KEY CONCEPTS
- •
Keratoconus is described as a “multifactorial” condition, implying that many factors contribute to its causation, structural alterations, and progression.
- •
The most important etiological association in keratoconus is believed to be eye rubbing; pressure on the eye, as seen with floppy eyelid syndrome and some lid and orbital tumors, is also a factor in the etiology of keratoconus.
- •
Atopic conditions are associated with 30% to 50% of cases of keratoconus and include vernal keratoconjunctivitis, atopic keratoconjunctivitis, atopic dermatitis (eczema), hay fever, and asthma.
- •
Connective tissue disorders and several syndromes with gene disorders are also important associations of keratoconus.
- •
Elastin degradation in the pre-Descemet (Dua’s) layer has recently been recognized in the pathophysiology of keratoconus.
Introduction
Most diseases fall into one of these categories: degeneration, dystrophy, inflammation, trauma, or neoplasia. The pathogenesis of keratoconus (KC), the commonest ectatic disorder of the cornea, involves all of these except neoplasia. It is described as a “multifactorial” condition, implying that many factors contribute to its causation, structural alterations, and progression, some better understood than others. Despite a plethora of alterations and associations identified with KC, the cause-and-effect relationship remains unclear. Every structure of the cornea, from epithelium to endothelium, is affected at some point during the natural course of the disease.
In this chapter the etiopathophysiology of KC will be considered under the subheadings of causation and associations; clinical pathology, structural and histopathology, and the clinic-pathological correlation; biochemistry; and biomechanics. The evidence will then be synthesized to provide an understanding of the pathogenesis of manifest KC.
Causation and Associations
EYE RUBBING OR OCULAR MASSAGE
The single most important etiological association in KC is believed to be eye rubbing, which can be a factor in up to two-thirds of cases. Several studies have confirmed this association and the fact that other associations like allergic disease and Down syndrome in turn have eye rubbing as a clinical feature. Contact lens (CL) wear is also a form of “eye rubbing” and wearers have a greater incidence of KC.
Analogous to eye rubbing, pressure on the eye, as seen with floppy eyelid syndrome and some lid and orbital tumors, is also a factor in the etiology KC. Unilateral lesions have been shown to be related to KC in the affected eye. This observation has also been reported with eye rubbing and unilateral atopic eye disease, in which the affected and most rubbed eye develops KC.
CONTACT LENS WEAR
The etiological relationship between KC and CL wear is uncertain. Given the early onset of myopia and astigmatism in KC, it is the case that many of these individuals will wear CLs. Similarly, many individuals in the general population also wear CLs and some of these wearers may have subclinical KC. CL wear can induce topographic changes in the cornea, rigid gas permeable CLs more so than soft lenses, with the former inducing early KC-like topography, which in the early stages is reversible on discontinuation of CL wear.
ATOPY AND ALLERGY
Atopy represents an inherent (genetic) predisposition to developing allergic eye disease. It is an enhanced type 1, immunoglobulin E (IgE)-mediated immune response to environmental allergens (antigens), especially those inhaled, ingested, or that make direct contact with the ocular surface. Allergy refers to any immune response; hence, all atopic reactions will be covered under the term allergy but not all allergic responses are atopy. Atopic conditions are associated with 30% to 50% of cases of KC and include vernal keratoconjunctivitis (VKC), atopic keratoconjunctivitis (AKC), atopic dermatitis (eczema), hay fever, and asthma. , , Serum IgE levels are significantly elevated in 50% or more of patients with KC and are even higher in those with atopy. , All these conditions are present in a higher proportion in patients with KC compared with the general population.
