5 Corneal Topographic Analysis for Intraocular Lens Implantation
5.1 Introduction
As refractive outcomes have become the focus of lens-based surgeries, with both patients and surgeons expecting optimal refractive results after intraocular lens (IOL) implantation, the need for preoperative corneal topographic analysis has moved from useful in some cases to necessary in most. Most surgeons understand the role of topography with corneal refractive procedures to screen out patients with subclinical ectatic corneal disorders; however, corneal topography is particularly useful in the context of IOL surgery to measure and evaluate corneal power and curvature and to determine the presence and extent of irregular astigmatism in addition to ectatic corneal screening. Topography also provides information about the homogeneity of the tear film, and the potential impact of seemingly insignificant scars or epithelial basement membrane dystrophy (EBMD). 1 All of these factors are critical in determining which IOL to implant and what possible refractive outcomes can be achieved. All cataract surgeons will need to become familiar and comfortable with topographic evaluation to maximize outcomes and prevent detectible complications.
This chapter highlights basic aspects of corneal topographic analysis that are necessary to use this technology accurately, as well as the importance of corneal topography for the calculation of the precise IOL power in various situations, including all IOL surgery with specific refractive goals, toric IOL implantation, and multifocal IOL implantation.
5.2 Using Topographic Data for Keratometry
The most obvious contribution of corneal topography in the field of cataract surgery is determining accurate keratometry measurements that can be used for reliable IOL power calculations. Through the implementation of reproducible measurements derived from the analysis of hundreds of points and a color-coded scale, corneal topography represents a greater stride toward more predictable data when compared with manual keratometry.
5.2.1 Placido Topography
Placido disc-based topography projects circular mires over a broad area of the cornea, allowing corneal curvature assessment at different points on the whole corneal area (Fig. 5.1), whereas the manual keratometer calculates corneal curvature based on two measurements at the steepest meridian and two measurements at the flattest meridian. 2 , 3

There are several different topographers available in addition to Placido disc-based corneal topography, each of which uses different technologies to obtain and process data.s. Literatur
Previous studies have found a high level of repeatability in devices that employ different technologies, including automated keratometry, Placido discs, rotating Scheimpflug, dual Scheimpflug imaging, and scanning slit technology.s. Literatur , 5 Most recent studies suggest that values of simulated and mean keratometry seem to be more reliable with either a Placido disc-based corneal topographer or a combined Placido disc-based and dual Scheimpflug tomographer (Fig. 5.2 a, Fig. 5.3 a) when compared with a Scheimpflug tomographer (Fig. 5.2 b, Fig. 5.3 b) with a single rotating camera, because the two former devices provide a tighter data spread when analyzing the limits of agreement of keratometry measurements. 6


