, its early stages may be complicated by a variety of macular and optic disc pathologic features, determined in part by the size and strength of the residual vitreoretinal adhesion.
Posterior vitreous detachment (PVD) is the most important event in the life of the human vitreous gel. Defined as a separation between the posterior vitreous cortex and the internal limiting membrane (ILM) of the retina, PVD is the culmination of aging changes in the vitreous and may induce a variety of pathologic events at the vitreoretinal interface. It has long been held that age-related PVD occurs as an acute event, precipitated by the abrupt development of a break in the thin posterior cortical vitreous layer overlying the macular region. This is thought to result in the sudden passage of liquefied vitreous into the subhyaloid space, causing a rapid separation of the vitreous cortex from the retina, beginning posteriorly and progressing peripherally to the vitreous base. In patients without retinal vascular disease, states of partial PVD are believed to progress rapidly to complete PVD.
The complications of age-related PVD conventionally have been regarded as those that develop subsequent to vitreopapillary separation. The most common are retinal tear, vitreous hemorrhage, rhegmatogenous retinal detachment, and retinal or optic disc hemorrhage. These complications are caused by dynamic vitreous traction on focal areas of firm vitreoretinal adhesion; the traction results from large movements of vitreous gel during ocular saccades. Large excursions of the vitreous gel during ocular rotations become possible only after release of the tether formed by the firm vitreous adhesion to the peripapillary retina.
Although the culmination of age-related PVD at the time of vitreopapillary separation occurs in many patients as an acute symptomatic event, there is substantial evidence that this terminal event is preceded by earlier stages that usually are chronic, occult, and asymptomatic. This article discusses evidence that age-related PVD begins as a shallow, localized separation of the vitreous from the perifoveal retina and progresses slowly over months or years until its completion at the time of vitreopapillary separation. In most individuals, the early stages of PVD are asymptomatic and clinically undetected until separation from the optic disc margin produces symptoms and signs of a Weiss ring. In a subset of patients, however, the traction effects of early PVD stages cause or exacerbate a variety of macular and optic disc pathologic features, determined in part by the size and strength of the residual vitreoretinal adhesion. Understanding the evolution and complications of the early stages of PVD increasingly is relevant in light of ongoing research in enzymatic methods for inducing PVD.
Aging Changes Leading to Posterior Vitreous Detachment
A detailed description of vitreous composition and biochemistry, vitreoretinal interface anatomic features and adhesion, and pathophysiologic characteristics of age-related vitreous liquefaction and syneresis is beyond the scope of this perspective article and has been reviewed recently elsewhere. Briefly summarized, aging of the human vitreous is characterized by gel liquefaction and the development of fluid-filled pockets, typically beginning in front of the macula and in the central vitreous cavity. Liquid vitreous appears in the human eye usually around the age of 4 years and involves approximately one fifth of the total vitreous volume by the middle to late teenage years. With advancing age, the liquefied lacunae increase in number, size, and coalescence, with liquefaction of at least 50% of the gel in most individuals older than 70 years. Vitreous liquefaction is accompanied by aggregation of fine collagen fibrils into bundles of parallel fibrils and fibers that are visible biomicroscopically.
In addition to vitreous liquefaction, the second critical factor in the pathogenesis of PVD is progressive age-related weakening of the adhesion between the posterior vitreous cortex and the ILM. Vitreous liquefaction alone is not sufficient to precipitate complete PVD, because autopsy studies have shown that among patients younger than 60 years, the vitreous remains attached to the retina in most eyes with extensive liquefaction. Past the age of 60, however, there is a strong relationship between the degree of liquefaction and prevalence of PVD. Therefore, instability of the vitreous gel accompanying advanced vitreous liquefaction usually does not result in age-related PVD until the vitreoretinal adhesion is sufficiently weakened.
Age-related PVD ultimately results, then, from progressive vitreous liquefaction in conjunction with progressive weakening of the vitreoretinal adhesion. As the premacular liquefied vitreous pocket enlarges, its posterior wall, the vitreous cortical layer overlying the macular area, becomes extremely thin ( Figure 1 ). Gradual dissolution and weakening of the adhesion between the vitreous cortex and the ILM in the posterior pole allows liquid vitreous to enter the retrohyaloid space, possibly through the prepapillary hole in the vitreous cortex or a microbreak in the thin cortical vitreous layer in the perifoveal area. Rotational eye movements likely contribute to the subsequent dissection of a plane between the vitreous cortex and the ILM, leading to progressive PVD. When PVD occurs, typically there is complete separation of all vitreous material from the retina, although splitting of the posterior vitreous cortex (vitreoschisis) occurs in a large subset of eyes, leaving remnants of cortical vitreous attached to the ILM in areas of firm vitreoretinal adhesion.
