Orbital disorders usually manifest with a unifying constellation of symptoms and signs that include proptosis, periocular swelling, blurred vision, and double vision. The presence of proptosis distinguishes diseases of the orbit from other neuro-ophthalmic conditions with similar symptomatology. In this chapter, relevant orbital anatomy and typical signs and symptoms encountered in orbital disease are put into clinical context; the advantages and disadvantages of various orbital imaging techniques are critically examined; and the orbital entities frequently encountered in neuro-ophthalmic practice are analyzed, including thyroid-associated ophthalmopathy, orbital inflammatory disorders, orbital tumors, and the sequelae of orbital infections and trauma. The pathogenesis, evaluation, and treatment of each orbital disorder are reviewed with particular emphasis on those conditions that can be vision- or life-threatening.
Orbital Anatomy
Familiarity with orbital anatomy is critical to understanding and recognizing the various conditions that are encountered. Several excellent texts and atlases are available along with excellent review articles with correlation to radiographic studies and congenital variations in orbital development. The orbit contains a wide variety of tissue types, including bone, periorbita, fibroadipose tissue, striated and smooth muscle, epithelial tissue of the lacrimal gland, the optic nerve, peripheral autonomic, sensory and motor nerves, the ciliary ganglion, arteries and veins, and cartilage in the trochlea of the superior oblique muscle. Although the orbit was traditionally thought not to contain lymphatics, current evidence suggests that there are lymphatics in the orbital fat, lacrimal gland, and optic nerve sheath.
A “functional” understanding of orbital anatomy and the interaction of all of these structures is grounded in the relationship of the various spaces within the 25–30 cc of the bony orbit. These spaces include the globe and the space immediately around it (Tenon’s space), the intraconal portion of the orbit (formed by the cone of the rectus muscles and containing the optic nerve), and the extraconal space ( Fig. 18.1 ). There is also a potential space between the bone and the periorbita which is a common site for orbital involvement by paranasal sinus infections and tumors. Further division into the superior and inferior extraconal spaces is helpful, as many conditions such as lymphoma and idiopathic orbital inflammatory syndrome (IOIS) have a predilection to develop in the superior and temporal orbit.

Bones of the Orbit
The orbit is bound by portions of seven different bones (frontal, sphenoidal, zygomatic, lacrimal, maxillary, palatine, and ethmoidal), and they contribute variably to form the walls of the orbit ( Fig. 18.2 , Table 18.1 ). The ethmoidal bone contains the medially located and thin lamina papyracea, which is vulnerable both to fractures and to the passage of infection from the paranasal sinuses. The posterior border of the orbit is the optic canal, and the anterior extent is the orbital septum, which originates in the periorbita and blends into the connective tissue of the upper and lower eyelids.

Orbital roof | Frontal bone Lesser wing of sphenoid |
Lateral wall | Greater wing of sphenoid bone Zygomatic bone |
Medial wall | Ethmoid bone Lacrimal bone Lesser wing of sphenoid Tip of maxilla |
Orbital floor | Maxilla Zygomatic Palatine |
There are three major bony openings into the orbit. The first is the optic canal through which passes the optic nerve, oculosympathetic nerves, and the ophthalmic artery. The second is the superior orbital fissure, formed by the greater and lesser wings of the sphenoid bone. The superior ophthalmic vein and the IIIrd, IVth, first (ophthalmic) division of the Vth, and VIth cranial nerves as well as some sympathetic nerves pass through the superior orbital fissure. Finally, the inferior orbital fissure is in the floor of the orbit, and through it passes the second (maxillary) branch of the Vth nerve, the inferior ophthalmic vein, heading into the pterygoid plexus, and branches of the sphenopalatine ganglion.
There are other important vascular foramina, including openings in the medial orbital wall for the anterior and posterior ethmoidal arteries. The ethmoidal arteries are branches of the ophthalmic artery and supply the dura in the anterior cranial fossa and the nose. The anterior ethmoidal nerve, a branch of the nasociliary nerve, also passes through the anterior ethmoidal canal and supplies sensation to the tip of the nose and upper lip. This sensory branch of the first division of the Vth cranial nerve is the reason why the tip of the nose is often involved in herpes zoster ophthalmicus (Hutchinson’s sign).
The bones of the orbit also contain important grooves and fossae. The infraorbital groove contains the infraorbital nerve, the terminal branch of the maxillary nerve, which exits the floor of the orbit through the infraorbital foramen to supply the sensation on the cheek and upper part of the jaw. The supraorbital nerve (a branch of the frontal nerve, which derives from the ophthalmic division of the Vth nerve) similarly reaches the skin of the forehead and frontal sinus through the supraorbital foramen or notch. The trochlear fossa is located superomedially 5 mm from the anterior orbital rim. Here the cartilaginous trochlea (through which the tendon of the superior oblique passes) is attached. The lacrimal fossa is located superotemporally in the frontal bone.
The lateral wall of the orbit is thickest anteriorly and is the strongest of the orbital walls. In the most anterior portion of the lateral wall, about 10–11 mm below the frontozygomatic suture, is the lateral orbital (Whitnall’s) tubercle. This bony tubercle serves as the point of attachment of the levator aponeurosis, the suspensory (Lockwood’s) ligament of the globe, the lateral palpebral ligament, and the check ligament of the lateral rectus muscle. The zygomatic canal is posterior to the tubercle, and through it passes the zygomatic nerve, a proximal branch of the maxillary nerve. It divides in the orbit into the zygomaticotemporal and zygomaticofacial nerves, which supply the skin on the lateral portion of the forehead and cheek.
Vascular Structures
The blood supply to the orbit derives from the ophthalmic artery, which is the first branch of the internal carotid artery after it pierces the dura of the cavernous sinus. The artery enters the orbit inferiorly through the optic canal and moves laterally between the optic nerve and lateral rectus, then superiorly and finally medially. Its terminal branches are the supratrochlear and dorsal nasal arteries. Some blood reaches the orbit through the facial, maxillary, and temporal branches of the external carotid artery. The branches of the ophthalmic artery (see Fig. 4.1 ), the structures they supply, and their important external carotid artery anastomoses are summarized in Table 18.2 .
Branch of Ophthalmic Artery | Structure(S) Supplied | Important Anastomoses With External Carotid Artery |
---|---|---|
Central retinal artery | Retina, optic nerve | |
Lateral posterior ciliary artery | Choroid, optic nerve (20 short branches) Ciliary muscle, iris, anterior choroid (2 long branches) | |
Lacrimal artery | Lacrimal gland | With transverse facial, zygomatic, and frontal branches of superficial temporal arteries With middle meningeal artery from maxillary artery |
Lateral muscular artery | Superior rectus, superior oblique, lateral rectus, and levator muscles Rectus muscles give off anterior ciliary arteries to anterior segment of eye | With orbital branches of infraorbital artery, a branch of maxillary artery |
Posterior ethmoid artery Supraorbital artery (together or separately) | Posterior ethmoid air cells Eyebrow, forehead Levator muscle | With lateral nasal artery from maxillary artery |
Medial posterior ciliary artery | See lateral posterior ciliary artery | |
Muscular artery | Inferior rectus, inferior oblique, and medial rectus muscles | |
To areolar tissue | Areolar tissue | |
Anterior ethmoid artery | Anterior ethmoid cells Frontal sinus Dura in anterior fossa Nose | With sphenopalatine artery from maxillary artery |
Medial, inferior medial, and superior medial palpebral arteries | Eyelids | |
Dorsal nasal (terminal) artery | Nose | With angular branch of facial artery |
Supratrochlear artery | Superomedial orbit |
The orbital veins are valveless. The superior ophthalmic vein and central retinal vein drain into the cavernous sinus. The inferior orbital vein drains into the pterygoid plexus. Anterior venous drainage can also occur into the facial veins via the angular and inferior ophthalmic veins.
Extraocular Muscles
The six extraocular muscles are striated with unique properties. The four rectus muscles (each about 3–4 cm long) originate from the annulus of Zinn at the orbital apex, which is contiguous with the dura surrounding the optic nerve and the periorbita. In addition to their insertion on the globe, secondary insertions in the connective tissue surrounding the muscle create “pulleys,” which function as secondary origins for rectus muscle action and facilitate compartmental contractions that increase the diversity of extraocular muscle functions. The levator palpebra also originates from the annulus of Zinn. The superior oblique originates just posterior to the annulus of Zinn and passes through the trochlea before turning posterolaterally to insert on the globe under and posterior to the insertion of the superior rectus. The inferior oblique originates from the bone just posterolateral to the nasolacrimal fossa and travels posterolaterally under the inferior rectus (attached to the inferior rectus by Lockwood’s ligament) to insert both into the connective tissue between it and the lateral rectus (its pulley or secondary origin ) and posteriorly on the globe in the region of the macula. The nerves innervating the rectus muscles enter them at the junction of the posterior and middle third of their bellies. These structures are discussed in more detail in Chapter 15 .
Periorbita and Septae
The periorbita is the periosteum of the orbital bones. It is only loosely adherent to the bones, except where it is fixed at the anterior orbital rim, the margins of fissures and canals, and the lacrimal crest. Posteriorly it is contiguous with the dura at the superior orbital fissure and optic nerve. The intraconal space is defined by the extraocular muscles and the intermuscular septa, which are denser anteriorly and may be absent posteriorly, although the anatomic distinction of the intraconal and extraconal spaces may be more complex, resulting from radial fibrovascular connective tissue septae, which also attach the muscles to the periorbita. These septae separate the orbital fat into lobules. The orbital septum is the anterior border of the orbit. It originates at the anterior orbital rim and superiorly fuses with the levator aponeurosis and inferiorly with the capsulopalpebral fascia.
Tenon’s capsule is a layer of delicate connective tissue attached to the globe near the limbus. The capsule envelopes the globe, has fine posterior attachments to it, and separates it from the orbital fat. The extraocular muscles pierce the capsule anterior to the equator of the globe and are enveloped in a sleevelike extension of the capsule. The muscles travel in the capsule for 7–10 mm, and the capsule gives off lateral extensions, forming the intermuscular membrane between the rectus muscles. Important attachments between the rectus muscles and the surrounding connective tissue may serve as the functional origin for each muscle.
Ciliary Ganglion
The ciliary ganglion (also discussed in Chapter 13 (see Fig. 13.3 )), lies in the posterior orbit between optic nerve and the lateral rectus muscle. The ganglion contains the synapses of the preganglionic parasympathetic fibers from the Edinger–Westphal nucleus of the IIIrd nerve and provides the postganglionic neurons destined for the ciliary body and pupillary sphincter. Preganglionic fibers reach the ciliary ganglion with the oculomotor branch to the inferior oblique muscle. Postganglionic fibers reach the anterior portion of the globe via short posterior ciliary nerves. Sensory fibers from the ophthalmic division of the Vth nerve and postganglionic sympathetic fibers pass through the ciliary ganglion on their way to the globe but do not synapse there.
Lacrimal Gland
The lacrimal gland sits in the lacrimal fossa and is separated from the globe by Tenon’s capsule and orbital fat. The most lateral portion of the levator aponeurosis separates the lacrimal gland into the smaller palpebral and larger orbital lobes. Portions of the palpebral lobe can be seen superolaterally upon eversion of the eyelid. The blood supply to the lacrimal gland derives from the lacrimal artery, a branch of the ophthalmic artery. Sensory innervation is from the ophthalmic division of the trigeminal nerve. Autonomic innervation is from parasympathetic fibers which synapse in the pterygopalatine ganglion and travel with the zygomatic branches of the maxillary division of the Vth nerve before reaching the lacrimal gland.
Neuro-Ophthalmology of Orbital Disease
Symptoms
Patients with orbital disease present with a variety of symptoms including vision loss, double vision, swelling, pain, ptosis, and proptosis. Most orbital disease is space occupying, and therefore the hallmark of orbital disease is proptosis or exophthalmos due to axial or forward displacement of the globe. Axial proptosis ( Fig. 18.3 ) is usually caused by disease within the muscles or muscle cone while nonaxial proptosis (displacement of the globe down, up, or sideways in addition to forward) is usually caused by extraconal lesions. Although many patients may notice prominence of one eye, others may have unrecognized moderate to severe proptosis. Lid retraction (sclera visible between the superior limbus and upper eyelid) is the hallmark of thyroid eye disease. Other causes of proptosis are generally not associated with lid retraction. In fact, superior orbital disease might also be associated with ptosis. Patients with orbital disease (particularly thyroid-associated ophthalmopathy (TAO) and IOIS) also present with eyelid soft tissue swelling or conjunctival injection. Patients with orbital fractures or bone loss in association with sinus disease (see Silent Sinus Syndrome ) can present with enophthalmos or sinking back of the globe (see Fig. 18.3 ). Here there may also be exaggeration of the superior sulcus, adding to the “sunken appearance.” Enophthalmos can also occur with processes associated with scarring of orbital tissue (e.g., metastatic breast cancer).