CONNECTIVE TISSUE DISORDERS
Many connective tissue disorders have KC as a manifestation of their clinical spectrum of signs, suggesting that the corneal collagen changes leading to KC are a manifestation of systemic disorders of collagen metabolism. In the overall scheme of things, the disorders are rare and the number of cases of KC with these associations is therefore small. However, the association with diseases such as mitral valve prolapse, Ehlers-Danlos syndrome, pseudoxanthoma elasticum, osteogenesis imperfecta, and others does point to a fundamental affection of collagen metabolism. Other seemingly unrelated associations are also important, particularly systemic and ocular conditions with a genetic basis such as Apert, Bardet-Biedl, Crouzon, Down, and Turner syndromes; Leber congenital amaurosis; retinitis pigmentosa; Avellino and granular dystrophies; and endocrine dysfunction such as in the thyroid and sex hormone glands. KC is also significantly associated with learning disability, as part of one of the aforementioned genetic disorders or from other causes.
Histocompatibility antigens HLA B5 and B15 are increased in KC and HLAb7 is found with reduced frequency in KC, compared with controls.
Clinical Pathology
Pathological changes seen in KC relate to the underlying, usually bilateral but asymmetric, thinning and ectasia of the cornea. This results in increased curvature of the cornea inducing a change in the refraction of the optical system of the eye causing myopia and myopic astigmatism, with the latter becoming irregular as the disease advances. Myopia is also secondary to an increase in the axial length of the eye. The vitreous cavity length of KC eyes is reported to be longer by a mean of 0.55 mm compared with emmetropic controls. The anterior chamber depth and axial length too are significantly greater. The corneal thickness and radius of curvature of the cornea are decreased in KC. The characteristic cone can be small and round, central “nipple cone,” or oval shaped, and predominantly inferonasal or an inferotemporal “oval cone.”
Histopathology and In Vivo Confocal Microscopy
EPITHELIUM
The corneal epithelial cells are irregular in shape and show degradation of cell membrane, with an epithelial layer of irregular thickness. Epithelial abnormalities such as blebbing, thinning, and degeneration are noted early in KC. Ferritin molecules accumulate in basal intercellular spaces and in intracytoplasmic vacuoles, especially at the base of the cone, resulting in the clinical sign of Fleischer ring seen in 30% of cases. Epithelial changes are postulated to initiate KC by inducing release of proteolytic chemicals leading to the fragmentation of Bowman’s layer. The basement membrane of the epithelium is thickened and disrupted. Fibrillar debris is seen accumulated beneath the basement membrane. Reduced basal epithelial cell density, preceded by degenerative changes in the form of enlargement and irregular arrangement of the basal epithelial cells, is also demonstrated by in vivo confocal microscopy (IVCM).
BOWMAN’S LAYER
Fragmentation of Bowman’s layer is a common feature seen in 70% of cases, especially those with oval cones. , The breaks in Bowman’s layer are filled with abnormal collagen derived from underlying keratocytes, fibroblasts, and overlying epithelium. Epithelial cells in Bowman’s layer breaks expressing the integrin α3β1 are seen just outside the cone. Linear scars so produced extend across Bowman’s layer to the anterior stroma and are responsible for the clinical sign of anterior white lines. Ultrastructure examination demonstrates infiltration of the Bowman’s layer with fine cellular processes and fragments that stain positive for vimentin, suggesting that they originate from keratocytes.
STROMA
The most marked changes in KC are seen in the corneal stroma, especially in the region of the cone. These changes include a reduction in collagen fibers, thinning of the lamellae, and redistribution of the lamellae such that there are fewer lamellae in the cone with a more compact arrangement. With progression, the lamellae become wider, thinner, and disorganized, with loss of normal architecture. A reduction in interlamellar adhesions and interlacing of the lamellae at the apex of the cone with reduced number of lamellar insertions in the Bowman’s layer have been described.
Corneal stromal cells, the keratocytes, show reduced density, especially in the anterior stroma ( Fig. 5.1 ). Keratocytes also show morphological changes in the form of pseudopodia, excessive cytoplasmic organelles and vacuolation, and nuclear changes. Some keratocytes are surrounded by fibrillogranular material and intercellular debris. , The reduction of keratocyte density, attributed to accelerated keratocyte apoptosis, , is also seen as a consistent feature on IVCM.