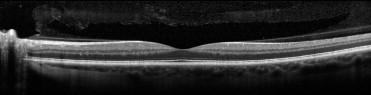
Complications of PVD are more likely to occur in eyes where accelerated vitreous liquefaction occurs before adequate weakening of the vitreoretinal adhesion, a phenomenon that has been called anomalous PVD . Vitreous liquefaction and PVD occur at a younger age in myopic compared with hyperopic or emmetropic eyes. Similarly, premature vitreous liquefaction and syneresis occur in hereditary vitreoretinal syndromes involving type II collagen metabolism, such as Stickler and Marfan syndromes. Other factors that accelerate vitreous liquefaction and PVD include retinal vascular diseases, trauma, aphakia, inflammation, and vitreous hemorrhage. Finally, reduced hyaluronic acid synthesis associated with declining estrogen levels in postmenopausal women may promote vitreous liquefaction, possibly explaining the higher incidence and younger age of onset of PVD in women.
Evolution of the Early Stages of Posterior Vitreous Detachment
The belief that age-related PVD occurs as a sudden event is based on the fact that patients with symptoms of complete PVD describe the acute onset of photopsias, floaters, or both. Historically, the early stages of shallow, partial PVD that precede vitreopapillary separation were difficult or impossible to detect with biomicroscopy or ultrasound. More recently, B-scan ultrasonography performed with meticulous attention to perpendicularity has proven capable of detecting shallow posterior hyaloid detachments. More importantly, optical coherence tomography (OCT) has, over the past decade, provided substantial new capacity for high-resolution cross-sectional imaging of vitreoretinal relationships.
Because of the inability to identify and image its early, asymptomatic stages, the pattern and rate of PVD evolution until recently have been impossible to determine. Investigators long have suspected that age-related PVD begins in the macular region, possibly related to the premacular liquefied vitreous pocket universally found in older eyes ( Figure 1 ). In 1999, the initial stages of PVD were demonstrated by OCT in fellow eyes of macular hole patients, beginning in the macular periphery, then spreading gradually throughout the entire perifoveal area while remaining attached at the foveola ( Figure 2 ). Subsequently, the early stages of PVD were imaged with OCT in a large prospective study of healthy, asymptomatic middle-aged individuals. Most of these subjects were found to have varying degrees of localized vitreous detachment from the perifoveal retina with persistent attachment to the fovea, optic disc, and midperipheral retina. Partial PVD involving the entire macula and complete PVD were found in a smaller subset of eyes.
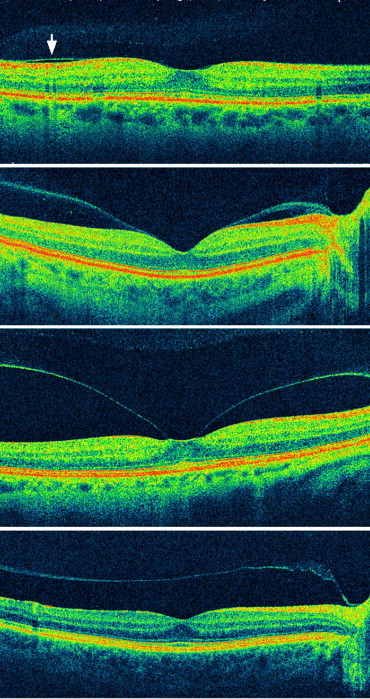
The fact that age-related PVD begins in the perifoveal region is not surprising in light of known topographic variations in strength of the vitreoretinal adhesion. The vitreous is attached to the retina most firmly in areas where the ILM is thinnest, including the vitreous base, along large retinal vessels, the optic disc margin, and the 500-μm diameter foveola. A landmark scanning electron microscopic study of autopsy eyes with spontaneous PVD found remnants of vitreous cortex in the foveal area in 44% of eyes. Most remnants had the configuration of a 500-μm diameter plaque of vitreous adherent to the foveola. In some eyes, a 1500-μm diameter ring of vitreous remnant was adherent to the foveal margin. These observations and similar observations during intraoperative induction of PVD strongly suggest that the central 500-μm foveolar area and the margin of the 1500-μm fovea are sites of firm vitreoretinal adhesion.
