The role of the central visual pathways is to process and integrate visual information that travels to the brain by means of the optic nerves. Although the eye is responsible for transducing patterns of light energy into neuronal signals, it is the brain that is ultimately responsible for visual perception and cognition. Vision at the level of the central nervous system is perhaps the best understood of all the sensory systems, partly because there is a wealth of information on its neuroanatomic and functional organization. In this chapter, an overview of the central visual pathways is given, beginning with a brief description of the retinal projections and their putative functional roles and ending with an overview of the consequences of lesions along the visual pathway. Schematic views of the central nuclei that receive a retinal projection are shown in Figures 27.1 and 27.2 . The most significant, in terms of the number of optic nerve fibers, is the projection to the lateral geniculate nucleus (LGN) in the thalamus. The LGN, described in detail in Chapter 29 , is an important relay in the visual pathway involved with form vision. The precision and specificity of the retinogeniculate connections are crucial to form vision. From the LGN, the visual pathway proceeds to the primary visual cortex, V1. It is at the level of V1 that visual signals undergo complex processing, which is detailed in Chapter 30 . Visual processing of a combination of stimulus features such as form, color, contrast, motion, texture, depth and is further enhanced by over 30 extrastriate cortical areas, some of which are described in Chapter 31 . Finally, we discuss in detail the role of experience and visual deprivation on the development of the central visual pathways in Section II.


Targets of the retinal projections
The major targets of the retinal ganglion cell axons are the LGN, superior colliculus (SC), pretectum, and pulvinar. There is a weaker projection to several small hypothalamic nuclei (including suprachiasmatic, supraoptic, paraventricular nuclei) and the accessory optic system (AOS) (including the nucleus of the optic tract (NOT) and the dorsal, medial, and terminal nuclei) ( Box 27.1 , Fig. 27.2 and see Fig. 9.8 ).
- •
LGN
- •
Superior colliculus
- •
Pretectum
- •
Pulvinar
- •
Hypothalamic nuclei
- •
AOS
The LGN of the thalamus is the major termination site of the retinal ganglion cells, and plays an important role in the visual pathway leading to the primary visual cortex. Approximately 90 percent of all retinal ganglion cells project to the LGN, which is laminated and displays retinotopic organization. Each LGN layer receives input from a specific eye and class of ganglion cell. While electrophysiological studies have determined that the neuronal signals coming into and leaving the LGN are not appreciably different, the processing that takes place in the LGN appears to be involved in regulating the flow of information to the primary visual cortex (V1), the major projection target of the LGN (see Chapter 29 ).
The SC is a midbrain structure which, in conjunction with the cortical frontal eye fields and the brainstem reticular formation, is involved in the generation of visually guided saccadic eye movements , (see Chapter 9 ). The SC is a laminated, retinotopically organized nucleus, and as seen in the LGN, the retinal projection retains eye segregation with alternating columns of left and right eye terminals forming a banded pattern throughout the superficial layers. Approximately 10 percent of all retinal ganglion cells project to the SC. The majority of retinal axons that terminate in the SC are small caliber, originate from ganglion cells with small dendritic fields, and do not project to other retinal targets.
The pretectal complex, a group of small midbrain nuclei, is just rostral to the SC. It receives signals from a group of small-diameter retinal ganglion cells with large receptive fields and is involved with the control of the pupillary light reflex (see Chapter 25 ) by means of a projection to the Edinger–Westphal nucleus of the oculomotor complex. The pupillary light reflex demonstrates a consensual response primarily resulting from crossed and uncrossed optic nerve fibers that enter each pretectal complex which in turn sends a bilateral projection to the Edinger–Westphal nucleus (see Chapter 9 ).
Retinal ganglion cells also project to three of four major subdivisions of the pulvinar nucleus of the thalamus. , The pulvinar is the largest nuclear mass in the primate thalamus and receives a projection from the small-caliber fibers from the optic nerve and the SC. It projects to several visual cortical areas, including V1, and extrastriate, parietal areas. Thus, the pulvinar represents a pathway that can bypass the LGN to get to V1 and may play a role in processing form vision. More recent studies point to a role for the pulvinar in the coding of the “importance” of visual stimuli (i.e. visual salience or “attention”). It has been shown that the pulvinar integrates neural signals associated with eye and hand and arm movements and may receive signals associated with saccadic eye movements, which suggests its role is also one of formulating reference frames for hand-eye coordination.
Several small hypothalamic nuclei receive a direct retinal projection. The suprachiasmatic nucleus receives a sparse projection from fibers that leave the dorsal surface of the optic chiasma and has been implicated in the synchronization of circadian rhythms. The paraventricular and supraoptic nuclei are likely also involved with the regulation of the light–dark cycle for neuroendocrine functions.