Double vision secondary to impaired eye movements is a frequent complaint. Double vision results from misalignment of the two eyes. Strabismus in this setting is usually noncomitant and results from a mechanical effect where the mass lesion or inflammatory process interferes with the movement of the eye directly or indirectly. Double vision can also result from extraocular muscle dysfunction by restricting the movement of the globe, as in TAO. Lastly, diplopia can also result from cranial nerve (IIIrd, IVth or VIth) dysfunction within the orbit or through invasion of the tumor into the cavernous sinus. Facial hypoesthesia can result from involvement of the Vth cranial nerve or its orbital branches.
Slowly expanding benign orbital neoplasms may not cause any visual symptoms. However, optic nerve tumors (gliomas and meningiomas) and large intraconal tumors frequently present with proptosis and decreased vision. Patients may describe a blur or recognize a loss of visual field (see Chapter 5 ). A peculiar variation of transient monocular vision loss occurs in patients with orbital mass lesions and is termed gaze-evoked amaurosis . Vision darkens as the eye is moved, presumably because the optic nerve or arterial blood supply is compressed by the mass lesion. Vision clears upon moving the eye back to the central position. However, gaze-evoked amaurosis has been described in association with a variety of other conditions including intracranial lesions, vitreopapillary traction syndromes, orbital fractures, sinus disease, TAO, sarcoid optic neuropathy, and elevated intracranial pressure. Decreased vision can also result from direct compression of the optic nerve or from indentation of the globe by a mass lesion, compression of the optic nerve by enlarged eye muscles in TAO, or an inflammatory perineuritis associated with idiopathic orbital inflammation. Rarely, individuals with shallow orbits or proptosis can develop spontaneous episodes in which the globe is trapped in front of the lids ( Fig. 18.4 ). This can be associated with blurred and double vision and is easily reduced by gently pushing the globe posteriorly.


Examination
The details of formal neuro-ophthalmic examination have been described in Chapter 2 . As with all patients, examination of patients with orbital disease begins with careful assessment of visual function including visual acuity, color vision, and visual fields. Beyond this, certain features of the examination of patients with orbital disease are specific.
Examination overview . The external examination begins by assessing the outward appearance of the eye, looking for asymmetry, globe position, swelling, and abnormal eyelid position. Since lid retraction is often associated with lid lag, the patient is asked to look down. The lid is then observed to see if it “hangs up” abnormally. Since mass lesions can often be felt around the eye, particularly if they primarily involve the lacrimal gland, the orbit and globe should be palpated. At the same time the globe is retrodisplaced into the orbit, and the presence of abnormal resistance or firmness, typical of most orbital disease, can be noted. Careful examination should be performed of the preauricular area and neck to look for evidence of abnormal adenopathy. The orbit can also be auscultated since bruits are present in some patients with arteriovenous malformations.
Proptosis can often be better appreciated if patients are asked to tilt their head backward. Then the examiner looks from below, comparing the position of the eyes in relation to the brow or anterior orbital rim. Formal measurements of proptosis can be accomplished with various devices. The Hertel exophthalmometer is a combination of a scale and mirror (see Fig. 2.36 ) and is placed on the patient’s lateral orbital rims. The examiner can then determine the relative position of each globe compared with the lateral orbital rim. Generally, the eyes are within 1–2 mm of each other, and any difference greater than that suggests either enophthalmos or exophthalmos. Serial measurements of proptosis with exophthalmometry are important in patients with active orbital disease to assess progression.
Optic disc findings . Initially, the optic nerve usually appears normal in patients presenting with orbital disease. Subsequently, chronic low-grade compression of the intraorbital optic nerve can lead to atrophy of the optic nerve and disc pallor. However, some patients with optic nerve tumors, and rarely patients with other orbital tumors, may present with optic nerve head swelling from compression of the anterior half of the optic nerve, leading to impaired axonal transport. Lesions around the orbital apex usually do not cause optic disc swelling. As a rule, optic disc swelling is not associated with intracanalicular or intracranial tumors unless there is associated mass effect, elevated intracranial pressure, and resulting papilledema. Disc swelling secondary to a chronic compressive lesion is sometimes further characterized by the presence of collateral or shunt vessels. These abnormal vessels develop to shunt blood flow from the retinal to choroidal circulation, bypassing the chronically obstructed central retinal vein. Collateral vessels are a common feature of optic nerve sheath meningiomas and are less commonly encountered with other causes of orbital disease.
Retinal findings . Retinal vascular changes are sometimes observed in patients with orbital disease. Venous engorgement or impending venous occlusion may be seen with optic nerve tumors but are very rare in patients with neoplastic or inflammatory disease of the orbit. The most common fundus abnormality in patients with orbital mass lesions is choroidal (chorioretinal) folding. These have also been described in patients with TAO, inflammatory diseases of the sclera and orbit, and mucoceles. The folding ( Fig. 18.5 ) is the result of extrinsic compression of the globe, leading to flattening of the sclera and subsequent wrinkling or folding of the retina and choroid. This creates a highly characteristic ophthalmoscopic finding of parallel, alternating lines or folds most often seen in the posterior pole. The finding is easily confirmed on optical coherence tomography, and the fluorescein angiographic pattern, first described by Norton, has a diagnostic appearance of alternating light and dark lines (see Fig. 18.5 ). The recognition of choroidal folds demands investigation for orbital disease, tumors, and globe compression, although they may also be secondary to hyperopia, hypotony, choroidal or retinal detachments, raised intracranial pressure, scleral buckles, subretinal neovascular membranes, and choroidal tumors. In many patients, and particularly in bilateral cases, choroidal folds are idiopathic.