The pattern of evolution of early-stage PVD can be studied with 3-dimensional maps constructed with OCT imaging. A recent study using OCT mapping in eyes with idiopathic macular hole suggests that PVD begins in the perifoveal macula, extends next into the superior and temporal midperiphery (possibly because of gravitational effects), and then into the fovea, the inferior midperiphery, and finally the optic disc margin, resulting in complete PVD. The preterminal stage, in which there is complete PVD except for vitreopapillary attachment, typically is asymptomatic and therefore is recognized uncommonly. However, this configuration sometimes is noted on ultrasound or biomicroscopic examination and occasionally causes optic disc hemorrhages or visual symptoms. The observations cited above suggest the following staging scheme for age-related PVD ( Figure 3 ): stage 0, absence of PVD; stage 1, perifoveal PVD with vitreofoveal adhesion; stage 2, macular PVD (no vitreofoveal adhesion); stage 3, near-complete PVD with vitreopapillary adhesion only; and stage 4, complete PVD.
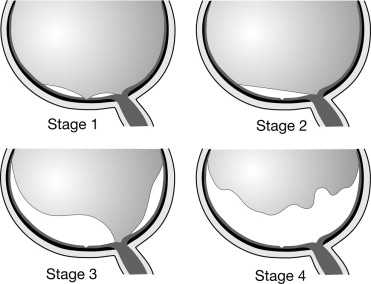
Several cross-sectional studies imply that early PVD stages in healthy eyes and in eyes with idiopathic macular hole and diabetic macular edema (DME) are chronic and slowly progressive. More recently, several longitudinal studies conclusively have demonstrated that early PVD stages persist chronically and progress to complete PVD slowly over months to years. In a study of eyes with various vitreomacular disorders followed up longitudinally, only 10% of 31 eyes with stage 1 or 2 PVD progressed to complete PVD over an average of 30 months. Likewise, in a study of fellow eyes of patients with unilateral macular hole, 58 eyes with stage 1 PVD were followed up by OCT for 24 months. During this period, progression to stage 2 PVD occurred in 29%, and progression to complete PVD was observed in only 12% of eyes. Similar slow rates of progression of early-stage PVD were seen in longitudinal studies of macular pseudo-operculum and unoperated idiopathic macular hole.
Evolution of the Early Stages of Posterior Vitreous Detachment
The belief that age-related PVD occurs as a sudden event is based on the fact that patients with symptoms of complete PVD describe the acute onset of photopsias, floaters, or both. Historically, the early stages of shallow, partial PVD that precede vitreopapillary separation were difficult or impossible to detect with biomicroscopy or ultrasound. More recently, B-scan ultrasonography performed with meticulous attention to perpendicularity has proven capable of detecting shallow posterior hyaloid detachments. More importantly, optical coherence tomography (OCT) has, over the past decade, provided substantial new capacity for high-resolution cross-sectional imaging of vitreoretinal relationships.
Because of the inability to identify and image its early, asymptomatic stages, the pattern and rate of PVD evolution until recently have been impossible to determine. Investigators long have suspected that age-related PVD begins in the macular region, possibly related to the premacular liquefied vitreous pocket universally found in older eyes ( Figure 1 ). In 1999, the initial stages of PVD were demonstrated by OCT in fellow eyes of macular hole patients, beginning in the macular periphery, then spreading gradually throughout the entire perifoveal area while remaining attached at the foveola ( Figure 2 ). Subsequently, the early stages of PVD were imaged with OCT in a large prospective study of healthy, asymptomatic middle-aged individuals. Most of these subjects were found to have varying degrees of localized vitreous detachment from the perifoveal retina with persistent attachment to the fovea, optic disc, and midperipheral retina. Partial PVD involving the entire macula and complete PVD were found in a smaller subset of eyes.
The fact that age-related PVD begins in the perifoveal region is not surprising in light of known topographic variations in strength of the vitreoretinal adhesion. The vitreous is attached to the retina most firmly in areas where the ILM is thinnest, including the vitreous base, along large retinal vessels, the optic disc margin, and the 500-μm diameter foveola. A landmark scanning electron microscopic study of autopsy eyes with spontaneous PVD found remnants of vitreous cortex in the foveal area in 44% of eyes. Most remnants had the configuration of a 500-μm diameter plaque of vitreous adherent to the foveola. In some eyes, a 1500-μm diameter ring of vitreous remnant was adherent to the foveal margin. These observations and similar observations during intraoperative induction of PVD strongly suggest that the central 500-μm foveolar area and the margin of the 1500-μm fovea are sites of firm vitreoretinal adhesion.