5.2.2 Scheimpflug Tomography
Dual and rotational Scheimpflug systems have become popular due to their ability to provide information about corneal thickness and posterior surface in addition to anterior corneal curvature (Fig. 5.2). The dual Scheimpflug system (Galilei, Ziemer Ophthalmic Systems) integrates rotating Scheimpflug camera data and Placido ring data to measure anterior and posterior corneal surfaces (Fig. 5.2 a). Height data from the Scheimpflug images, and slope data, converted into height data from the Placido, are merged to calculate a surface fitted to the anterior corneal surface. The posterior corneal surface is measured using only data provided by the Scheimpflug system. The system acquires between 15 and 60 Scheimpflug images per scan and two Placido top view images at 90° apart, as the cameras rotate around the central axis. Placido and Scheimpflug data are acquired simultaneously, and then a motion correction algorithm is applied to the combined dataset. Through ray tracing, the dual-Scheimpflug system is also theoretically able to determine the total corneal power (TCP), which may be particularly useful to determine the IOL power after myopic refractive surgery. The Scheimpflug camera rotates 360° around the line of fixation, with a slit of 475 nm wavelength monochromatic light to illuminate the cornea, and records 50 sectional images in the anterior segment. From the pictures, the system derives a three-dimensional model of the anterior segment from up to 138,000 true elevation points.
Direct comparative studies between Scheimpflug with a single rotating camera, such as the Pentacam (Oculus) (Fig. 5.2 b) and dual Scheimpflug technology showed that there are significant differences in the keratometry values obtained and that they should not be used interchangeably for anterior segment measurements, neither for normal nor for keratoconic eyes. 7 , 8 The scientific evidence about predictability when measuring keratometry is of particular importance when calculating IOL power and selecting toric IOL models. Recently, some authors have advocated the use of combined measurements of astigmatism, acquired from different technologies, to improve the accuracy of toric IOL calculations. 9
5.3 Corneal Topographic Evaluation: Beyond Keratometry
Color corneal topography allows rapid evaluation of corneal surface curvature by evaluating corneal keratometry and power over a large area and assigning a color code to curvature values derived by measuring the distance between individual Placido rings (Fig. 5.1). Using this system, changes in corneal curvature, especially focal and asymmetric changes, become readily apparent. Accurate topographic evaluation requires a systematic, consistent approach to the evaluation of these scans (Table 5-1).
Identify color scale |
Confirm adequate scan |
Evaluate for artifacts |
Identify topographic pattern |
5.3.1 Color Scale
The first step in topographic analysis is determination of the color steps being used in the topography. Most machines are programmable to output in 0.25 to 2 diopter (D) scales; however, most refractive surgeons use 0.5 or 1 D scales. Increasing the sensitivity with smaller scales (0.25 D) adds “noise” to the analysis by including multiple colors in any pattern, whereas reducing the sensitivity with large scales (2 D) misses all but large curvature changes. Because refractive surgeons are looking for subtle curvature differences, most advocate using the 0.5 D scale, which provides a balanced association of sensitivity and specificity. Color step identification is critical because identical topographies can look significantly different based on the color step used (Fig. 5.4), and it is critical that the topography pattern presents the scale the surgeon is accustomed to viewing.

5.3.2 Topographic Pattern Analysis
Once the color steps are identified, specific topographic patterns can be evaluated. Although a variety of subtle differences exist between many specific topographies, the patterns can be divided into four major categories (Table 5-2): normal, mildly asymmetric/suspicious, highly asymmetric/suspicious, and abnormal. A spectrum of topographic patterns from normal to suspicious is demonstrated in Fig. 5.5.
1. Normal (including round, oval, or regular bowtie patterns) |
2. Mildly asymmetric |
|
|
3. Highly asymmetric |
|
|
4. Abnormal patterns |
|
|
|

Although these pattern classifications are critical for determining corneal refractive surgical candidacy, especially in younger patients, differentiating subtle pattern differences is less essential for lens-based surgery. However, these patterns become important if any laser vision correction (laser in situ keratomileusis [LASIK] or photorefractive keratectomy [PRK]) is contemplated for residual refractive error after lens-based surgery, and full screening must be done preoperatively to determine whether or not patients are adequate laser vision correction candidates during the initial decision-making process.
Abnormal patterns, such as significant focal steepening (Fig. 5.6) or an asymmetric bowtie with skewed radial axis (AB/SRAX) (Fig. 5.7), may represent stable curvatures in older patients; however, these patients are still at risk for progressive irregular astigmatism with laser vision correction. 10 Pellucid marginal corneal degeneration (PMD) patterns typically exhibit a “crab-claw” pattern, with steepening in the far inferior periphery between the 4 and 8 o’clock meridian (Fig. 5.8).