VOGT’S STRIAE
These fine vertical lines are present in the deep stroma and the Descemet membrane (DM). These are reported to be due to the unraveling of stromal lamellae along their lengths with opening of their bifurcations, which lead to the formation of undulations or wrinkles in the collagen lamellae. The striae are mainly formed of collagen VI fibrils bound together with glycosaminoglycans (GAGs), lumican, and keratocan. Keratoconic eyes with Vogt striae are at greater risk of acute hydrops. On IVCM in acute corneal hydrops, the presence of certain unusual cell phenotypes within the epithelium and in the stroma can predict the formation of new blood vessels and subsequent development of corneal neovascularization.
DESCEMET MEMBRANE AND ENDOTHELIUM
The DM shows variation in thickness, nodularity, and folds in 8% of cases, and endothelial changes such as pleomorphism and polymegathism, which may be secondary to mechanical stress and hypoxia from CL wear. , On IVCM, endothelial cell density (ECD) shows a positive correlation with pachymetric parameters and significant negative correlation with keratometric and elevation parameters, suggesting that ECD is associated with the progression of KC.
CORNEAL NERVES IN KC
Increased visibility and prominence of stroma nerves on slit-lamp examination are an early sign of KC. IVCM and whole-mount staining techniques have demonstrated a range of significant changes in corneal nerves, throughout their course, in all stages of KC ( Fig. 5.2 ). There is a significant decrease in the morphometric parameters of subbasal nerves including central corneal nerve branch density, nerve fiber length, total branch density, and nerve fiber area. Some structural-functional disconnect is present, as a reduction in corneal sensation has been demonstrated only among CL-wearing KC patients. IVCM and immune-histological studies on KC tissue obtained during corneal transplant surgery have revealed abnormal subbasal nerve architecture with loss of the normal radiating and central whorl pattern. This is replaced by a tortuous, coiled network of nerve fiber bundles, some terminating in closed loops, within the region of the cone. , Subbasal nerves fibers at the base of the cone present as concentric rings that follow the contour of the base. The bulb-like termination of stromal nerves anterior to the Bowman’s layer is thickened in KC corneas. ,

Stromal nerve bundles are thicker than normal, especially within the area of the cone, explaining why prominent corneal nerves are often seen on clinical examination. Some authors have linked these nerve changes to progression of the disease. On histology, stromal nerves within the conical region show a series of alterations of varying severity and have been classified into three grades based on the extent and severity of the morphologic changes. In grade 1, mild looping and coiling of the central stromal nerve bundles is seen. In grade 3, an excessive sprouting of tortuous nerves forms a very complex network within the central cornea, sparing the peripheral innervation, and suggests a pathologic stimulus that is predominantly confined to the cone. Changes in the subbasal nerve orientation are likely to be due to the loss or disturbance of subbasal nerve-epithelial cell interaction. In addition, bulging of the central cornea and subsequent mechanical stretching of the subbasal nerves would distort the orientation and disrupt the normal whorl orientation. ,
Whether nerve alterations in KC are associated causally or result from the overall metabolic derangement remains unclear. Nevertheless, these findings provide evidence of the involvement of corneal nerves in KC and indicate that neurodegeneration is likely to play a role in the pathophysiology of the disease and its progression. ,
ACUTE HYDROPS
In acute hydrops, the DM and Dua’s layer (DL; pre-Descemet layer) both rupture, and the broken ends curl with the endothelial cells outside. A break in DM alone does not lead to acute hydrops. Breaks in both layers can be seen separately on optical coherence tomography (OCT) and are associated with adjacent DM and DL detachment over a variable area. The stromal thickness of stromal lamellae increases and the space between them widens with accumulation of fluid lacunae. The collection of fluid is due to a direct passive ingress of aqueous humor. When acute hydrops is associated with hyphema, for example, after blunt trauma to a keratoconic eye, red blood cells can be seen in the stromal fluid lacunae. The overlying Bowman’s layer may rarely break, causing epithelial edema and a positive Seidel’s sign. Acute hydrops resolves by the regeneration of a very thin DM and fibrosis along the torn edges of DM and DL. The relatively acellular DL shows infiltration with keratocyte-derived cells in chronic cases. Subepithelial scarring may also occur and extend into the anterior stroma.