Retinal arterial abnormalities are uncommon. Rarely, occlusions of the retinal arteries can occur in association with acute trauma and retrobulbar hemorrhage or secondary to angioinvasive forms of mucormycosis or aspergillus. Exudative retinal detachments can be seen in patients with IOIS.
Eye movement abnormalities . A complex pattern of eye movement impairment is the hallmark of orbital disease and may reflect both muscle restriction and cranial nerve dysfunction. The speed of ocular ductions should be noted. For instance, if an eye moves quickly but abruptly stops, a restrictive process or mass lesion is suggested. When the movement is slow for the entire excursion it suggests a cranial nerve palsy or myopathic process rather than a restrictive process. Neurogenic and mechanical eye movement limitation may also be distinguished by performing forced duction and forced generation testing (see Fig. 2.24 ). In general, patients with orbital tumors will have a mixed pattern of eye movement limitation that does not fit clearly into the pattern of a single cranial nerve. For instance, if a patient can neither elevate nor abduct an eye, it is more likely the result of mass effect or restrictive myopathy than a combined partial IIIrd and VIth nerve palsy.
Diagnostic Orbital Imaging
The diagnostic evaluation of orbital disease includes noninvasive imaging of the orbit either by echography (orbital ultrasound), computed tomography (CT), or magnetic resonance imaging (MRI). Each modality has advantages and disadvantages ( Table 18.3 ), but the techniques are often complementary. Imaging studies may be nondiagnostic except for the unique patterns seen with thyroid eye disease and paraorbital sinus disease. However, taken in conjunction with the history and examination, a tentative diagnosis can usually be established.
Modality | Advantages | Disadvantages |
---|---|---|
Echography | Instant results Readily available Echogenicity helps determine lesion type Images globe 30-degree test Images eye muscles well | Requires skilled operator Does not image posterior orbit |
CT | Available Affordable Evaluates bony changes Demonstrates calcification | Radiation exposure Inferior to MRI for soft tissue of the brain Poor imaging of cavernous sinus Lacks multiplanar reformatting capabilities of MRI |
MRI | Surpasses resolution of CT Exquisite soft-tissue detail Demonstrates optic nerve enhancement Images cavernous sinus and orbital apex well No radiation Possible to perform magnetic resonance angiography Images wood foreign body Uniquely images melanin and hemosiderin Unique signal characteristics can identify active inflammation or edema | Does not image bone Expensive (cost of unit, slower, more technician time) Thicker sections Unwanted nonspecific data (white matter changes) Longer scan time Claustrophobia is prohibitive for some patients Incompatible with magnetic materials or pacemakers |
Echography
Two types of analysis are used in orbital echography: amplitude-mode (A-scan) and brightness-mode (B-scan) ( Fig. 18.6 ). Many different orbital disorders can be diagnosed and followed echographically. An A-scan provides one-dimensional images and displays echoes as spikes. Elevated spike height implies increased echogenicity. The length between the transducer and the tissue is represented by the distance between spikes. An A-scan is commonly used to look for calcifications in the setting of optic nerve head drusen, to measure the size of lesions or of the extraocular muscles, and to determine the reflectivity (echogenicity) of a lesion or abnormal tissue. B-scan produces two-dimensional images of tissue sections, and images of the orbit are obtained along several different planes. An increase in the acoustic density of a tissue results in an increase in the brightness of the image. Fluid-filled structures (blood vessels, the globe, and cysts, for example) appear dark.

Echography is generally not used as the definitive study for orbital disease, although it has some particularly useful roles. One example is posterior scleritis, a form of IOIS, where the typical finding of fluid in sub-Tenon’s space can be seen on echography (see Fig. 18.6 ). Similarly, enlarged extraocular muscles of thyroid disease are easily demonstrated, and ultrasound can be used to quantify extraocular muscle thickness and reflectivity. Echography may be particularly useful in identifying patients with ocular misalignment due to occult thyroid eye disease, and color Doppler may be useful for assessing blood flow changes before and after treatment of thyroid ophthalmopathy. In children, echography along with color Doppler imaging may be helpful in identifying hemangiomas and dermoids. In the evaluation of mass lesions, the nature of the mass lesion and its relation to the globe can be characterized. In addition, the internal reflectivity of a lesion, suggestive of a vascular or cystic nature, may be ascertained. These properties allow use of echography to guide needle biopsies of orbital lesions.
Computed Tomography
Since bone, calcification, fat, and blood all have unique radiographic absorption patterns, CT is a very effective modality for orbital imaging ( Fig. 18.7 ). In fact, the contrast of the surrounding orbital fat allows for excellent imaging of the soft tissues (muscles and optic nerve) of the orbit by CT. Specific absorption patterns can be highlighted (“windows”) to emphasize bone, soft tissue, or blood.

CT images can be obtained in the axial, coronal, and sagittal planes and generally are taken at 3 mm intervals. If necessary, CT of the orbit can have a 1 mm slice interval with good resolution. Axial and coronal images are obtained directly, and sagittal images are usually obtained by computer reformatting. Coronal images are often not included in regular studies and should be requested when ordering orbital CT. Occasionally patients will be unable to position for coronal images and reformatted views are necessary. Bone windows and blood windows are usually viewed as well, and contrast is reserved for evaluation of mass lesions or to evaluate for intracranial extension of orbital disease (MRI is preferred; see the later discussion). Routine facial bone imaging is done with 5 mm axial and coronal slices, and when optic canal fractures are suspected, 1–3 mm coronal images should be obtained. In these cases, contrast is not utilized if bone and soft-tissue windows are viewed. Images are reformatted to produce three-dimensional, reconstructed views of the bony surface or deeper soft-tissue areas for presurgical evaluation of facial fractures and for evaluation of the skull in children with craniosynostoses and other skull deformities; they are not necessary in routine orbital imaging.
Magnetic Resonance Imaging
MRI is considered noninvasive because it does not utilize radiation. Instead, images are created when the nuclei of atoms found within the various tissues align with a powerful magnetic field and an applied radio frequency (RF) pulse. Most of the signals produced are from mobile protons of hydrogen atoms found within the water and lipids of tissues, and protons which are immobile (bone) give no appreciable signal. Signals are received by RF receiver coils and can be reconstructed in the axial, coronal, or sagittal planes.
Before the advent of surface coils (receiver coils which wrap around the patient’s face and eyes) and fat suppression techniques, CT was the preferred procedure for orbital imaging, even for the evaluation of soft tissue. However, MRI now offers many advantages over CT. MRI provides excellent images of the globe, orbit, and visual pathways and has become an invaluable tool in the diagnosis of pathologic lesions in the orbit and cavernous sinus. MRI provides excellent contrast resolution between soft tissues, and multiplanar imaging can be done without repositioning the patient ( Fig. 18.8 ). As with CT, contrast agents are used (gadolinium) to highlight normal anatomy, show breakdown of the normal blood–brain barrier, identify inflammatory and mass lesions, and demonstrate vascularity. MRI gives very little information about bones and fractures. CT should always be used when evaluating bony changes from tumors and in trauma. MRI scan times are considerably longer than those for CT. MRI is very sensitive to movement artifact (especially with surface coils), which can become significant when imaging the globe and orbit in uncooperative patients. MRI is contraindicated in patients with metallic objects in their eyes or head, cardiac pacemakers, or surgical clips that are not compatible with magnetic resonance.

Approach to the Diagnosis of Orbital Disease
Clinical History
The time course over which pain, proptosis, blurred vision, and double vision develop often helps distinguish neoplastic disease from infectious and inflammatory disease. Patients with infectious or non–thyroid-associated inflammatory disease of the orbit generally have an acute course with symptom development over hours or days. In contrast, neoplastic conditions, including primary optic nerve tumors, usually evolve insidiously. The exceptions are with malignant tumors such as adenoid cystic carcinoma of the lacrimal gland, some metastatic tumors, and secondary orbital tumors extending from the paranasal sinuses.
Age
The patient’s age group helps in the differential diagnosis of orbital disease. In the pediatric age group, congenital, developmental, and infectious lesions predominate. Fortunately, the majority of children with orbital disease have a benign process. Rhabdomyosarcoma is the most common malignant tumor of the orbit in children, while neuroblastoma and Ewing’s sarcoma may metastasize to the orbit. In less developed countries, retinoblastoma spreading to the orbit is a common cause of proptosis. Both idiopathic inflammatory disease of the orbit and TAO may occur in children but are less frequent entities. In adults, orbital cellulitis is less common, and thyroid eye disease is the most frequent cause of orbital dysfunction. Tumors of several different types may occur, with lymphoma being the most common. In older adults, metastatic and secondary orbital tumors and dural sinus fistulas increase in frequency.
General Physical Findings
General physical findings are particularly important in several instances. In suspected TAO, the physical examination might show vital signs suggesting hyperthyroidism or hypothyroidism. Other patients may have a goiter. Any patient with an orbital mass lesion should have a careful examination for other signs of malignancy such as adenopathy that might be associated with systemic lymphoma or metastatic disease.
Imaging
Imaging will help localize the process and will often define an etiology. Although TAO may be diagnosed clinically, imaging is confirmatory and helps in staging and in determining whether the patient is at risk for vision loss. Patients with idiopathic orbital inflammatory disease should be imaged (echography, CT, or MRI) to aid in the differential diagnosis and determine disease extent at presentation. The vast majority of patients with orbital tumors undergo neuroimaging with either CT or MRI. CT is the preferred modality in patients with a recent history of trauma and in patients in whom evaluation of bony changes is important. Both lytic lesions (malignant tumors) and hyperostosis (meningiomas or fibrous dysplasia) can be best demonstrated on CT. MRI has a distinct advantage over CT in terms of the details of soft tissue anatomy and abnormalities around the orbital apex and cavernous sinus. Both CT and MRI provide the necessary information to determine the relationship of an orbital mass lesion to the optic nerves, extraocular muscles, paranasal sinuses, and intracranial structures. Specific radiologic features for each of the various lesions are discussed in the following sections.
Neuro-Ophthalmic Examination
Although there is significant overlap in the examination findings of patients with each of the different forms of orbital disease, and most cases are best qualified by diagnostic imaging studies, there are certain neuro-ophthalmologic examination features that help establish specific diagnoses (see Examination ). In general, the examiner must focus attention on identifying orbital disease. Externally the presence of swelling, chemosis, and hyperemia of the skin suggests an infectious or inflammatory process. Preseptal and orbital cellulitis are commonly associated with significant enough swelling to close the eye and create pseudoptosis. IOIS characteristically causes redness and swelling. The swelling of TAO is usually less acute but can be significant. Rarely, metastatic tumors and vascular lesions (with recent bleeding) can present with acute swelling of the eyelid and periocular skin. The eyelid findings of lid retraction and lid lag are virtually diagnostic of TAO. Characteristic S-shaped deformity of the eyelid and nonaxial proptosis is present in patients with masses in and around the lacrimal gland ( Fig. 18.9 ).

