Introduction
In ocular therapeutics, the current literature describes drug delivery limitations posed by outward directed transport mechanisms of the eye. Drug delivery to ocular tissues is restricted by various factors such as limited tissue penetration, rapid clearance rate, and dose-limiting toxicity. Though diffusion plays a role, efflux transport proteins constitute a major driving force behind selective removal of therapeutic agents from ocular tissues. Therapeutic agents must penetrate the globe through the anterior or posterior route, depending on the mode of administration. The cornea forms a major barrier for anterior segment drug delivery. Presence of epithelial tight junctions, along with a wide array of efflux transporters causes poor permeation of drugs across the cornea. Apart from these, the drug molecules should possess optimum physicochemical properties to penetrate the different corneal layers. The blood–retinal barrier (BRB) forms a major barrier to posterior segment ocular drug delivery. The BRB is composed of retinal vascular endothelium and retinal-pigmented epithelium and is categorized as inner BRB and outer BRB. Inner BRB is composed of a monolayer of endothelial cells, which are non-fenestrated, and outer BRB is composed of retinal-pigmented epithelial cells.
Multi-drug resistance mediated by the efflux transporter (pump) is a widely explored field in the area of cancer research. Efflux pumps belong to the ATP binding cassette (ABC) transporter family. Family members such as P-glycoprotein (P-gp), multidrug resistance associated protein (MRP), breast cancer resistance protein (BCRP) and lung resistance protein (LRP) are known to play a vital role in imparting drug resistance to cancer cells. Efflux transporters are known to be protective in preventing the entry of toxic substances into cells which results in outward directed transport of toxins.
The presence of efflux transporters on various physiological barriers protecting the eye was only recently discovered. Their existence in various ocular tissues such as cornea, retina, and conjunctiva has been established at molecular and functional level by various researchers. To a large extent the role of these efflux transporters in ocular drug resistance remains to be explored.
Various drug delivery strategies are employed to bypass the efflux transporters. The prodrug strategy, which involves linking of the active drug molecule to a promoiety, is known to reduce or eliminate the substrate specificity of drugs to the efflux transporters. Our recent findings revealed that amino acid and peptide prodrugs of quinidine, val-quinidine and val-val-quinidine were able to bypass the MRP class efflux transporters on the corneal epithelial cells. Earlier it was shown that val-quinidine and val-val-quinidine can effectively bypass P-gp mediated efflux on the cornea. This chapter comprises a discussion on the presence of efflux transporters in various ocular tissues and their possible role in outward directed transport. Various strategies employed to modulate or bypass efflux transporters are presented.
Efflux transporters – brief history
Though outward directed transport mediated by efflux transporters is a new field in the area of ocular drug delivery research, it has been widely explored in the area of cancer therapeutics. The role of ABC transporters in treatment failure has been attributed to overexpression of efflux transporters.
First evidence of outward directed transport resulting in drug resistance was recognized in 1973. Ehrlich astrocytes accumulated lower amounts of daunorubicin intracellularly primarily due to outward transport. Later a higher-molecular-weight glycoprotein (P-gp) was identified in multidrug-resistant cells. It took a considerable amount of time and effort to confirm the hypothesis that a protein binds to the therapeutic agents or toxins intracellularly and transports them out of the cells utilizing energy from ATP hydrolysis. P-gp was confirmed as a primary drug efflux transporter leading to drug resistance. This protein was later found to be a major efflux pump involved in resistance to various cancer drugs including paclitaxel and doxorubicin. P-gp is also demonstrated as ATP-binding cassette sub-family B member 1 (ABCB1), MDR1 and PGY1. It is also designated as cluster of differentiation 243 (CD243). It is a ~170 kDa transmembrane glycoprotein with 10–15 kDa of N-terminal glycosylation. P-gp has wide substrate specificity and is involved in the transport of lipids, steroids, bilirubin, xenobiotics, steroids, cardiac glycosides (digoxin), glucocorticoids (dexamethasone), HIV drugs (non-nucleoside reverse transcriptase inhibitors and protease inhibitors) and immunosuppressive agents. Apart from being overexpressed in cancerous tissues, P-gp is expressed on various tissues including capillary endothelial cells of the blood–brain barrier (BBB), liver, intestine and renal proximal tubules.