The AOS consists of several small nuclei, the lateral terminal nucleus (LTN), the medial terminal nucleus (MTN), and the dorsal terminal nucleus (DTN), as well as the NOT in the midbrain. The AOS plays an important role in optokinetic nystagmus (OKN) in which slow compensatory and pursuit-type eye movements alternate with fast saccadic-type eye movements in response to viewing prolonged large field motion (see Chapter 9 ). In primates, lesions of the NOT and the DTN have been shown to modify the OKN and reduce or abolish optokinetic after-nystagmus (OKAN).
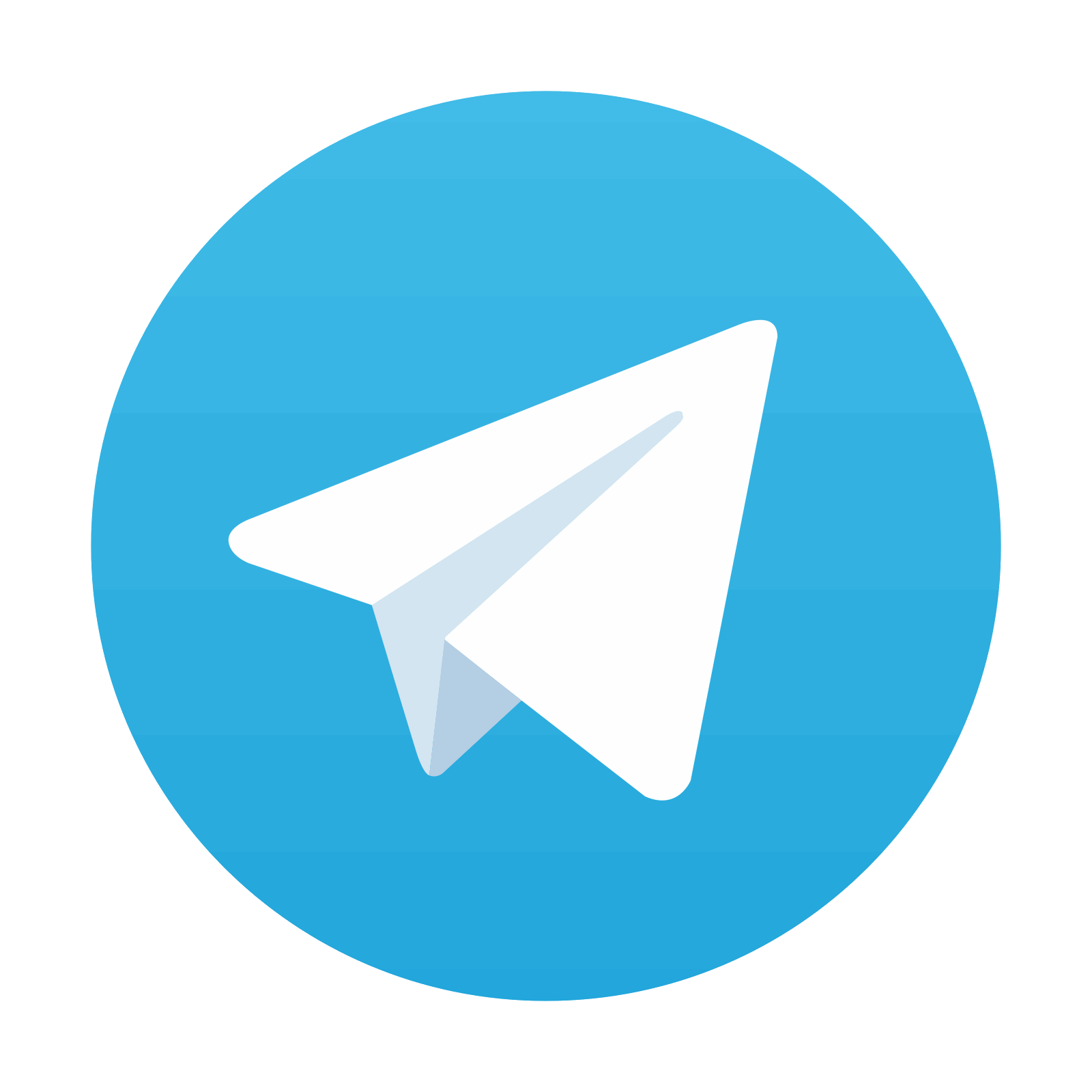
Stay updated, free articles. Join our Telegram channel

Full access? Get Clinical Tree
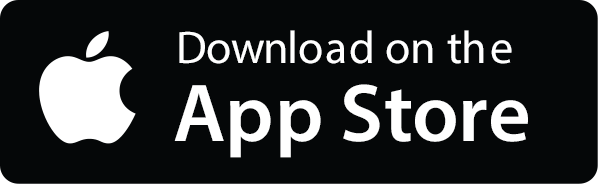
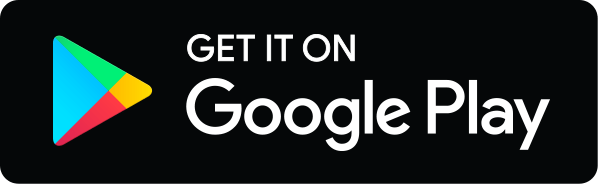