The pattern of evolution of early-stage PVD can be studied with 3-dimensional maps constructed with OCT imaging. A recent study using OCT mapping in eyes with idiopathic macular hole suggests that PVD begins in the perifoveal macula, extends next into the superior and temporal midperiphery (possibly because of gravitational effects), and then into the fovea, the inferior midperiphery, and finally the optic disc margin, resulting in complete PVD. The preterminal stage, in which there is complete PVD except for vitreopapillary attachment, typically is asymptomatic and therefore is recognized uncommonly. However, this configuration sometimes is noted on ultrasound or biomicroscopic examination and occasionally causes optic disc hemorrhages or visual symptoms. The observations cited above suggest the following staging scheme for age-related PVD ( Figure 3 ): stage 0, absence of PVD; stage 1, perifoveal PVD with vitreofoveal adhesion; stage 2, macular PVD (no vitreofoveal adhesion); stage 3, near-complete PVD with vitreopapillary adhesion only; and stage 4, complete PVD.
Several cross-sectional studies imply that early PVD stages in healthy eyes and in eyes with idiopathic macular hole and diabetic macular edema (DME) are chronic and slowly progressive. More recently, several longitudinal studies conclusively have demonstrated that early PVD stages persist chronically and progress to complete PVD slowly over months to years. In a study of eyes with various vitreomacular disorders followed up longitudinally, only 10% of 31 eyes with stage 1 or 2 PVD progressed to complete PVD over an average of 30 months. Likewise, in a study of fellow eyes of patients with unilateral macular hole, 58 eyes with stage 1 PVD were followed up by OCT for 24 months. During this period, progression to stage 2 PVD occurred in 29%, and progression to complete PVD was observed in only 12% of eyes. Similar slow rates of progression of early-stage PVD were seen in longitudinal studies of macular pseudo-operculum and unoperated idiopathic macular hole.
Tractional Effects of Perifoveal Posterior Vitreous Detachment
Separation of the posterior hyaloid from the perifoveal retina likely exerts traction on the foveola by at least 2 mechanisms. First, the elastic properties of a trampoline-like posterior hyaloid detachment with focal adhesion to the foveola exerts traction on the foveola anteriorly toward the plane of the detachment. Because the 3-dimensional plane of posterior hyaloid detachment is anterior to the plane of the inner retinal surface, cross-sectional images (such as OCT and ultrasound) show the hyaloid to have a biconvex configuration where it is tethered posteriorly at the foveola. This static anterior traction associated with stage 1 PVD often pulls the inner portion of the fovea above the plane of the surrounding macula, as seen in OCT images of early macular hole states, vitreofoveolar traction syndrome, and traction DME.
Second, stage 1 (perifoveal) PVD localizes to the fovea the dynamic vitreous traction forces generated during ocular rotations. This is similar to peripheral retinal break formation, in which dynamic vitreous traction develops at a focal vitreoretinal adhesion after vitreous detachment from the surrounding retina. Although both static and dynamic traction forces contribute to macular pathologic features, dynamic forces likely are more important, especially in eyes without an abnormally thickened or taut posterior hyaloid.
It is plausible that the anatomic variation placing certain eyes at risk for macular complications of stage 1 PVD is an especially strong vitreofoveal adhesion. This delays or prevents the smooth and complete vitreofoveal separation that normally occurs during the slow evolution of uncomplicated age-related PVD, allowing the static and dynamic traction forces associated with perifoveal hyaloid detachment to act on the fovea over time. The specific macular disorder that develops in a given eye depends in part on the size of the persistent vitreomacular adhesion. In eyes with perifoveal PVD, a statistically significant correlation has been found between smaller diameters of vitreous attachment and the amount of foveal deformation observed. This follows from the prediction that the tractional stress (force per unit area) acting on the fovea should increase with decreasing area of vitreous attachment.
