Physiology of the Facial Nerve
The facial nerve is the motor nerve of the face, scalp, auricle, and neck via the platysma. Mastication and swallowing are in part driven by facial nerve innervation of the posterior belly of the digastric and the stylohyoid muscles. The facial nerve is also the sensory supply to the external auditory canal and the taste buds of the anterior two-thirds of the tongue, and it provides parasympathetic contributions to the submandibular, sublingual, and intralingual salivary glands. Involuntary mimetic function and involuntary motor reflexes are also mediated by the facial nerve; these include the corneal reflex, blink reflex, stapedial reflex, and the oculomentalis reflex. The latter is a contraction of obicularis oculi and mentalis in relation to loud sound or sudden bright light.
Structural Units
Normal motor function of the muscles of facial expression, which is the focus of this discussion, relies on the integrity of the facial nerve. The nucleus of the facial nerve resides in the pons. Seven thousand neuron cell bodies send axons from the pons to the muscles of facial expression. A single neuron can innervate up to 25 muscle fibers.
Each neuron has an axon coated in myelin produced by Schwann cells. Transmission along neurons is by saltatory conduction: depolarization goes from node of Ranvier to node of Ranvier, which are gaps in myelin ( Fig. 2.1a, b ). In the normal physiological state, this saltatory transduction increases the firing rate of the nerve.
Each nerve fiber is surrounded by connective tissue, the endoneurium. The endoneurium is closely adherent to the Schwann cells. Highlighting the structural relevance to normal physiological function, Schwann cells serve as a conduit for regenerating nerve fibers following injury. The perineurium is a sheath of concentric layers of polygonal cells surrounding groups of endoneurium-covered neurons. The perineurium provides tensile strength and intrafunicular pressure. At the outermost layer, the epineurium, loose areolar tissue, holds and separates nerve fasicles. The epineurium contains the vasa nervorum and lymphatic vessels, which provide nutrition to the nerve fibers.
Axons
Axons are the basic structural unit, and axon function relies on:
An ion concentration–mediated negative resting potential. Activation of the cell membrane reverses this potential, generating the action potential. The propagation of the action potential by reversal of the ion gradient is referred to as depolarization.
Each neuron is segmentally insulated by myelin. Where myelin is absent, transmission occurs by a saltatory conduction to the node of Ranvier, propagated along the unmyelinated axon where there is increased cell membrane permeability. Myelination of the nerve allows depolarization to jump from one node of Ranvier to the next, thereby increasing the speed of conduction.
A sufficient stimulus is needed to generate an action potential. Following the action potential, there are refractory and absolute refractory periods during which only large or no stimuli can elicit an action potential.
The action potential is transmitted to the muscle. Each nerve fiber invaginates into the muscle fiber with terminating branches known as sole feet. The space between the sole feet and the muscle fiber membrane is known as the synaptic cleft. Transmission through the synaptic cleft is mediated by acetylcholine. The acetylcholine increases the permeability to sodium ions, changing the resting potential leading to the generation of the action potential.1
Injuries of the Facial Nerve
Anatomic injury of the facial nerve negatively affects normal function. In general, the purview of the clinician treating facial nerve disorders centers on pathology that negatively affect the structural integrity of the nerve and therefore its normal physiological function. Sunderland has described patterns of facial nerve injury based on histological findings ( Fig. 2.2a–f ).2 First-degree nerve injury is a neuropraxia: an intraneural compression that prevents conduction of the impulse. First-degree injuries present with sensory-motor problems distal to the site of injury. The endoneurium, perineurium, and epineurium are all intact, and there is no wallerian degeneration. Conduction is intact in the proximal and distal segment of the nerve, but no conduction occurs across the area of injury. If only some fibers are involved, the nerve is still intact and able to transmit signals, but its ability to function normally is reduced. This is typically seen with partial facial nerve paralysis. This type of injury usually does not require surgical intervention, and the nerve will return to normal function within hours to weeks. It is the mildest type of peripheral nerve injury.

Second-degree injury is a compression that results in loss of axons also known as axonotmesis. The axon degenerates distal to the point of injury in a process known as wallerian degeneration. In second-degree injury, the surrounding Schwann cells maintain their integrity. The expectation is complete recovery without synkinesis as the endoneural tubes, and Schwann cells are presumed intact and help guide regenerating nerve fibers. Electromyography demonstrates fibrillation potentials and positive sharp waves (2 to 3 weeks postinjury). Axonal regeneration occurs, and recovery usually returns to normal without surgical intervention. Occasionally, scar tissue may form and is thought to be responsible for incomplete recovery.