Biochemical Pathology
The morphological changes noted in KC are related to a plethora of biochemical changes that occur in the tear film, all layers of the cornea, and the aqueous humor and beyond, manifesting in the circulatory system. , These biochemical phenomena are primarily attributable to the three underlying processes : (1) alteration of extracellular matrix (ECM) composition and enzymatic degradation of the cornea; (2) inflammation; and (3) oxidative stress.
ALTERATION OF EXTRACELLULAR MATRIX COMPOSITION AND ENZYMATIC DEGRADATION OF THE CORNEA
Collagen and proteoglycans, including keratan sulfate (e.g., lumican, keratocan, and osteoglycin) and chondroitin sulfate (e.g., biglycan and decorin), represent the main constituents of the ECM of cornea. Type I, III, V, VI, and XII collagens, which are normally present in the corneal stroma, are shown to be reduced in KC, , suggesting a generalized loss of the ECM. Similarly, a reduction in type I, VI, VII, XII, and XIII collagens has also been reported in the corneal epithelium. The loss of collagen content in the cornea has also been indicated by a reduction in hydroxyproline content and prolyl-4-hydroxylase, which is an enzyme essential for hydroxylation of proline residues of collagen.
Alteration of GAGs and proteoglycans, including keratan sulfate and chondroitin sulfate, is similarly observed in KC. , Studies have shown that the amount and gene expressions of major structural proteins, including lumican, keratocan, biglycan, and/or decorin, are significantly reduced in the corneal stroma of keratoconic eyes. , These proteoglycans are involved in the regulation of collagen fibrils, matrix assembly, and corneal transparency, , and reduction of these structural proteins may lead to corneal instability and contribute to the development of corneal thinning and ectasia. Transforming growth factor beta (TGFß) signaling, which regulates the production of ECM by keratocytes, is shown to be affected in KC. In addition, dysregulated expressions of a number of genes coding for ECM proteins have been reported, including the upregulation of TGFBI and downregulation of THBS1, ADAMTS1, and SPP1, among others.
Progressive stromal thinning in KC has been attributed to the enzymatic degradation of the cornea secondary to a dysregulated balance between proteases (e.g., matrix metalloproteinases [MMPs]) and proteases inhibitors (e.g., tissue inhibitors of metalloproteinases [TIMP]). , MMPs, particularly MMP-1 and MMP-9 (or gelatinase B), have been shown to be upregulated in the tears and corneas of patients with KC. The level of MMP-9 in keratoconic eyes could increase as much as 10-fold when compared with normal eyes and subclinical keratoconic eyes, and it might correlate with the severity of KC. Interestingly, Shetty et al. observed that the progression of KC was arrested when MMP-9 level was reduced by topical ciclosporin treatment. The role and expression of other types of MMPs, such as MMP-2 (or gelatinase A), MMP-3, MMP-7, and MMP-13, in KC have also been investigated but results were somewhat variable and conflicting at times. , Increased levels of other types of degradative enzymes such as cathepsin-B, cathepsin-G, and cathepsin-V/L2, phosphatases, and esterases are also demonstrated in KC.
In addition to the increased MMP-driven proteolytic activity, downregulation of protease inhibitors, particularly TIMPs (the main inhibitors of MMPs), alpha-1 proteinase inhibitor, and alpha-2 macroglobulin, has been observed in keratoconic eyes, contributing to corneal stromal lysis and development of KC. , ,
INFLAMMATION
Although KC is traditionally regarded as a non-inflammatory corneal disease, emerging evidence suggests that inflammation plays a key role in the etiopathogenesis of KC. , At the ocular surface (particularly the tears and the corneal epithelium), a range of inflammatory cytokines, including interleukin (IL)-4, IL-5, IL-6, IL-8, and tumor necrosis factor (TNF)-α, , are shown to be upregulated. Moreover, Pahuja et al. observed that the corneal epithelium obtained from the cone apex of keratoconic eyes expressed a significantly higher level of inflammatory cytokines (e.g., IL-6 and TNF-α) and MMP-9 when compared with the peripheral corneal epithelium. This phenomenon may explain the focal structural weakness observed in KC.