Another drug efflux transporter resulting in outward directed transport is multidrug resistance associated protein (MRP). It was identified in 1992 in a human lung cancer cell line (H69AR) demonstrating multidrug resistance. The cell line did not express P-gp despite conferring significant resistance to the absorption of doxorubicin. Later, the efflux transporter responsible for outward directed transport was found to be MRP.
Apart from MRP and P-gp, various other human ATP binding cassette (ABC) transporters have been identified. A new efflux protein which is currently known as breast cancer resistance protein (BCRP) has been identified at protein level and cloned by three research groups. It is also known as the mitoxantrone-resistance protein (MXR) or placenta-specific ABC protein (ABCP). This protein is an ~70 kDa efflux transporter belonging to the ABC family and proved to play a significant role in drug resistance.
Another new efflux transporter belonging to the ATP binding cassette family is lung resistance protein (LRP). It is also known as major vault protein (MVP) and can cause multidrug resistance. LRP was initially identified in P-gp negative MDR lung cancer cells and is composed of complex ribonucleoprotein particles. Vaults are present mainly in the cytoplasm and partially in the nuclear membrane. These structures are assumed to cause nucleocytoplasmic and intracellular transport. The presence of LRP in cancer cells was found to result in resistance development to cisplatin, doxorubicin, carboplatin and melphalan. Other than the eye it is present in various tissues such as colon, lung, macrophages, adrenal cortex and renal proximal tubules. However, contradictory reports have appeared in the literature questioning the role of LRP or MVP. A few studies revealed that MVP does not play a significant role in drug resistance. The primary physiological function of LRP is not completely understood and is being further evaluated.
Multidrug resistance conferred by efflux transporters is a relatively new area of interest in ocular drug delivery. Most of the transporters are ubiquitously expressed in various tissues of the body and have a protective role. The presence of efflux transporters in various ocular tissues and their role in ocular drug efflux resulting in drug resistance is discussed in the following sections.
Efflux transporters in ocular tissues
P-gp
P-gp in isolated primary culture of rabbit corneal epithelial cells was reported by Kawazu et al. The presence of P-gp at protein level and a series of uptake experiments demonstrated that the tear-side-facing membrane (apical) of rabbit corneal epithelial cells expressed P-gp. This article further concluded that the presence of P-gp on corneal cells protects them from toxic agents. Later the presence of P-gp in human cornea was demonstrated by Dey et al. This report further confirmed the expression of P-gp in rabbit cornea and rabbit corneal epithelial cells. Uptake of Rho 123, a P-gp substrate, was significantly higher in the presence of P-gp inhibitors like cyclosporine A (CsA) indicating the role of P-gp in corneal drug efflux. Ocular pharmacokinetic studies involving male New Zealand Albino rabbits revealed a significant elevation in aqueous humor drug concentrations in the presence of various P-gp inhibitors, i.e. testosterone, quinidine, verapamil and CsA. Moreover dose-dependent suppression in P-gp efflux was evident at various inhibitor concentrations. The presence of P-gp in the cornea was corroborated by a recent report on the quantitative expression of P-gp in human cornea. The relative role of P-gp in the outward directed efflux of drugs used in glaucoma, anti-bacterial and anti-viral agents has yet to be established.
An ocular microdialysis technique was applied to determine the role of efflux transporters on the cornea. Figure 17.1 shows a schematic diagram of the surgical implantation of the microdialysis probe. A well is placed on the top of cornea filled with drug solution. The sample is collected from the anterior end of the probe which protrudes from the cornea. Drug diffuses across the dialyzing membrane placed in the aqueous humor. This technique helps to quantitatively determine the drug concentrations in the aqueous humor at different time points. This study reported that P-gp inhibitors significantly raised drug concentration in aqueous humor (both C max and AUC) ( Table 17.1 ). The results demonstrated that P-gp can play a vital role in conferring resistance to drug absorption across the cornea. This article also suggested the use of efflux pump inhibitors along with therapeutic agents – a strategy that helps to increase the overall ocular bioavailability of drugs which are substrates of efflux pumps.