A mixed pattern eye movement abnormality, with positive forced ductions, is generally found on examination. Slowly expanding neoplasms can cause significant proptosis without motility restriction (unless they primarily involve an extraocular muscle), as opposed to TAO and myositis, which tend to restrict eye movements. The presence of optic nerve dysfunction with orbital signs suggests a primary optic nerve tumor, TAO, idiopathic orbital inflammatory disease, or a tumor of the sphenoid bone such as a meningioma. Other mass lesions and inflammatory diseases infrequently cause optic neuropathy.
Thyroid-Associated Ophthalmopathy
TAO is a self-limited, autoimmune condition usually occurring in association with hyperthyroidism. Historically a number of other different terms have been used to label this condition, including Graves orbitopathy, Graves ophthalmopathy, thyroid eye disease, thyroid orbitopathy, endocrine exophthalmos, malignant exophthalmos, and infiltrative ophthalmopathy . It is the most common orbital disorder in adults. In two separate series by orbital specialists, TAO constituted 32–47% of orbital disorders. This percentage is different than the frequency of TAO in biopsy series, since it rarely requires tissue confirmation to establish this clinical diagnosis. TAO should be considered in the neuro-ophthalmic differential diagnosis of double vision, particularly vertical double vision, and in any patient with orbital signs and symptoms.
Endocrine Aspects of Graves Disease
A negative feedback loop between the hypothalamus–anterior pituitary gland and the thyroid gland modulates thyroid function (see Chapter 7 ). The thyroid gland is composed of numerous tightly packed follicles containing a rich proteinaceous colloid whose major component is thyroglobulin, the matrix protein in which thyroid hormones are synthesized. The hypothalamus secretes thyrotropin-releasing hormone (TRH), which in turn releases thyroid-stimulating hormone (TSH), a glycoprotein whose actions are mediated by cyclic adenosine monophosphate (cAMP). The TSH receptor on thyroid follicles is the site of action of autoantibodies which cause hyperthyroidism in many patients with Graves disease. These antibodies are believed to be produced in the thyroid gland by clonally restricted B cells. However, T cell–mediated cytokine responses and various environmentally triggered, immunogenetic events may also contribute to the development of Graves disease.
Hormone production in the thyroid gland is followed by secretion of iodothyronines (T3 and T4). Most thyroid hormone is secreted as T4 and converted peripherally to T3. T4 is highly bound to thyroxin-binding globulin (TBG) and thyroxin-binding prealbumin (TBPα). Thyrotoxicosis can result from overproduction of the hormone (true hyperthyroidism) or from inflammation-induced glandular hormone leakage. Graves disease or diffuse toxic goiter are used to describe a number of different conditions associated with elevated levels of thyroid hormones and associated ophthalmopathy and infiltrative dermatopathy.
Patients who develop hyperthyroidism present with a classic constellation of symptoms including nervousness, fatigue, weight loss, palpitations, heat intolerance, increased appetite, increased bowel movements, and sweating. Examination findings include tachycardia and a palpable goiter over which a thrill can often be felt. Laboratory abnormalities in Graves disease include elevated T3 and T4 levels, increased T3–T4 ratio, low TSH levels, thyrotropin-receptor antibodies, antithyroid antibodies, and abnormal T3 suppression tests. A low TSH level is a good indicator of thyroid dysfunction, with elevated free T4 levels and presence of antibodies against the TSH receptor adding significant sensitivity to the diagnostic evaluation.
Incidence and Relationship of Thyroid-Associated Opthalmopathy to Thyroid Disease
Bartley et al. reviewed the Mayo Clinic series within the Rochester Epidemiology project and identified 120 patients (103 women) with TAO in Olmsted County over a period of 15 years. The age-adjusted incidence rate for men was 3 cases per year per 100 000 people and for women was 16 cases per year per 100 000 people. A bimodal age peak occurred in both sexes in the 40s and 60s, with the peak in men occurring 5 years later than the peak in women. Endocrine dysfunction (most commonly Graves disease) developed in approximately 80–90% of patients found to have TAO and can manifest before, after, or concurrently with the ophthalmic findings. However, many patients (35%) are diagnosed with TAO longer than 6 months after the diagnosis of hyperthyroidism. In the least common scenario, TAO precedes the endocrine dysfunction by more than 6 months (7%). Of all patients with hyperthyroidism, 25–50% develop TAO at some time, although the incidence varies even more widely in different studies due to differences in how authors select patients and define TAO. The disease is perhaps less common in Asian people and black people from South Africa.
Risk Factors
The most potent risk factor for development of TAO is hyperthyroidism, although a subset of patients remains euthyroid. There may be other risk factors for the development of TAO, including age, gender, smoking, and treatment of the hyperthyroid state with radioactive iodine. Smoking is a risk factor for the development and progression of TAO, so patients with hyperthyroidism or early TAO should be urged to stop smoking.
TAO may also be more common and more severe in women with hyperthyroidism and in patients (particularly men) as they get older. Although it remains a controversial issue, it appears that treatment of the hyperthyroid state with radioactive iodine is associated with new onset of TAO and exacerbations of TAO, perhaps associated with a sudden increase in autoantibodies. Use of oral corticosteroids may reduce the risk of developing TAO and its progression to more severe stages in patients treated with radioactive iodine (see later discussion). More recently, studies have suggested that surgical thyroidectomy and use of statin medications may further reduce the risk of developing TAO.
Genetic Predisposition
Graves disease and TAO occur in a population which is likely to be genetically at risk for an environmentally triggered autoimmune disease. Studies of monozygotic and dizygotic twins suggest 79% of risk is genetic while 21% is due to environmental factors. An immunogenetic predisposition is likely multilocus based on the increased incidence in certain human leukocyte antigen (HLA) (DR, B8, DQ3, and DW) types and genetic mapping of loci for Graves disease in some patients to chromosome 14 in the area of the TSH receptor gene. Recently, single-nucleotide polymorphisms in specific genes, including IL-21 and the TSH receptor genes, have been identified and support their role in the genetic susceptibility to Graves disease.
Pathophysiology
There is extensive literature concerning the autoimmune mechanism of Graves disease and TAO. It appears to be mediated by both humoral and cell-mediated immunity, although the cause, precise immunopathology, and specific autoantigen that results in lymphocytic infiltration of the orbit are unknown. The relationship between Graves disease and TAO may simply be a closely related organ-specific autoimmune condition with an antigenic similarity between orbital and thyroid tissues. Graves disease is likely to be mediated by an autoimmune process directed against a portion of the thyrotropin receptor. These antibodies bind to TSH receptor sites, increase the gland’s activity, and may stimulate development of intracellular matrix proteins (glycosaminoglycans) in orbital fat and eye muscles. This leads to swelling of the orbital tissue and inflammation, then results in scarring. Activation of orbital fibroblasts seems to play a role, as many express high levels of the TSH receptor (TSHR) as well as insulin-like growth factor 1 receptor (IGF-1R). Orbital fibroblasts may therefore be activated by binding of TSHR antibodies or IGF-1R antibodies, leading to cell proliferation, secretion of hyaluronic acid, and expansion of the orbital tissues seen in TAO patients. Furthermore, bone marrow–derived fibrocytes that are capable of differentiating into fibroblasts are present in increased numbers in the circulation of patients with Graves disease and differentiate after entering the orbital tissue.
Previously, abnormal binding of the sera of patients with TAO to extraocular muscles in culture was demonstrated, leading to a vast literature on the potential role of direct muscle stimulation that is still possibly part of the disease mechanism, but seemingly is less important than fibroblast stimulation. It may be that the thyrotropin receptor or a 64 kDa membrane antigen, tropomodulin, provides a cross-reactive antigen to muscle as well as other orbital tissue, and the G2s (a portion of mitochondrial succinate dehydrogenase) protein is another candidate for the cross-reactive antigen in eye muscle. Other potential antigens include the 67 kDa flavoprotein (Fp) subunit of succinate dehydrogenase; calsequestrin, a 63 kDa calcium-binding protein; and collagen XIII, a connective tissue antigen. Wall and associates postulated a two-stage process in which first autoantibodies and CD4 (helper) T cells cross-react with eye muscle proteins leading to inflammation and swelling. In the second, more cytotoxic, stage there is inflammation and scarring of the muscles and orbital connective tissue secondary to released muscle membrane antigens during stage one. However, the antibodies to eye muscles may not be part of the pathogenesis but may develop subsequent to muscle damage by the cytotoxic T cells. While direct binding to muscle may still be involved, the increasingly recognized role of direct effects of TSHR antibodies on orbital adipose cells stimulating adipogenesis and increased orbital fat volume, and antibodies to IGF-1R stimulating hyaluronan production in fibrocytes, likely play a more critical role in the development of inflammation.
Pathology
The pathology of TAO reflects the described autoimmune inflammation. Infiltrating plasma cells, lymphocytes, and mast cells are seen. In addition, there is deposition of hydrophilic glycosaminoglycans and collagen that are part of the scarring process. In acute stages, changes are largely inflammatory. As the disease progresses there is more deposition of collagen and muscle degeneration. In the chronic inactive stage there is often fatty infiltration of the muscles.
Neuro-Ophthalmic Features of Thyroid-Associated Ophthalmopathy
The clinical presentation of TAO is highly variable and has been divided and grouped in many different ways. Most classifications are artificial and do not reflect the stepwise progression that often characterizes TAO.
Symptoms . These include periocular soft-tissue swelling ( Fig. 18.10 ), lid retraction, and bulging of the eyes. Some patients recognize and present with abnormal head postures or binocular double vision in upgaze or downgaze. Bartley and associates reviewed their 120 patients and noted that 36 complained of pain, 20 had double vision, 25 had tearing, 19 had photophobia, and 9 had blurred vision. Only 2 patients complained of decreased vision attributed to optic nerve dysfunction at presentation. Thus, vision loss secondary to optic neuropathy is usually recognized while the patient is being monitored.