In addition, KC is strongly linked to eye rubbing and atopic diseases. Studies have shown that trauma induced by eye rubbing could promote ocular surface inflammation and proteolytic activity in both keratoconic and healthy eyes, evidenced by the increased level of proinflammatory mediators and MMPs in tears. , Therefore, patients with KC must be advised against eye rubbing, to reduce the inflammatory drive and the risk of disease progression. Independent of the effect of eye rubbing, a significantly higher level of inflammatory cytokines (e.g., IL-1ß, IL-4, IL-5, IL-6, and IL-10, and TNF-α) has been shown to be expressed in tears during the active phase of atopic diseases such as VKC and AKC. These inflammatory responses may account for the increased severity of KC observed in patients with VKC.
OXIDATIVE STRESS
Oxidative stress, a phenomenon related to the disrupted balance between reactive oxygen species (ROS) and antioxidants, has been postulated to be another key mechanism in the pathogenesis of KC. , Superoxide dismutase (SOD), catalase, and glutathione peroxidase represent the most important first-line defense antioxidant enzymes in the human body. Behndig et al. observed that the extracellular activity of SOD in central corneas was only half in KC compared with normal corneas, potentially contributing to an increased oxidative stress. More compellingly, examination of the previously transplanted corneas in patients with an original diagnosis of KC similarly revealed a reduction in extracellular SOD, suggesting that the cause of reduced SOD level was related to reduced host cellular activity.
Kenney et al. observed a significantly upregulated messenger RNA (mRNA) level of degradative enzymes such as cathepsins-B, -G, and V/L2 in keratoconic eyes, which could stimulate the production of hydrogen peroxide and upregulate the expression of catalase as a compensatory host antioxidative response. The increase in endoplasmic reticular stress and oxidative stress in the corneal epithelium and stroma of keratoconic eyes was further supported by a comprehensive proteomic study.
Proteomic analysis of the aqueous humor obtained from keratoconic eyes has revealed a dysregulated expression of the proteins that participate in proteolytic regulation and oxidative stress response. Furthermore, the plasma concentration of homocysteine has been shown to be significantly higher in patients with KC compared with healthy subjects. Homocysteine is known to increase oxidative stress via the activation of protease-activated receptor (PAR)-4, which enhances the production of ROS. Hence, a higher level of homocysteine indicates the mechanistic role of oxidative stress in KC.
Genetics of Keratoconus
Although KC often presents as an isolated clinical entity, the implication of genetics in the development of KC has been supported by various observations and studies such as history of familial inheritance (5%–20%), twin studies, candidate gene analysis, genome-wide linkage studies (GWLS), and genome-wide association studies (GWAS). ,
GWLS are comprehensive genetic analyses used to map the relevant genetic markers of a particular disease across the genome of the affected families. GWAS is another powerful genetic tool used to analyze the genetic variations across the genomes, particularly single nucleotide polymorphisms (SNPs), of a population to determine genotype-phenotype associations. To date, GWLS have been used to map out a range of genetic markers and changes in KC, including LOX gene at the 5q23.2 chromosomal region (which encodes for lysyl oxidase—a collagen cross-linking enzyme), CAST gene at the 5q15 region (which encodes for calpastatin—a protease inhibitor), and DOCK9 gene at 13q32.3 region (dedicator of cytokinesis 9), among others. In addition, GWAS have successfully identified several genetic mutations in patients with KC, including HGF SNP rs3735520, RAB3GAP1 SNP rs4954218, PNPLA2 gene, TGFBI gene, VSX1 gene, and others. , A meta-analysis of around 7000 participants also revealed that mutation in central corneal thickness (CCT)-associated loci, namely FOXO1 and FNDC3B, could significantly increase the risk of KC.