Third-degree injury is a neurotmesis. Endoneural tubules are disrupted secondary to neural compression. As the endoneurium can no longer guide axons, regeneration is incomplete. Axon regeneration will be appropriate and inappropriate. Axons that regenerate and make contact with fibers other than their original endpoint result in synkinetic patterns of facial movement.
First-, second-, and third-degree injuries are mediated by compression. Fourth-degree injury is a disruption of the perineurium, and fifth-degree injury is complete disruption of the nerve. Without intervention, recovery is not expected with fourth- and fifth-degree injury patterns.
Effects of Injury and Mechanisms of Repair
The repair of facial nerve injuries is mediated by a complex array of molecular changes, many that at present are only understood at the gross cellular level. Immune function, a patient′s nutritional status, and the degree of neural disruption all affect the ultimate outcome of recovery. It follows that appropriate return of function will be more variable with a greater degree of injury.
Following injury, there is an increase in cell body volume. This physiologically coincides with chromatolysis, loss of Nissl staining ribosomes and rough endoplasmic reticulum, and increases in ribonucleic acid, enzyme production, and distribution.3,4 There is an increase in calcitonin-related peptide expression, GAP43, and cytoskeletal proteins such as actin and tubulin.5 Proximally, the severed axon begins forming a growth cone.1,3 At 3 days post injury, sprouting begins at the growth cone. Growth occurs at ∼1 mm/day; the facial nerve should therefore be able to grow from the cerebellopontine angle to the periphery within 6 months.6 This activity is usually complete within 3.5 to 4 months.

Favorable and unfavorable factors coincide with this regeneration: axons shrink, allowing new ones to grow around, and microglial cells proliferate, which can interfere with normal synaptic transmission. Sprouting axons maintain a responsiveness to trophic factors. Several neurotrophic factors are known to play a role in regeneration; for a more extensive review, the reader is referred to Tuszynski.7
Regenerating axons travel through Bunger bands formed by Schwann cells that have lined the degenerating axons during wallerian degeneration; the Bunger bands serve as an endoneural tube. The basement membrane of the endoneurium helps to guide regeneration. Regenerating fibers have no known specificity during regeneration; however, the Schwann cell will ultimately myelinate them.8
Endoneural tubules are replaced by connective tissue within 3 to 4 months so that the axons that have not found a target will degenerate and axons that are too far apart to form contact may form a neuroma. Nerve repair is therefore advocated as early as the injury occurs. Distal to the site of injury, nerve resorption with Schwann cell proliferation begins quickly and is very advanced by 48 hours. This process is termed axolysis and myelinolysis, and occurs distal to the site of injury.
Denervation at the muscle results in loss of muscle weight with a decrease in muscle fiber diameter. Early changes in the muscle occur at the cellular level within a week of denervation. These cellular changes include increased mitochondria number, deoxyribonucleic acid, satellite cells, and chromatin change. This represents the plastic state of denervated muscle. Over time, fibers disappear and are replaced by fat and connective tissue. The speed of contraction decreases, and spontaneous action potential known as fibrillations can be recorded. The number of motor end plates increase, and inhibitor substances decrease.9
Facial muscles can be reinnervated by contralateral axons after injury, especially muscles close to midline structures. Prior to reinnervation, muscle viability is tested by volitional movement which if absent is tested by electromyography. If electromyography shows no response, a muscle biopsy can be performed.
The time at which muscle is no longer available to accept reinnervation is not well established. The literature includes case reports of reinnervation occurring after several years, but a “sooner the better” philosophy is advocated by the time course of the described degenerative and regenerative changes observed.