While discomfort, corneal irritation, foreign body sensation, and “burning” are common complaints in patients with TAO, significant pain and pain on eye movements are unusual. In fact this is an important historical point to clarify in a patient with an acute orbital syndrome. The presence of significant pain makes TAO less likely and idiopathic orbital inflammation, infections, and tumors more likely. Similarly, the tempo of presentation is usually gradual as symptoms develop over weeks. Orbital symptoms developing over hours or even a few days are more consistent with other acute conditions, including infections and nonthyroid inflammatory conditions. The majority of patients with TAO will have bilateral signs and symptoms, although asymmetric and even unilateral disease does occur.
Eyelid signs . The eyelid manifestations of TAO are virtually pathognomonic. Lid retraction, present if the upper eyelid rests above the superior corneal limbus, is evident in most patients with TAO (see Fig. 18.10 ), although it is not always present ( Fig. 18.11 ). Eyelid retraction likely results from local adhesion of the levator muscle to fixed orbital tissue. Other postulated mechanisms include superior rectus overaction, secondary inferior rectus scarring, increased circulating sympathomimetics, and muscle hypertrophy. Lid retraction can also be seen in patients with dorsal midbrain dysfunction, ptosis of the contralateral eyelid, use of sympathomimetic drugs, aberrant regeneration of the third nerve, previous retinal detachment surgery, and Guillain–Barré syndrome (see Chapter 14 ). Lid lag (persistent elevation of the upper eyelid in downgaze; see Fig. 18.10 ) and lagophthalmos (inferior corneal exposure) are other common eyelid manifestations of TAO. Swelling and edema of the eyelids and periocular skin, dermatochalasis, and orbital fat herniation are also common (see Fig. 18.10 ). Periorbital swelling is typically worse in the morning after the patient sleeps, during which the eye is in a gravity-dependent position. Conjunctival chemosis and injection (particularly over the rectus muscle insertions) is often present (see Fig. 18.10 ). Swelling and redness in the area of the caruncle is a particularly important sign of active TAO (see Fig. 18.10 ). Occult TAO should be considered in the differential diagnosis of patients with dry eye.

Proptosis . Axial proptosis is recognized in about two-thirds of patients with TAO. On average the normal exophthalmometry measurement for each eye is 17 mm, and in most patients it is less than 22 mm. Any interocular difference greater than 2 mm is considered abnormal. The base used should be the same from examination to examination so that measurements from each visit can be compared.
Myopathy . The presentation of TAO-associated myopathy may be symptomatic (double vision or eye movement limitation) or asymptomatic (recognized on imaging studies). Patients may unknowingly and insidiously develop a chin-up posture because of ocular misalignment or restriction, with fusion occurring more easily in downgaze. Muscle dysfunction usually begins gradually, may be intermittent, and can occur without other evidence of significant inflammation. The inflammatory process has a peculiar predilection for the inferior and medial rectus muscles, and the restrictive myopathy results in characteristic hypertropia and esotropia, respectively ( Fig. 18.12 ). Involvement of the superior rectus is less frequent, and lateral rectus involvement is also less common.



Ocular ductions and alignment . Efforts have been made to measure eye movements in TAO, particularly for the purpose of classification within a grading system. Mourits and associates described a precise and reproducible method using a hand perimeter to measure the extent of ocular ductions, but this is not widely available and in our opinion does not aid in management of TAO-associated myopathy. Our preference is to grade eye movement limitations on a scale from −4 to +4 or in percentages (see Chapter 2 ) and to quantify ocular misalignments in all fields of gaze with prisms. “Diplopia visual fields” are also an effective method that can be used to “quantify” and follow patients with TAO-associated ocular misalignments. A Goldmann perimeter is used for this test; both eyes are left uncovered, and the head is positioned in the center. The patient is asked to follow the stimulus and report when it becomes double. A field of single binocular vision is then charted ( Fig. 18.13 ). Photographic documentation of ocular ductions may also be helpful in following patients (see Fig. 18.12 ).


Ocular cyclodeviations have also been described in TAO both spontaneously and as a complication of surgical treatment. Involvement of the inferior rectus (without obvious eye movement limitations) can closely mimic the appearance of a contralateral superior oblique palsy. Infiltrative TAO with restrictive myopathy is also associated with elevation of intraocular pressure on upgaze. The significance of the pressure elevation is uncertain and is rarely used to make a diagnosis. Other causes of extraocular muscle dysfunction in patients with TAO include ocular neuromyotonia and concurrent myasthenia gravis.
Optic neuropathy . Optic neuropathy is the most feared complication from TAO. The incidence of visual loss is between 2% and 9% of all patients with TAO. However, optic neuropathy occurs in up to 50% of patients with “severe” TAO requiring orbital decompression. Vision loss from optic nerve dysfunction requires immediate management. Combined medial and lateral decompressions are highly successful for reversing vision loss, while in some cases recurrent optic neuropathy can occur, requiring repeat surgery.
In one report, dysthyroid optic neuropathy occurred in 58 (8.6%) patients out of 675 with TAO. About 20% of patients presented unilaterally. At the time of presentation roughly half the patients had normal-appearing nerves, one-quarter showed pallor, and another quarter had swelling and hyperemia ( Fig. 18.14 ). About half the patients had acuity better than 20/40, but half were worse. In this and other studies, many patients were 20/20 but were still found to have other evidence of optic nerve dysfunction. Visual field defects were measured in two-thirds of patients, most commonly increased blind spots, nerve fiber defects, central or centrocecal defects, and generalized constriction. Many patients can have relatively quiet and inactive-appearing disease at the time of their presentation with optic neuropathy. Persistent elevation of intraocular pressure in patients with TAO may be associated with glaucomatous optic neuropathy, and this should be considered in the differential diagnosis of visual field defects in these patients.

Apical crowding of the optic nerve by the enlarged extraocular muscles is the main mechanism of vision loss ( Fig. 18.15 ). Vision loss can also be associated with stretch or tenting of the optic nerve from severe proptosis ( Fig. 18.16 ). Men with TAO seem to be at greater risk for development of optic neuropathy. Advancing age, diabetes, the amount of restrictive strabismus, and total volume of the extraocular muscles have all been reported as risk factors.





Imaging in Thyroid-Associated Ophthalmopathy
Noninvasive imaging of the orbit is the gold standard for diagnosis of TAO, using echography, CT, or MRI. The classic finding is enlargement of the extraocular muscle belly with relative sparing of the tendon ( Fig. 18.17 ), although a small percentage of patients will demonstrate involvement of the tendon on CT or MRI. Proptosis may also be recognized without extraocular muscle enlargement, presumably from increased swelling and volume of the intraorbital fat. In addition, CT and MRI frequently demonstrate proptosis, enlargement of the lacrimal gland, and eyelid soft-tissue swelling. Enlargement is most commonly seen in the inferior rectus and medial rectus muscles (see Figs. 18.15–18.17 ) and is usually symmetric, but isolated muscle involvement and primarily lateral rectus involvement are seen ( Fig. 18.18 ). CT and MRI, unlike echography, provide excellent images of the orbital apex and usually document enlarged muscles surrounding and in contact with the optic nerve in patients with optic neuropathy (see Fig. 18.15 ). Low-density areas may be noted in the muscle bellies and may represent collections of lymphocytes or glycosaminoglycan deposition. With chronic inactive disease, larger low-density lesions can be seen within the muscles, owing to fatty infiltration ( Fig. 18.19 ). Occasionally chronic medial rectus muscle enlargement can be associated with bone molding and bowing into the ethmoid sinus.