Clinically, the spectrum of myopia, myopic astigmatism, KC, pellucid marginal degeneration, and keratoglobus are seen in family members and in the two eyes of the same individual, suggesting a phenotype spectrum of an underlying common etiopathogenic mechanism.
Biomechanics in the Pathophysiology of Keratoconus
Biomechanics is defined as “the development, extension, and application of mechanics for a better understanding of physiology and physiopathology and consequently for a better diagnosis and treatment of disease and injury.” Understanding of certain terms used to describe physical properties helps in the understanding of biomechanics.
Stress refers to the tension, force, or pressure exerted on the cornea.
Strain refers to the amount by which the cornea is stretched or deformed in response to stress. Strain is the response to stress.
Shear refers to the strain (change in shape) induced by the sliding or lateral shifting of the lamellae in relation to one another, caused by stress (pressure) in the substance of the cornea.
Shear stress is the stress (pressure or sliding force) that causes the change in shape (strain) without a change in volume of the cornea.
Viscosity is the resistance of a liquid to flow, which is determined by the frictional forces within the fluid. Viscosity measures the consistency of a fluid. The greater the viscosity the greater the inability to flow. Corneal constituents can be regarded as fluid of high viscosity.
Elasticity is the ability of the corneal tissue to resume its normal shape after being stretched or compressed. In other words, it is the ability of the deformed cornea to return to its original shape and size when the forces causing the deformation are removed.
Viscoelasticity refers to a substance that has both viscosity and elasticity. The cornea is viscoelastic. Any stress applied to the cornea results in immediate elastic strain and a slightly delayed viscous strain dependent on duration of the stress.
Corneal hysteresis refers to the measure of the amount of stress (energy of the pressure or force applied) that can be absorbed or distributed within the stroma. Hysteresis of a substance creates a lag between the application of stress and the deformation of the substance and conversely between the cessation of the applied stress and the recovery. For example, when one lies on a mattress, the mattress is first compressed (energy distributed within the mattress—hysteresis) before it assumes a degree of concavity. Corneal hysteresis is calculated by measuring the difference between the pressure at which the cornea bends inward during an air jet applanation and the pressure at which it bends out again. This is determined by an infrared laser during an intraocular pressure measurement.
The corneal resistance factor is a measurement provided by an instrument called the Ocular Response Analyzer (Reichert, Buffalo, NY). It is a measure of the overresistance of the cornea and determined largely by the elasticity of the cornea.
The cornea is a viscoelastic tissue that displays all the biomechanical properties described earlier, enabling it to function as a biological mechanotransducer of stress. The corneal stroma accounts for most of the corneal strength. The contributions of the epithelium, DM, and endothelium are generally weak, and the contribution of Bowman’s layer is still debatable. The anterior 40% of the corneal stroma is the strongest region, whereas the posterior 60% of the stroma is at least 50% weaker according to tensile strength studies in human donor corneas. The impact of the recently discovered pre-Descemet layer or DL on the posterior corneal biomechanics and its implications in corneal disease and during corneal surgeries are being investigated.
Alterations in corneal biomechanical properties are believed to precede corneal tomographical changes in KC. Reduced mechanical stability has been attributed to many factors such as apoptosis and subsequent reduction in keratocyte density, loss of stromal fibrils, altered architecture and orientation of collagen fibers, and reduced number of cross-links. Therefore in vivo characterizations of corneal biomechanical parameters may enable the early diagnosis of subclinical (forme fruste) KC. Although both corneal hysteresis and corneal resistance factor describe uncertain viscoelastic properties, corneal hysteresis (viscous damping) is produced by the viscous GAGs, proteoglycans, and ECM interactions. Both corneal hysteresis and corneal resistance have been reported to be significantly weak in keratoconic corneas compared with healthy ones. The results lack specificity and sensitivity.