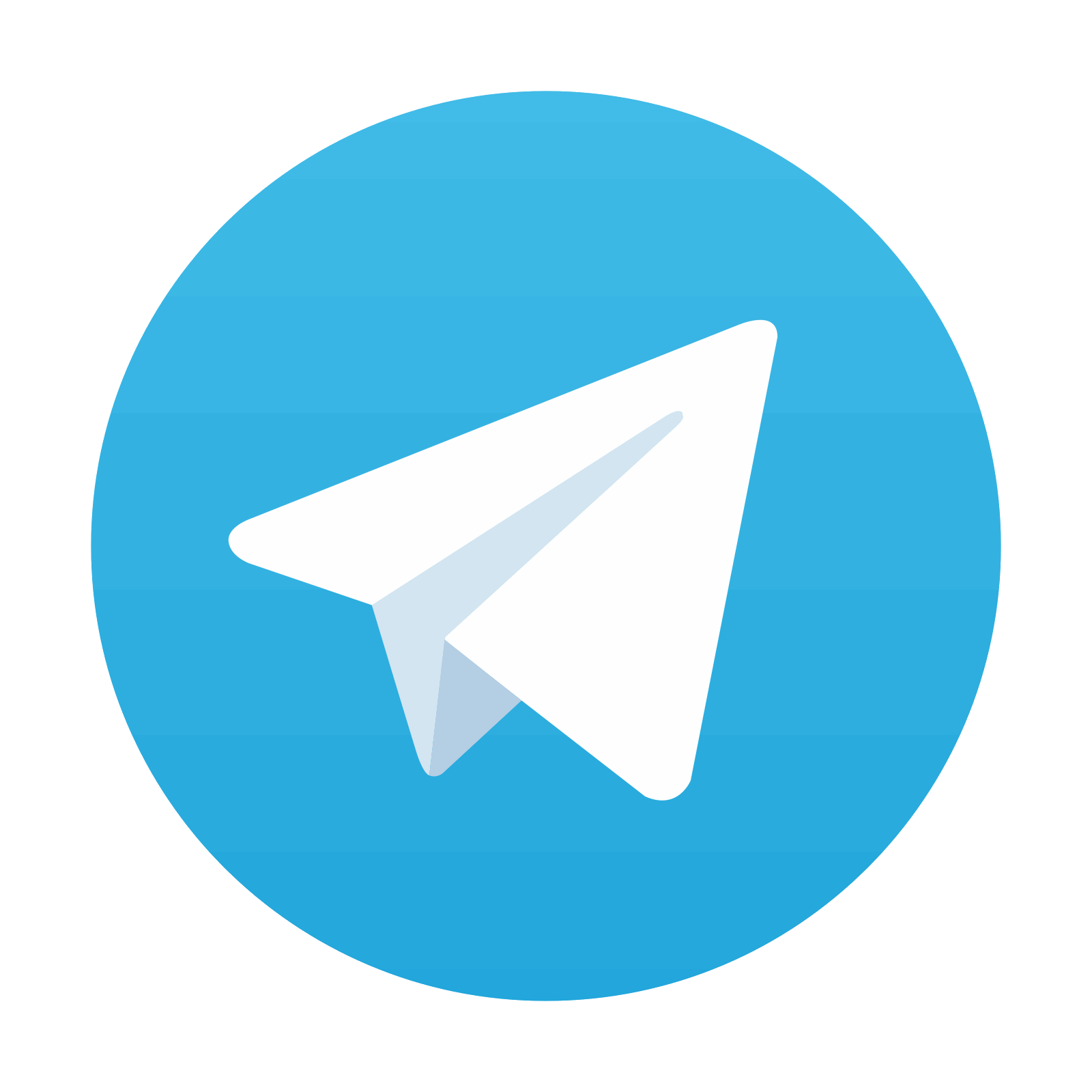
Stay updated, free articles. Join our Telegram channel

Full access? Get Clinical Tree
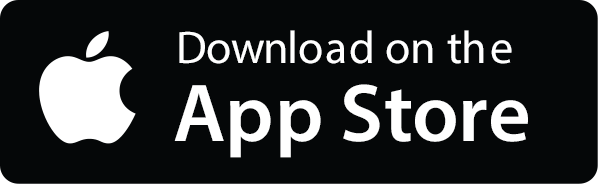
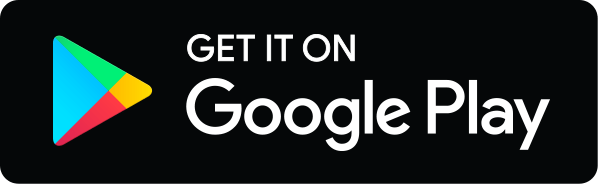