MRI of the orbit effectively images orbital soft tissues, including enlarged extraocular muscles and the orbital apex (see Figs. 18.17 and 18.19 ), and may have a distinct advantage over CT in assessing disease activity in addition to muscle size. One recommended MRI sequence is short tau inversion recovery (STIR), which highlights the extraocular muscles, and peak signal intensity from the most inflamed muscle correlates well with clinical activity of the disease by detecting high water content. This may be a method for following patients for radiographic evidence of persistent inflammation and may have specific use in monitoring response to antiinflammatory therapy. Use of newer MRI techniques such as diffusion-weighted imaging (DWI) and dynamic contrast-enhanced MRI take advantage of properties of inflamed tissues to provide additional potential methods to detect, in vivo, active disease within extraocular muscles. DWI may also be useful for detecting optic nerve abnormalities that correlate with functional visual measures in TAO patients. In addition to advanced MRI sequences, nuclear medicine scintography methods using nuclear-labeled specific inflammatory markers can detect TAO activity and may be useful in predicting which patients will respond to therapies such as orbital radiation.
Echography has proven valuable in the office diagnosis of TAO. Abnormal muscle size (A- and B-scan) and internal reflectivity (A-scan) can be documented ( Fig. 18.20 ). The ability to characterize muscle reflectivity in addition to enlargement is advantageous when trying to determine the etiology of an enlarged muscle. Echography was shown to be more sensitive than CT in detecting extraocular muscle enlargement and abnormal internal reflectivity secondary to separation of the muscle fibers. In addition, by demonstrating abnormal muscle reflectivity, echography may be particularly useful in the evaluation of patients with double vision and minimal external signs of TAO. Use of color Doppler ultrasound adds an ability to measure blood flow changes in the orbit that can be useful for monitoring response to therapy. However, the correlation between echography and MRI detection of TAO-related extraocular muscle thickening has varied between studies, and echography may not be as efficacious as other imaging modalities for diagnosing orbital tumors, suggesting a combination of methods may improve evaluation of suspected TAO.


Classification
Several different classification systems have been proposed for descriptive and clinical research studies of TAO. The NOSPECS classification system initially described by Werner was widely used in clinical research studies; however not all patients progress through the seven classes, and some have prominent features in the higher-numbered classes without some of the milder signs. The NOSPECS classification system has been criticized because (1) its poor characterization of the condition gives no indication of disease activity, marked underrepresentation of eyelid position, and overrepresentation of corneal problems; (2) it implies a continuous or step-ladder progression of disease; (3) parts of it are subjective; and (4) the gradings within several classes are poor. More recently, the European Group on Graves Orbitopathy (EUGOGO) proposed a more simplified system that divides patients into three groups ( Table 18.4 ), mild, moderate, and sight-threatening. Along with clinical activity score, these categories can be used to guide the types and urgency of treatment.
Classification | Findings |
---|---|
Mild |
|
Moderate |
|
Sight-threatening |
|
Classification of TAO must be combined with some measure of the clinical activity of the disease when trying to make decisions about treatment. The Clinical Activity Score (CAS) ( Box 18.1 ) has been proposed to assess the degree of active inflammation, which is critical when assessing different treatments in clinical trials. Patients can be considered to have mild, moderate, or severe periorbital swelling, with or without extraocular muscle dysfunction, and/or visual dysfunction secondary to compressive optic neuropathy or severe exposure. In this system patients with only mild or moderate symptoms are treated conservatively. Patients with more debilitating symptoms of swelling and eye muscle involvement and a high CAS score are considered for more aggressive treatment, including systemic corticosteroids and radiation therapy. Finally, patients with vision-threatening disease are treated emergently both medically (intravenous steroids) and with orbital decompression if necessary.
Spontaneous retrobulbar pain
Pain on eye movement
Redness of the eyelids
Redness of the conjunctiva
Swelling of the eyelids
Inflammation of the caruncle or plica
Conjunctival edema
A Clinical Activity Score (CAS) of ≥3/7 following signs and symptoms generally indicates active disease.
Treatment of Thyroid-Associated Ophtalmopathy
The treatment of TAO is complex, often requiring a multidisciplinary approach which includes a neuro-ophthalmologist, orbital specialist and surgeon, radiation therapist, and endocrinologist. Fortunately most patients do well, and in fact three-quarters of patients require no or only supportive therapy. Most patients do not develop sight-threatening complications of double vision or optic neuropathy. The disease usually runs a self-limited course of 18–36 months, and up to two-thirds of patients with TAO improve spontaneously.
Appropriate management is dependent on the accurate assessment of clinical findings at the time of presentation, including careful evaluation of visual function, eye movements, and orbital imaging. In patients with lid retraction and proptosis only, reassurance, ocular lubrication when exposure or ocular discomfort is present, elevation of the head of the bed to reduce dependency edema, reduction of risk factors by cessation of smoking, and maintenance of a euthyroid state are often the only treatments recommended. It is important to emphasize to the patient that the condition tends to remit, and that ultimately cosmetic aspects of the disease can be addressed.
Infiltrative disease with myopathy and increased orbital congestion are indications for more aggressive therapy. Patients with double vision and no congestive signs are often treated conservatively with prisms or patching as needed. The two major options for patients who require more aggressive therapy are systemic corticosteroids and orbital radiation, although these treatments are not always successful.
Steroids and other medical therapies . As signs and symptoms of severe congestive orbitopathy increase, treatment should first consist of oral (1–1.5 mg/kg of prednisone daily for 4–6 weeks followed by an oral taper) or intravenous corticosteroids (a single dose of 0.5 g methylprednisolone per week for 6 weeks followed by 0.25 g per week for 6 weeks or 1 g methylprednisolone for 3–5 days followed by an oral prednisone taper). These doses are arbitrary and are based on limited data. A review of various clinical case series suggests that intravenous steroids are slightly more effective and may be associated with fewer side-effects, About two-thirds of patients have reduction in their symptoms and swelling in about 1 week. Although symptoms of active inflammation improve on steroids, only about one-third of patients show improvement of the eye movements. Intravenous steroids can be given in simple pulses or followed by oral steroids, which then can be slowly tapered by 10 mg per daily dose each week. If symptoms worsen, reinstituting the original successful dose and radiation therapy should be considered; however, long-term use of recurrent doses of steroids is often associated with significant side-effects and should be avoided. In patients with more severe orbitopathy or significant vision loss from compressive optic neuropathy, intravenous methylprednisolone is favored and has been shown to be effective.
Other medical therapies, including plasmapheresis, intravenous immunoglobulin, azathioprine, and rituximab, have been shown to be effective but have not assumed primary roles in the medical management of TAO. B cell suppression with rituximab appears particularly promising, but randomized controlled clinical trials are needed to demonstrate efficacy. More targeted immunotherapy, with antibodies to specific pathogenic antigens such as the TSH receptor and IGF-1R, as well as IL-6 receptor antibodies, have shown initial promise but remain in the early stages of investigation. Recently a randomized placebo-controlled trial found that selenium improves quality of life and slows progression of orbitopathy in patients with mild disease, but serum selenium levels were not measured, and further studies will likely be needed before this becomes standard therapy.
As stated earlier, treatment of hyperthyroidism with radioactive iodine is associated with worsening of TAO. This deterioration may be lessened by the use of oral corticosteroids before, during, and after radioactive iodine treatment. Bartalena and associates found that 15% of patients treated with radioactive iodine alone developed or had worsening of TAO. In contrast, none of the patients treated with radioactive iodine and prednisone had progression of disease. Thus, prednisone is recommended in all patients with active TAO undergoing treatment with radioactive iodine for hyperthyroidism. In addition, a prolonged period of hypothyroidism after treatment should also be avoided. Steroids are not necessary in patients undergoing radioactive iodine treatment with no or inactive TAO. Some have recommended radioactive iodine treatment be avoided in patients with active or severe TAO and that medical therapy with antithyroid drugs be used instead.
Radiation therapy . Radiotherapy for TAO has been reported to be effective in 50–94% of patients, often taking several weeks (often with transient worsening requiring steroids) to take effect. Most patients considered for radiotherapy have congestive eye signs with diplopia or optic neuropathy and have continued activity despite a trial of steroids. Several series have demonstrated favorable responses in patients treated with radiation with or without steroids. However, Gorman and associates reported no long-term benefit of radiation in a prospective blinded study in which one orbit was radiated and the other left untreated. This study has been criticized, because some treated patients may have been in inactive phases of their disease. Other evidence suggests that radiation should not be considered as a last resort but more appropriately should be used early in the course of the disease in conjunction with steroid therapy in clinically active patients.
The technique generally involves use of 1500–2000 cGy in divided fractions over 10 days, and a total dose of 20 Gy was also shown to be more effective when administered in conjunction with glucocorticoid therapy. Care is taken to irradiate only the posterior two-thirds of the orbit, avoiding the globe. Radiation may be more effective than steroids in the treatment of optic neuropathy. In one study, only 1 of 29 patients treated with radiation for optic neuropathy required subsequent surgical decompression, while 6 of 16 treated with steroids needed surgery. Improvement in soft-tissue swelling almost always occurs. Careful, quantitative measurements of eye movements often demonstrate some improvement, although this does not correlate with improvement in double vision. Rootman found that only 14% of his patients had recurrence of symptoms after radiotherapy. Complications of radiation therapy are rare, although radiation retinopathy has been reported, and a slight increase in risk of secondary malignancy has been suggested. The use of radiation therapy for TAO remains controversial and will remain so until a prospective trial is performed, but most metaanalyses and reviews suggest a beneficial effect in patients with active orbital congestion, particularly with concomitant use of steroids.
Surgery . Surgical treatment of TAO is indicated in two separate situations. The first is orbital decompression for sight-threatening disease secondary to severe corneal exposure or optic neuropathy from orbital apex crowding, particularly after a trial of steroids has failed. The second is the use of orbital decompression, eye muscle surgery, and eyelid surgery in a patient with quiescent disease who has double vision, exposure, or a significant cosmetic problem. Orbital decompression should not be used in the acute treatment of proptosis and swelling from TAO unless there is sight-threatening disease. Mechanical decompression of the orbit has been described in several large series and is safe and effective in improving visual function in patients with apex crowding secondary to TAO-associated myopathy. Removal of the orbital floor and medial wall ( Fig. 18.21 ) using various approaches is the most common surgery performed. Comparison of the coronal method to the inferomedial and the inferomedial plus lateral approach found each to be equally effective in treating vision loss. Endoscopic approaches appear to be equally effective and avoid external scarring.