Another device, the Corvis ST, utilizes a noncontact air puff tonometer, combined with a high-speed Scheimpflug camera (4330 frames per second) to image a cross-section of the cornea during deformation. The device records 8.0-mm wide horizontal sections of the cornea and analyzes the deformation pattern. The software provides a range of measurements for different corneal biomechanical properties. Deformation amplitude (DA), which is the displacement from original position at highest concavity, is significantly larger in keratoconic corneas (1.2531–1.3230 mm) compared with healthy corneas. The sensitivity of DA is poor and, therefore, it cannot be used clinically to differentiate between normal and keratoconic corneas.
There is no doubt that significant biomechanical changes occur in the cornea in KC. These are likely to be secondary to the biochemical and cellular changes but in turn can affect cell behavior and contribute to the progression of KC.
The wide range of conditions that are associated with KC in a cause-and-effect, consequential, or coincidental relationship suggest that KC might be a common manifestation of different pathogenetic processes. Some environmental factors such as atopy and allergy, eye rubbing, and CL wear seem to be more important and common than others. Of several disorders with underlying genetic predisposition, Down syndrome stands out. However, the numerous other, though rare, associations and the well-documented racial and familial clusters of KC together with other ectatic corneal disorders such as keratoglobus and pellucid in different family members and also in the two eyes of the same individual strongly indicate a genetic basis. The bilateral but significantly asymmetrical nature of KC and forme fruste KC confound the pathogenetic hypotheses. Structural changes, namely thinning of the cornea, rearrangement of collagen lamellae, disruption of the Bowman’s layer, and stretching of the cornea causing anterior protrusion, are fairly consistent features of KC, but here too are inconsistencies as seen with the different locations of the cone and the primary affection of the posterior cornea in posterior KC.
Putting all the evidence together in one coherent sequence of events that can translate into the pathogenesis of KC is difficult despite, or perhaps because of, the vast number of studies and the data generated from them. Primarily environmental factors, on the background of a genetic predisposition in some individuals, are likely to be the initiating stimulus. Induced cellular changes, primarily in the stromal keratocytes but not excluding the epithelium and the endothelium, lead to biochemical changes and physiological stress. The enormity of nerve changes demonstrated in KC suggests that neurons, although distantly located, must be included in any theory on the pathogenesis of KC. Oxidative stress, enzymatic degradation, and inflammation join forces to alter the stromal matrix, ground substance, collagen, and elastin, leading to thinning. These alterations can be a consequence of insufficient or defective formation and degradation and destruction of normally formed elements of the matrix. The resultant stroma loses its physical structure and biochemical resilience. Elements of corneal biomechanics then come into play and alter the shape of the cornea, producing a conical protrusion that clinicians call KC.
The simplistic sequence described earlier does not imply that when one event leads to the other, the preceding event ceases. The whole process persists in a relentless cycle with perpetuation of the pathology and clinical progression. To illustrate, if atopy and eye rubbing were the initiating events, they will continue to influence the process when cellular and subcellular events are occurring in the stroma; alterations in corneal biomechanics will continue to be influenced by physiological biomechanical forces of blinking and corneal pulsation with heartbeats, compounded by eye rubbing. Thus, what is described as a linear process is in reality a complex maze of actions, counteractions, and interactions. Understanding of pathophysiology is crucial to developing treatment strategies. We are beginning to learn how to influence the process but are far away from a cure. KC remains an enigma.