Most patients presenting for surgical evaluation have at least mild topographic asymmetry. Among these asymmetric patterns, against-the-rule patterns warrant special attention. Against-the-rule steepening is an unusual topographic pattern for younger patients but is relatively common for patients presenting for cataract evaluation. It is important to distinguish stable against-the-rule as opposed to progressive PMD patterns, especially in younger patients (40s or 50s). In against-the-rule astigmatic patterns, special attention should be paid to the far periphery of the image to make sure that the pattern does not begin to “droop” downward, indicating the early stages of a crab-claw-type pattern.
Topographic asymmetry between eyes should also be seen with caution because this relevant pattern represent an early stage of progressive ectatic disorders.
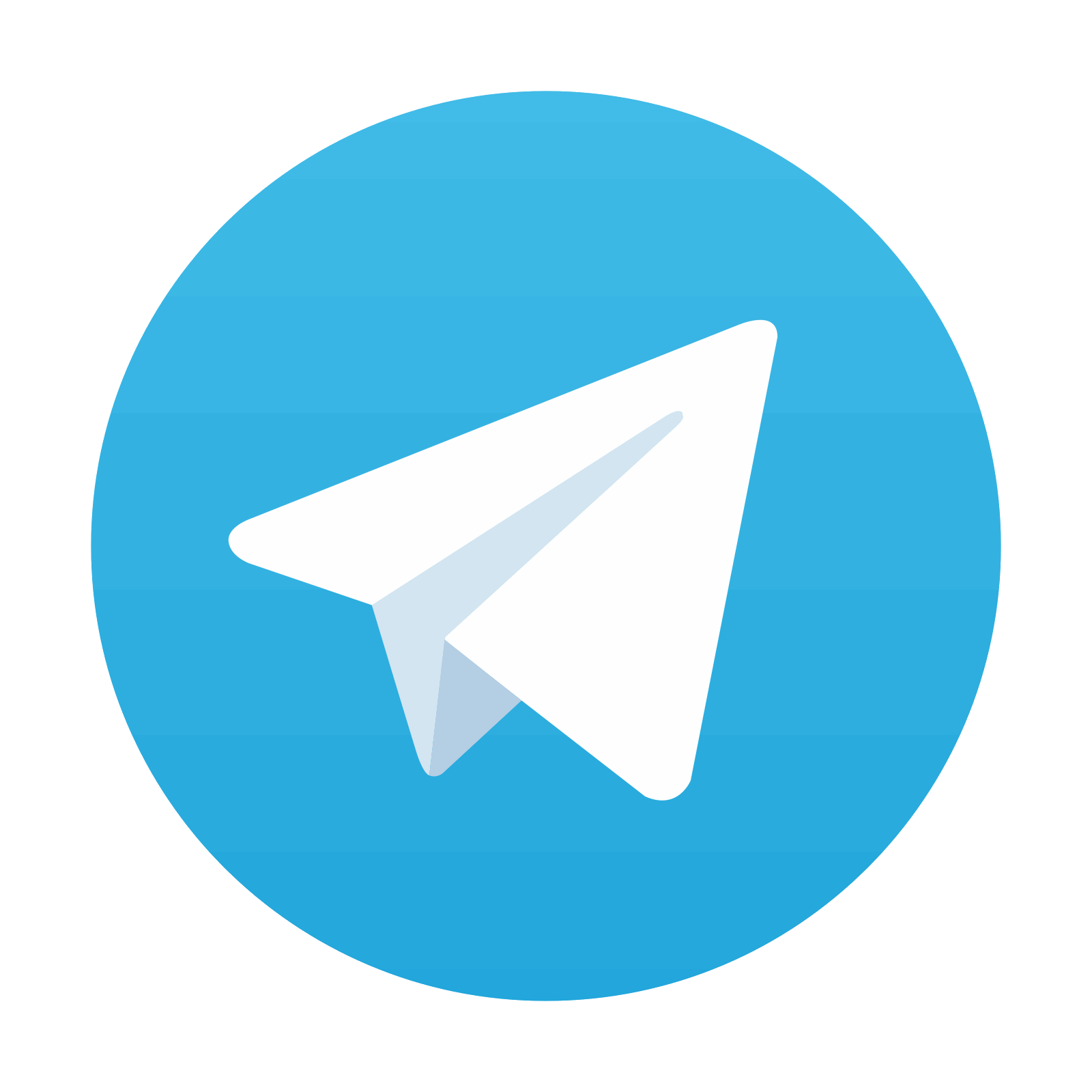
Stay updated, free articles. Join our Telegram channel

Full access? Get Clinical Tree
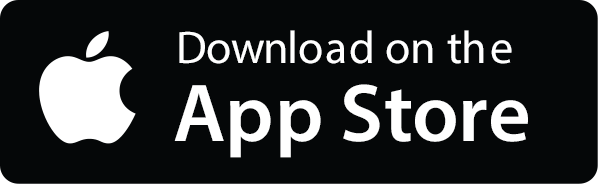
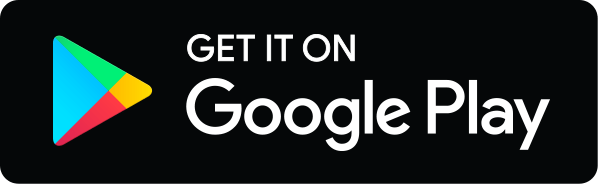