Drug and/or Inhibitor | AUC 0-∞ | C max |
---|---|---|
Erythromycin | 31.67 ± 4.99 | 0.136 ± 0.009 |
Erythromycin + 100 µM test | 40.21 ± 5.29 | 0.151 ± 0.021 |
Erythromycin + 150 µM test | 43.51 ± 7.75 | 0.183 ± 0.033 |
Erythromycin + 250 µM test | 88.58 ± 6.34* | 0.489 ± 0.095** |
Erythromycin + 500 µM test | 118.76 ± 10.72** | 0.672 ± 0.095** |
Erythromycin + 20 µM CsA | 65.18 ± 7.09* | 0.356 ± 0.060* |
Erythromycin + 200 µM Quin | 52.63 ± 7.79* | 0.286 ± 0.052* |
Erythromycin + 500 µM Vera | 43.56 ± 6.99 | 0.205 ± 0.039 |
Along with cornea, the BRB limits the permeability of various therapeutic agents into the eye. Retinal pigmented epithelium (RPE), situated between the retina and its choroidal blood supply, forms a diffusional barrier controlling access to subretinal space. Transport proteins present on the RPE regulate ionic composition and hydration of the subretinal space. Immunohistochemistry revealed that P-gp is localized both on the apical and basolateral sides of human RPE. Basolateral P-gp is attributed to the function of neural retina in clearing toxic and unwanted molecules from the subretinal space. Apical expression of P-gp appears to assist in the delivery of lipophilic substrates to subretinal space and engulfment by photoreceptors. Three RPE cell lines, ARPE-19, D-407 and h1RPE, are usually employed for in vitro studies. Screening of these three cell lines for P-gp expression revealed that D-407 is suitable for functional expression and drug transport screening. ARPE-19 cells lacked both molecular and functional expression of P-gp, whereas h1RPE lacked functional expression of P-gp. D-407 demonstrated both molecular and functional expression of P-gp. Also, P-gp was found to be expressed on the choroidal blood vessels. Further work is in progress to understand the role of P-gp in the choroid.
P-gp is also expressed in rabbit conjunctival epithelial cells. It is present on the apical side of the conjunctiva, conferring resistance to the absorption of cyclosporine A and other lipophilic drugs. Use of various P-gp inhibitors – such as verapamil, progesterone, cyclosporine A, rhodamine 123 and metabolic inhibitor 2,4-DNP – enhanced the uptake of the model drug propranolol in rabbit conjunctival epithelial cells. Yang et al concluded that P-gp can play a vital role in decreased absorption of various lipophilic drugs across the conjunctiva.
P-gp appears to play an important role in the outward transport of cytotoxic drugs and in the modulation of chloride channels which regulate and initiate osmotic cataract. Further, findings by Miyazawa et al revealed that P-gp might be induced by aldose reductase overexpression and osmotic stress. P-gp was found to maintain lenticular osmotic balance in sugar cataract. The role of P-gp in the drug disposition of various ocular agents is being further evaluated.
Mode of action and structure of P-gp
P-gp requires ATP for its function. There are two ATP binding sites, also known as nucleotide binding domains (NBD). Apart from NBD, there are two transmembrane domains incorporating six transmembrane domains at each site (a total of 12). A pictorial representation of P-gp is provided in Figure 17.2 .

MRP
Various classes of MRPs are expressed in the retina and other ocular tissues; the outer BRB expresses MRP. Functional characterization showed that the BRB is similar to the BBB. Functional studies using P-gp and MRP modulators revealed that outer BRB epithelium demonstrates similar pharmacological properties to endothelial BBB and expresses the same efflux transport systems. Also, Wilson et al demonstrated that retinoblastoma tumors express MRP1, 2 and 4. Immunohistochemistry revealed intense localization of these efflux pumps along with P-gp/BCRP in tumor tissue. The possible role of these transporters, either individually or collectively, in tumor therapy needs to be evaluated.
MRP is another major class of ABCC efflux transporter imparting drug resistance. The MRP family has nine members (MRP 1–9) and substrate specificity varies significantly among them. As shown in Figure 17.3 , RT-PCR performed on primary human corneal epithelial cells (based on protocol established in our laboratory ) revealed the expression of MRP1–7. Primary culture of human corneal epithelial cells was obtained from Cascade Biologics (Portland, OR) and RT-PCR performed based on an established protocol – primers used for the RT-PCR are presented in Table 17.2 . MRP is regarded as an organic anion transporter, but some MRP (MRP 4,5) are known to transport nucleoside and nucleotide analogs.