Regardless of the technique employed, successful treatment requires decompression of the orbital apex through removal of the apical portions of the orbital walls and opening or removing the periorbita (see Fig. 18.21 ). Visual improvement should be prompt, and if vision fails to improve, the decompression was likely incomplete at the orbital apex. Severe vision loss preoperatively is associated with a worse prognosis postoperatively, as only 4 of 7 patients with less than 20/200 vision preoperatively attained visual acuity of 20/40 postoperatively in one series. Significant improvement in nearly all parameters of the optic neuropathy including visual fields and optic disc swelling occurs in most patients. Patients also notice improvement in their congestive symptoms since the orbit is no longer under pressure. Most patients who undergo orbital decompression do so within 1 year from the onset of eye symptoms. Double vision is present postoperatively in 33–63% of patients and may be worsened because of associated postoperative scarring and loss of the bony structure supporting the position of the EOMs. Up to 70% of patients require one or two eye muscle surgeries to correct their double vision after orbital decompression. Interestingly, approximately 5% of patients experience improvement in their double vision after orbital decompression.
Other indications for orbital decompression include severe orbital inflammation, reduction of proptosis before eye muscle surgery, exposure, and steroid intolerance. After the disease becomes quiescent, orbital decompression is performed more commonly for cosmetic reasons. Reductions in proptosis of 4–5 mm may be achieved with good patient satisfaction. Further surgery may be performed for eyelid (many patients experience worsening of lid retraction) and eye muscle abnormalities. In one series of 15 patients with no preoperative double vision who underwent transantral orbital decompression primarily for cosmetic reasons, 11 (73%) developed double vision postoperatively, although no patient was dissatisfied with their surgical result. A modified approach to orbital decompression for cosmetic purposes involves only removing orbital fat without bone removal.
Eye muscle surgery for dysthyroid myopathy is often required to correct double vision. The strabismus may be more difficult to repair in the setting of previous orbital decompression when a large-angle esotropia is present. The goal is to establish a field of single vision that is as large as possible, including in primary position and downgaze. Rootman found that 9% of his patients ultimately required eye muscle surgery. The most common misalignment is a combined esotropia and hypotropia, for which inferior rectus recession and/or medial rectus recessions are required either unilaterally or bilaterally ( Fig. 18.22 ). Resections should be avoided, although lateral rectus resections can be successfully used for persistent esotropia after medial rectus recessions. Adjustable sutures can be particularly helpful, resulting in good surgical outcomes in 96% of patients (65% with no diplopia without correction, and 31% requiring less than 10 prism diopter correction to resolve diplopia postoperatively) in one study, and we advocate their use when possible. A relaxed muscle technique has been described in which the detached muscle is sutured to the sclera at the point where the relaxed muscle rests freely on the globe, rotated away from the muscle being recessed. Surgery should not be performed until measurements have been stable for 6 months and a similar time has elapsed since the last intervention (radiation or decompression). Intraoperative decision-making is always based on results of forced duction testing, and the surgeon should be prepared to alter the plan, particularly if the superior rectus is found to be tight. In cases of severe scarring, surgery and exposure of the muscle may be difficult. The initial surgical goal for hypertropias should be a slight undercorrection, as a drift toward overcorrection occurs postoperatively. About two-thirds of patients achieve a successful result of improved eye movements ( Fig. 18.23 ) with single vision in the primary position and downgaze after a single operation. However, undercorrections as well as late overcorrections (particularly for inferior rectus recessions) are common. It is not uncommon for patients to require multiple procedures.





Eyelid surgery is reserved as the last procedure performed (after decompression and muscle surgery), because lower eyelid retraction is a common complication. Surgery usually entails lowering the upper eyelid and raising the lower eyelid. Procedures on the lower eyelid usually require detaching the capsulopalpebral fascia from the tarsus and inserting some type of spacer (sclera, hard palate, cartilage, or fascia). The upper eyelid can be lowered by Müllerectomy or levator recession, and in many instances, because of chronic swelling and fat herniation, this is combined with blepharoplasty.
Course and outcome . TAO usually runs a course of 18–36 months. There is some evidence that clinical activity correlates with thyroid-stimulating immunoglobulin (TSI) levels, although its ability to detect responses to treatment is unclear. Symptoms may vary on a diurnal basis depending on the amount of venous and lymphatic drainage. Careful monitoring of the patient’s endocrine status must be performed, and we believe it is inadequate for the ophthalmologist or neurologist to simply order “thyroid function tests” and not involve the expertise of an endocrinologist.
Bartley and associates followed their cohort of 120 patients for a median time of 10 years. Only two eyes had persistent vision loss from optic neuropathy (20/30 and 20/60). Seven patients had intermittent double vision, and two other patients had constant double vision which was correctable by prisms. Twenty percent of patients underwent at least one surgical procedure. Many patients complained of persistent ocular discomfort, which in most cases was the result of dry eyes. Although many patients (one-third) were unhappy with their appearance, with successful treatment functional impairment seems to be uncommon.
Pediatric thyroid eye disease . Pediatric Graves disease or hyperthyroidism is not uncommon and rarely may be associated with development of TAO. Medical therapy with methimazole is the first-line therapy, with propylthiouracil avoided due to liver toxicity, but remission rates are low and radioiodine therapy or thyroidectomy are frequently needed. It is unclear how treatment affects development of ophthalmopathy in children. Orbital signs and symptoms occur in about one-third to one-half of patients, with 47.5% of pediatric patients with Graves disease exhibiting signs of ophthalmopathy in one long-term follow-up study. Proptosis may be dramatic. The incidence may be higher in countries where teenage smoking is more common. Fortunately restrictive myopathy, exposure, and optic neuropathy are rare in children. Echography, CT, and MRI have all demonstrated enlargement of extraocular muscles in children, although motility for the most part remains normal. The majority of children can be treated conservatively, and orbital fat decompression has been reported to be helpful in reducing proptosis.
Drug-induced thyroid-associated ophthalmopathy–like disease . Patients treated for metastatic melanoma with ipilimumab, a humanized monoclonal antibody that blocks cytotoxic T-lymphocyte antigen 4 (CTLA4), preventing T-cell suppression, can develop proptosis and extraocular muscle thickening with the same clinical and radiographic features seen in TAO patients. Combined with observations that genetic mutations in the CTLA4 are linked to Graves disease, it has been suggested that suppression of CTLA4 function may be involved in the pathogenesis of TAO. Antiprogrammed death-1 monoclonal antibody (nivolumab) therapy for metastatic melanoma has also been found to induce autoimmune thyroid disease ; thus baseline eye examination and periodic monitoring of patients initiating these therapies may be warranted.
Idiopathic Orbital Inflammatory Syndrome (Orbital Pseudotumor)
Patients with IOIS are typified by their acute onset of proptosis and eyelid swelling associated with pain and double vision. There is usually conjunctival swelling (chemosis) and injection ( Fig. 18.24 ). Eyelid erythema similar to that seen with cellulitis is often present ( Fig. 18.25 ), and there may be a violaceous injection over the muscle insertions. IOIS is usually distinguished from other causes of orbital disease (except infections and hemorrhages) by the rapidity of onset and discomfort. Symptoms result from a non–thyroid-related, noninfectious inflammation of the orbit characterized by polymorphous orbital infiltration of lymphocytes and plasma cells with varying amounts of fibrosis and mass effect.