Complications of Early Posterior Vitreous Detachment Stages
There is growing evidence that a list of the complications of age-related PVD should comprise at least those disorders included in Table 1 . Complications of early PVD stages occur in the posterior pole and usually begin insidiously, whereas complications of complete (stage 4) PVD occur in the retinal periphery and typically present with acute symptoms and signs. Because the complications of late-stage PVD have been reviewed and discussed extensively in previous literature, this article focuses on those disorders that are caused or exacerbated by the early stages of PVD (stages 1–3).
Early-stage PVD |
Epiretinal membrane |
Macular microhole |
Foveal red spot |
Idiopathic macular hole |
Pseudo-operculum |
Inner lamellar macular hole |
Vitreofoveolar traction (traction cystoid macular edema) |
Vitreomacular traction syndrome |
Traction diabetic macular edema |
Myopic traction maculopathy |
Neovascular age-related macular degeneration |
Vitreopapillary traction syndrome |
Late-stage (complete) PVD |
Retinal or optic disc hemorrhage |
Vitreous hemorrhage |
Retinal tear |
Rhegmatogenous retinal detachment |
It is appealing from a pathoanatomic perspective to group the macular complications of perifoveal PVD according to the size of the vitreomacular adhesion zone ( Table 2 ). Small vitreous adhesions (500 μm or less), by imparting greater tractional stress to the foveola, typically result in one of several macular hole conditions or in localized cystoid foveal thickening. The lower tractional stress associated with larger vitreomacular adhesions (typically 1500 μm or more) is less likely to cause a macular dehiscence and is more likely to result in diffuse macular thickening, traction macular detachment, or possibly exacerbation of conditions such as age-related macular degeneration (AMD). Among eyes with a primary diagnosis of epiretinal membrane (ERM), there is significant variability in the size, number, and presence or absence of vitreomacular attachments.
Variable vitreomacular adhesion |
Epiretinal membrane |
Adhesion size ≤ 500 μm |
Macular microhole |
Foveal red spot |
Idiopathic macular hole |
Pseudo-operculum |
Inner lamellar macular hole |
Vitreofoveolar traction (traction cystoid macular edema) |
Adhesion size ± 1500 μm |
Vitreomacular traction syndrome |
Traction diabetic macular edema |
Myopic traction maculopathy |
Neovascular age-related macular degeneration |
Epiretinal Membrane
PVD is believed to play a critical role in the pathogenesis of idiopathic ERM through 1 of 2 mechanisms. First, transient vitreoretinal traction during the development of PVD may cause dehiscences in the ILM through which glial cells can migrate and proliferate on the inner retinal surface. Alternatively, and perhaps more likely, ERMs may result from the proliferation and transdifferentiation of hyalocytes contained within vitreous cortical remnants left on the retinal surface after PVD. Data from recent electron microscopic studies suggest that such vitreous remnants, formed by a splitting or schisis within the vitreous cortex, strongly may promote subsequent fibrocellular proliferation.
In large clinical studies, partial or complete PVD has been found in 80% to 95% of eyes with idiopathic ERM. Several authors have suggested that at least 10% to 25% of idiopathic ERMs develop in eyes with no PVD. However, a clear explanation of the pathogenesis of ERM in eyes with absence of PVD has never been offered. In a recent prospective study of eyes with idiopathic ERM, 27 (100%) of 27 eyes were found by OCT, ultrasound evaluation, or both to have complete or partial PVD. Similarly, a 3-dimensional evaluation of ERM using spectral-domain OCT found complete or partial PVD in 35 (97%) of 36 eyes. It therefore is likely that the association between idiopathic ERM and PVD is universal, and that eyes previously reported as having no PVD actually had early stages of PVD that could not be discerned clinically.
Recent OCT studies demonstrate that the PVD associated with ERM is an early-stage PVD in 14% to 52% of eyes. In such eyes, the ERM is located on the retinal surface in areas underlying detached posterior hyaloid ( Figure 4 ). The observation that ERM often develops during the early stages of age-related PVD may explain some of the peculiar clinical features of ERMs. For example, macular pseudohole may represent a central defect in the ERM in the area of vitreofoveolar adhesion during stage 1 PVD. Similarly, foveal prolapse through a central defect in an ERM is an uncommon clinical finding that may be explained in part by vitreofoveal traction associated with stage 1 PVD.