MRP1 (NM_004996) | A G G T G G A C C T G T T T C G T G A C C C T G T G A T C C A C C A G A A G G T |
MRP2 (NM_000392) | G C A G C G A T T T C T G A A A C A C A C A A C A G C C A C A A T G T T G G T C |
MRP3 (AF085691) | G C T T C T A T G C A G C C A C A T C A A C A A G G A C C C T G A A C A C C A G |
MRP4 (AY081219) | G G T T C C C C T T G G A A T C A T T T G A C A A A C A T G G C A C A G A T G G |
MRP5 (AB209454) | A C C C G T T G T T G C C A T C T T A G G C T T T G A C C C A G G C A T A C A T |
MRP6 (AF076622) | C A C C T G C T A A A C C G C T T C T C G A G C A T T G T T C T G A G C C A C A |
MRP7 (NM_033450) | G A A C G G C T G C T T A A C T T T G C A G G T A T A C A C A G G C C G C A T C |
The presence of MRP2 at the gene and protein level, as well as its functional expression in rabbit corneal epithelial cells, was established in 2007 in our laboratory. A possible role of MRP2 in conferring resistance to the absorption of erythromycin across rabbit corneal epithelial cells was reported. In an article by Karla et al, MRP2 expression on rabbit corneal epithelial cells at the gene and protein level was reported. [ 14 C]-erythromycin was selected as a model substrate and affinity to the transporter was measured employing a specific inhibitor, MK571. This work demonstrated apically polarized localization of the transporter in rabbit corneal epithelial cells. Also, uptake and transport of [ 14 C]-erythromycin was significantly elevated in the presence of MK571, suggesting that the transporters might act in conjunction towards outward directed transport.
Later studies by Karla et al revealed significant expression of MRP2 conferring drug resistance in human corneal epithelial cells. (As stated in our earlier publication:
“Significant elevation in uptake of model drugs [ 14 C]-erythromycin and [ 3 H]-cyclosporine-A in the presence of MK-571 indicated the functional activity of MRP2 in human corneal epithelium. Expression of ABCC2 may play a critical role in reducing the bioavailability of topically applied drugs. Cyclosporine-A is widely used as an immunosuppressive agent in corneal transplants and it is also indicated in the treatment of various corneal opacities. Erythromycin in various topical formulations has been used a drug of choice for treating minor corneal opacities. Though these compounds are substrates for P-gp, use of MK-571 reveals contribution of only MRP. A significant elevation in uptake of [ 14 C]-erythromycin in the presence of unlabeled cyclosporine-A was also observed. The results indicate a dose-dependent inhibition of ABCC2 in both rPCEC and SV40-HCEC cell lines. Further studies indicate similar MDR expression profiles in human and rabbit corneal epithelium, which validate rabbit as a suitable animal model for in-vivo MDR-mediated drug absorption studies. Transport studies across SV40-HCEC and rabbit cornea revealed a significant increase in drug concentration in receiver chamber in the presence of inhibitor. Enhanced permeability of [ 14 C]-erythromycin and [ 3 H]-cyclosporine-A from apical to basolateral side across SV40-HCEC in the presence of MK-571 reveals the important role of ABCC2 as a barrier in corneal drug influx. In human corneal epithelial cells apical to basolateral side transport of [ 3 H]-cyclosporine-A was elevated almost ~4-fold as compared to basal to apical transport, which increased ~2-fold in the presence of an inhibitor. Similar trend is observed for the passage of [ 14 C]-erythromycin where elevation in apical to basolateral transport was almost ~4-fold as compared to control whereas basal to apical transport was only ~1.5-fold higher than control. Acceleration in the basal to apical transport might be due to the presence of a homolog in MRP family.”)