References
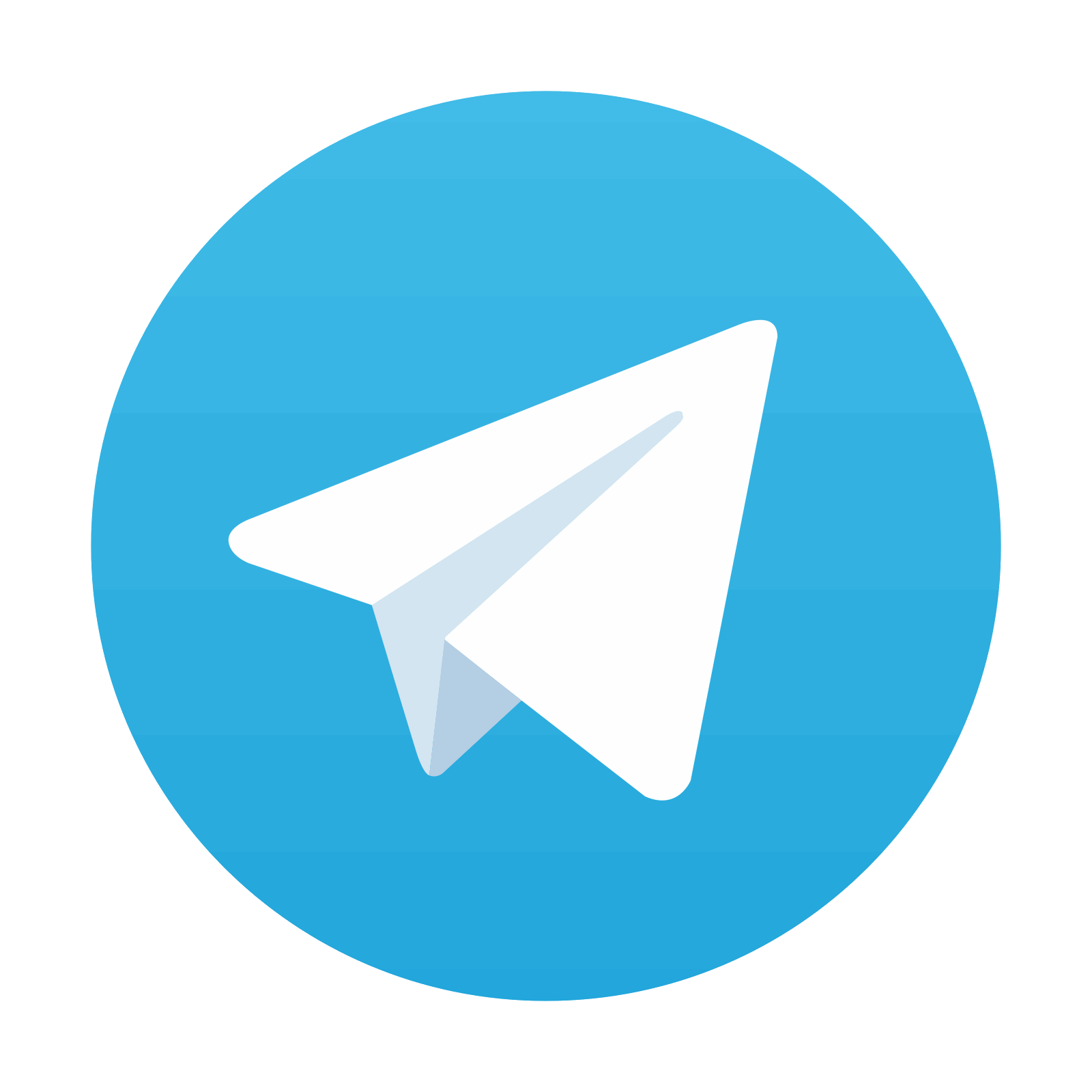
Stay updated, free articles. Join our Telegram channel

Full access? Get Clinical Tree
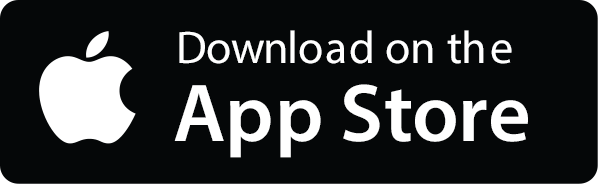
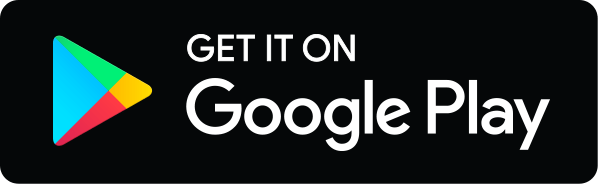