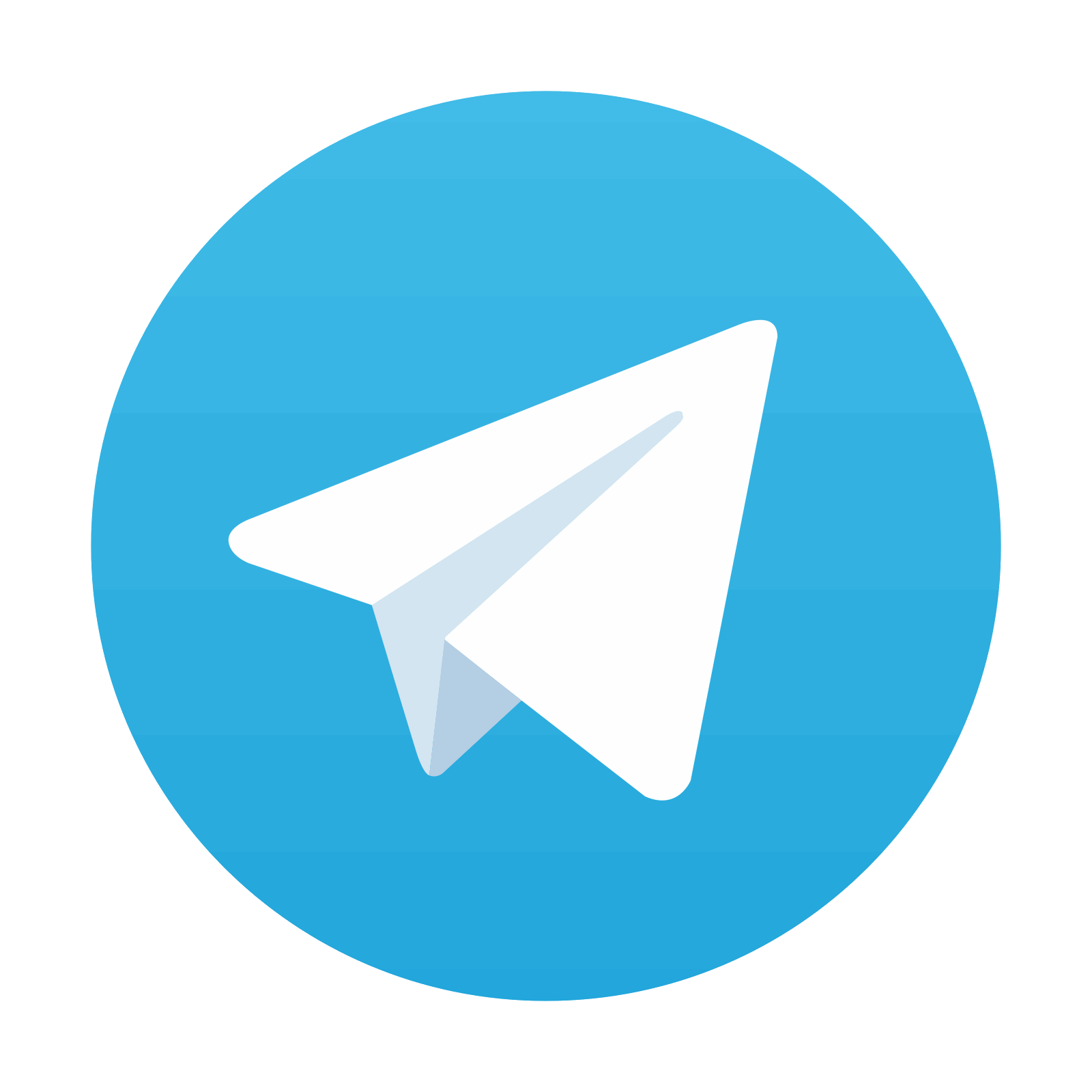
Stay updated, free articles. Join our Telegram channel

Full access? Get Clinical Tree
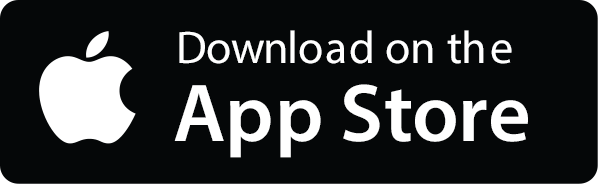
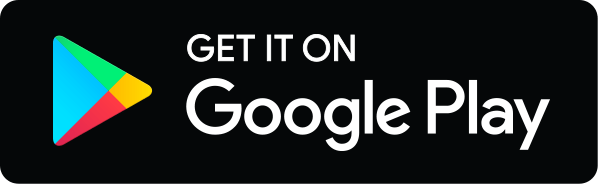