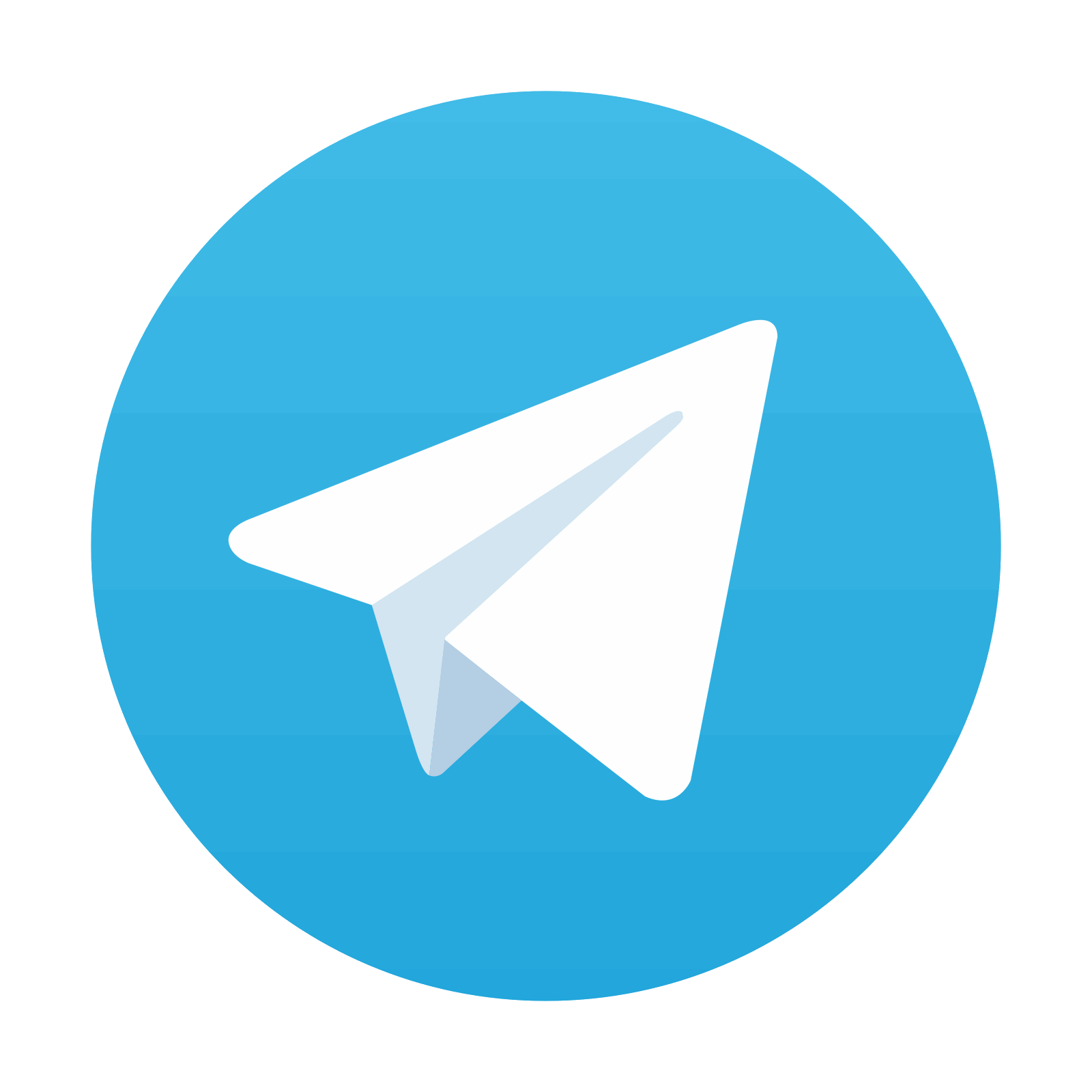
Stay updated, free articles. Join our Telegram channel

Full access? Get Clinical Tree
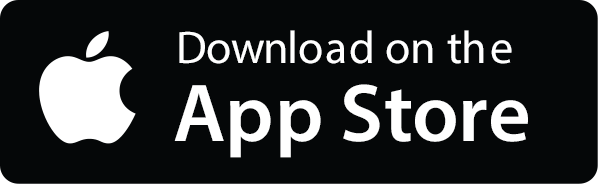
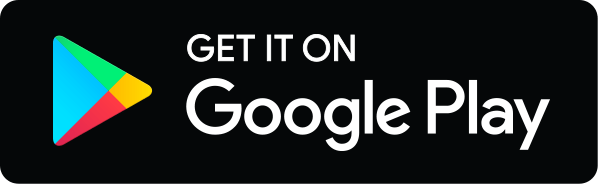