IOIS is an umbrella term that applies to a heterogeneous group of presentations. It may be further subclassified according to the location of the inflammation within the orbit. In this section, the pathogenesis and pathology of IOIS are discussed, followed by a review of its classification, imaging, and treatment.
Pathogenesis
The pathogenesis of IOIS is unknown, and theories include viral, allergic, and autoimmune mechanisms. Based on the lymphocytic nature of the infiltration, it is assumed to be an immune-mediated condition mediated by both B and T lymphocytes. Whether it is a genetically predetermined autoimmune condition versus an environmentally triggered immune event is unclear. Other theories include aberrant wound healing and infection. Several observations suggest an autoimmune inflammatory condition, although the significance of each is unclear. These include identification of antibodies to eye muscle surface antigens in patients with IOIS; reports of patients who had upper respiratory tract infections before developing IOIS; and the occasional association of IOIS with similar nonspecific inflammation of the paranasal sinuses. High levels of specific cytokines, including interferon-gamma and interleukin-12, in orbital biopsy samples from patients with IOIS suggests possible inflammatory signaling pathways involved in triggering or maintaining inflammation.
Recently IgG4-related disease has been identified as a specific subset of inflammatory diseases, including IOIS. IgG4-related disease is a sclerosing inflammatory disease, marked by infiltration of lymphocytes and plasma cells, first described as systemic disease with autoimmune pancreatitis and subsequently recognized in most other organs. In the orbit, IgG4-related inflammation can affect almost any tissue, including lacrimal gland, optic nerve sheath, extraocular muscle, sclera, trigeminal nerve, and orbital fat, contributing to the full spectrum seen in IOIS. While serum IgG levels may be elevated, they are not diagnostic, with a high IgG4/IgG ratio detected on histologic evaluation within biopsy or resected tissue specimens typically required to establish the diagnosis. A high IgG4 ratio has been identified in anywhere from 19–53% of IOIS patients examined, and many of these cases have other systemic manifestations of inflammatory disease. Thus, systemic evaluation should be considered in patients with IgG4-related IOIS.
Differential Diagnosis
IOIS-like presentations may occur with orbital inflammation due to systemic inflammatory conditions or vasculitis, including temporal arteritis, Wegener’s granulomatosis, polyarteritis nodosa, systemic lupus erythematosus, gout, psoriatic and rheumatoid arthritis, Behçet disease, syphilis, tuberculosis, sarcoidosis, ulcerative colitis, and Crohn disease. Contrary to older theories concerning IOIS, it is no longer believed to be related to orbital reactive lymphoid hyperplasia (pseudolymphoma) or a lymphoid tumor. There is no apparent potential for malignant transformation.
Pathology
Histologically, IOIS is characterized by lymphocyte, plasma cell, macrophage, and polymorphonuclear cell infiltration associated with a fibrovascular stroma. This inflammation eventually leads to fibrosis, but there should be no vasculitic features. More chronic forms of IOIS are characterized by insidious development of fibrosis or desmoplasia leading to an apparent soft-tissue mass, without an acute phase of cellular infiltration. Although there is significant overlap, some have suggested subgrouping IOIS pathologically into lymphoid, granulomatous, and sclerosing varieties. However, usually a more clinical and anatomic classification is preferred (see later discussion).
Prevalence
IOIS is a relatively uncommon condition. In clinical series of patients presenting with orbital disease, patients with IOIS comprise between 6% and 8%. It can occur at any age, with most patients presenting in middle life. There is no sex or race predilection.
Classification
Several different classifications schemes for IOIS have been described. Rootman and Nugent proposed a system which groups patients depending on location of orbital involvement. Categories include anterior, diffuse, apical, lacrimal gland, and myositis. This system is helpful in understanding the highly variable clinical presentations of IOIS. For the purposes of neuro-ophthalmic differential diagnosis, we find another related system helpful and consider patients with IOIS in six different categories: posterior scleritis, diffuse, myositis, dacryoadenitis, perineuritis, and sclerosing inflammation. The new additional classification of some patients with IgG4-related disease is also important for predicting clinical course and to inform treatment decisions. This classification system allows for separating patients into categories that are unique with regard to their differential diagnosis, evaluation, and treatment. The combined use of histopathologic and localization-based classification may provide additional reliability for research studies in IOIS.
Posterior scleritis . These patients have inflammation focused on the eye wall and surrounding Tenon’s capsule. Patients develop pain, proptosis, ptosis, lid swelling, conjunctival injection and chemosis, and decreased vision. It occurs in all age groups but seems to be a common form of IOIS in children, where it can present bilaterally or unilaterally. Imaging reveals anterior ragged orbital inflammation, which involves the globe and is associated with retinochoroidal thickening. Echography may demonstrate sclerotenonitis with edema or widening of Tenon’s space (see Fig. 18.6 ). This effusion at the neuroocular junction and doubling of the optic nerve shadow creates a specific echographic sign called the T-sign ( Fig. 18.26 ). This form of IOIS may be characterized by ophthalmoscopic abnormalities including choroidal folds, exudative retinal detachment ( Fig. 18.27 ), papillitis, and uveitis. Fluorescein angiographic abnormalities may include patchy choroidal infiltrates, exudative retinal detachments, and macular edema. Treatment is with oral steroids, and their effect is usually prompt and dramatic. Glaucoma may complicate posterior scleritis. Mechanisms include choroidal effusion with anterior rotation of the lens–iris diaphragm or uveitic glaucoma. While posterior scleritis is typically part of IOIS, rare cases have been associated with inflammatory bowel disease.


Diffuse inflammation . In the diffuse presentation, inflammation involves the entire orbit. Clinically, patients have proptosis, eyelid swelling, ptosis, and occasionally extraocular muscle involvement and double vision. Papillitis and exudative retinal detachments may be present. On imaging studies, the entire orbit is involved by the irregular infiltrate that often obscures normal orbital anatomy ( Fig. 18.28 ). When orbital inflammation primarily involves the orbital apex (often subcategorized as “posterior IOIS”), there may be a disproportionate amount of visual and eye movement dysfunction, and intracranial extension can occur. Pupillary defects (internal ophthalmoplegia) have also been described with posterior orbital involvement, presumably due to disruption of the ciliary ganglion or third nerve.


Myositis . Patients with myositis have a clinical presentation dominated by symptoms of double vision and pain on eye movements. Ptosis and eyelid swelling are common, and chemosis and conjunctival injection in the area overlying the inflamed muscle are prominent (see Fig. 18.25 ).
Clinical distinction from TAO can usually be made based on the rapid development of myositis and its characteristic pain, eyelid findings, and diffuse involvement of the muscle and tendon on CT or MRI ( Fig. 18.29 ; see also Table 18.5 and Box 18.2 as well as the discussion of other causes of enlarged eye muscles later in the chapter). Orbital echography with A- and B-scan ( Fig. 18.30 ) can be used to identify muscle enlargement with tendon involvement and low reflectivity consistent with edema. The most common muscles involved in a series by Rootman et al. were the superior rectus–levator complex and the medial rectus, but any or multiple muscles may be involved. Involvement of the superior oblique with point tenderness over the superomedial aspect of the orbit has been described.

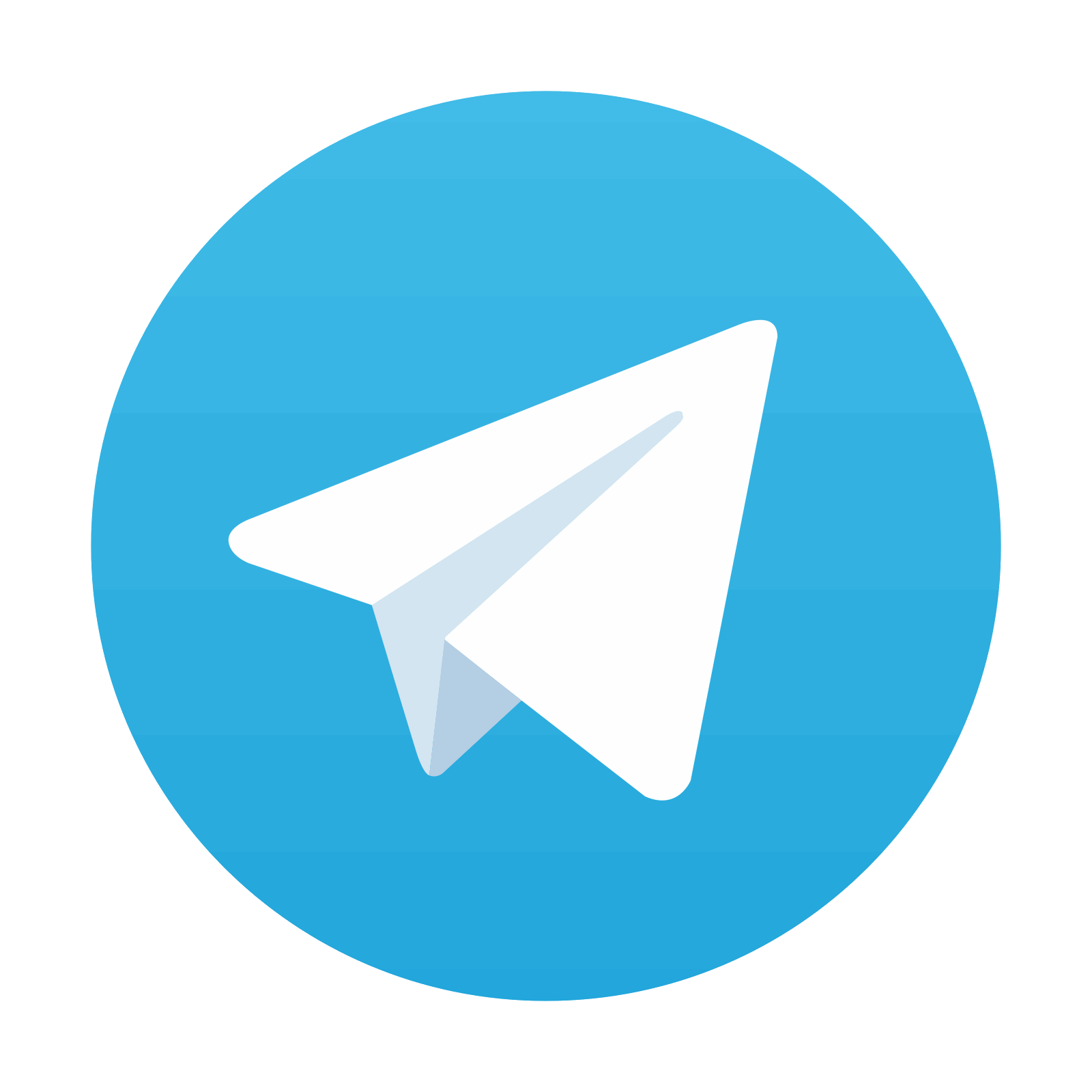
Stay updated, free articles. Join our Telegram channel

Full access? Get Clinical Tree
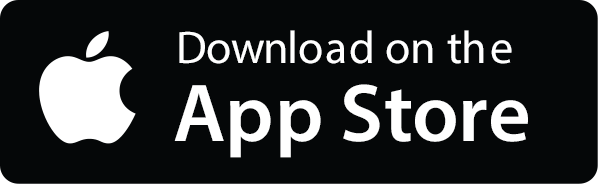
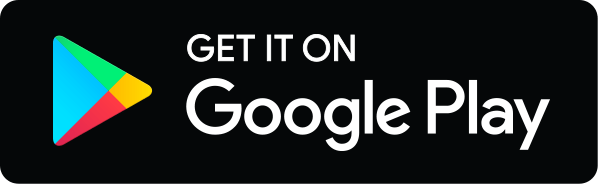
