Glaucoma is an optic neuropathy with characteristic optic nerve appearance and visual field loss for which elevated intraocular pressure (IOP) is one of the main risk factors. This characteristic optic nerve appearance results from structural glaucomatous changes which usually precede functional deterioration (visual field loss). Therefore, improvement of the diagnostic methods of structural abnormality and change can result in earlier diagnosis of the disease.
Structural evaluations of the optic nerve head (ONH) and retina are key to the diagnosis and follow-up of glaucoma patients. Imaging tests complement slit-lamp biomicroscopy exam and stereo photos of ONHs. Ophthalmoscopy and even sequential stereo photos are dependent on the expertise and skills of the observer. High intra-observer and inter-observer variability has been demonstrated in several studies. The goal is to diagnose the disease or its progression as early as possible, to increase the probability of preventing visual loss.
There are three predominant imaging technologies currently in use for the diagnosis and evaluation of glaucoma in Europe and the U.S. These devices are: confocal scanning laser ophthalmoscopy (CSLO) (the most common commercial application is known as Heidelberg retina tomography (HRT)); optical coherence tomography (OCT); and scanning laser polarimetry (SLP), whose commercial application is known as GDX. Scans of the ONH, retinal nerve fiber layer (RNFL) and of the macula have been studied for their relevance to glaucoma. Not all imaging technologies have the capability of imaging all three intraocular structures. The ONH can be scanned with HRT and OCT. The nerve fiber layer can be scanned with GDX and OCT, and the macula can be scanned with OCT. All these technologies work differently and have their own strengths and limitations as well as different measures of reliability. Full comprehension of all of these points will allow the clinician to accurately interpret the data obtained by each one of the imaging tests, as well as their correlation with one another. The ultimate goal is to improve the early diagnosis of glaucoma and detection of glaucomatous progression.
CONFOCAL SCANNING LASER OPHTHALMOSCOPY (CSLO)
HEIDELBERG RETINA TOMOGRAPHY (HRT)
Confocal scanning laser ophthalmoscopy is the imaging technology and HRT (Heidelberg Engineering, Heidelberg, Germany) is the major commercially available instrument that utilizes this imaging system to study the eye ( Fig. 14-1 ). Heidelberg retina tomography has three generations: HRT, HRT II and HRT 3.

Confocal scanning laser ophthalmoscopy is capable of obtaining three-dimensional images of the optic disc by acquiring high-resolution images, both perpendicular to the optic axis ( x – and y -axis) and along the optic axis ( z -axis) ( Fig. 14-2 ). It is based on the principle of spot illumination and spot detection. Conjugated pinholes are placed in front of the light source and light detector and allow only light originating from a determined focal plane to reach the detector. Sequential sections are obtained by moving the depth of the focal plane through the whole depth of the tissue being studied; in this case, the optic nerve. The focal plane depth is adjusted by shifting the confocal aperture or pinhole ( Fig. 14-3 ).


Heidelberg retina tomography makes use of a 670 micron diode laser to perform rapid scanning of the fundus. Oscillating mirrors in the HRT device redirect the laser beam to the x – and y -axis, along a plane of focus that is perpendicular to the optic axis ( z -axis). A bi-dimensional image (15 × 15degrees) is obtained at each focal plane. As the device changes the focal plane, other bi-dimensional images of the optic nerve are obtained. Each one represents an optical section of the optic nerve. A total of 64 sections, each done with 1/16 mm of depth interval, are obtained and used to create a three-dimensional image of the optic nerve. These 64 sections are equivalent to a depth of 4 mm.
Each optical section is composed of 384 × 384 points compose each optical section. Each one of these points has an x (horizontal), y (vertical) and z (depth) value to locate it in space. The amount of light or ‘reflectance’ along the z -axis is measured at each scanned point, and the more intense the light is at a given point, the higher (closer to the surface) this is. In this way, the peak distribution of the reflected laser light intensity corresponds to the retinal/ONH surface. The peak intensity along the z -axis is assumed to correspond to the internal limiting membrane that overlies the retina and optic disc. A matrix of retinal height measurements is then created. The result is a topographical map of 384 × 384 height measurements of retinal and optic nerve surface topography. The light intensity measured at each one of the 384 points and used for the creation of the mean topography map is the result of an average of three scans which are automatically obtained by the newer generations of HRT. The transverse resolution is 10 microns and the axial resolution is 300 microns.
Once the image is taken, the operator delineates the optic nerve contour line over the reflectance or topography image. The operator places points at the external edge of the disc border and the machine draws a ‘best-fit’ ellipse linking these points together ( Fig. 14-4 ). Heidelberg retina tomography proceeds to define the reference plane based on the disc contour drawn by the operator. The reference plane is located 50 microns posterior to the mean height along a 6° arc of the contour line at the temporal inferior sector. Structures above the reference plane and within the contour line are considered to be rim. Anything below the reference plane is considered cup ( Fig. 14-5 ). Computation of stereometric parameters, classification of the eye and comparison to previous examinations are then done by the HRT. Classification of the eye is done with Moorefield’s regression analysis or other discriminating method in HRT II, or with neural network analysis in HRT 3.


The latest software available, HRT3, can provide ONH stereometric analysis without manual delineation of the disc margin by the operator. After a three-dimensional model of the ONH is constructed, five optic nerve parameters are calculated and then analyzed with an artificial intelligence classifier, the relevance vector machine (RVM). From this analysis, a glaucoma probability score (GPS) is created.
Adjustments are made to the parameters to account for differences in age and disc size by both versions of HRT. However, HRT 3 is equipped with a larger and more diverse normative database than its predecessor. It currently includes large samples of three different ethnicities: Caucasian (>700), African descent (>200) and Indian or south-east Asian (>100). In comparison, the HRT II normative database includes 112 eyes, all from Caucasian subjects.
There are several printout formats currently available: initial report, follow-up report and ‘OU report,’ among others. Below, the different components of the HRT printout are discussed.
Components of the HRT report
- 1.
Patient data: name, sex, date of birth, patient ID, and date of exam are provided here ( Figs 14-6 and 14-7 ).
Fig. 14-6
HRT 3 baseline printout. After patient information, a Quality score is provided with classification for quick quality assessment. Scores below 30 represent good quality images. The cup section represents the disc topography. The cup parameters shown in this row are cup/disc (C/D) area ratio and cup shape measure. The rim section represents reflectance data with overlay of mean rim area (MRA) classification. The middle column shows rim parameters: rim area and rim volume. The last section contains the RNFL profile graph. It displays the height values at the optic disc margin going around the optic disc from the temporal side, to superior, nasal, inferior, and back to temporal (TSNIT). The green shaded area gives the normal range for that particular age, optic disc size, and ethnicity. Height measures that fall into the yellow zone are borderline, and those that fall into the red zone indicate abnormal values.
Fig. 14-7
HRT II baseline printout.
- 2.
Topography image: located on the left upper corner of the printout. It is a false-color image. More superficial areas appear darker and deeper areas appear of a lighter color. Additional colors are added to the map: red indicates the cup (area below the reference plane) and green and blue indicate neuroretinal rim tissue (above the reference plane). Blue indicates sloping rim.
- 3.
Reflectance image: located in the right upper corner of the unilateral report or below the topography image on the ‘OU report.’ It is also a false-color image and is similar to a photograph with the brighter areas representing highest reflectance, like the cup. The reflectance image is overlaid with Moorfields analysis.
- 4.
Retinal surface height variation graph: this appears once the disc contour is drawn and accepted. It is the graphical representation of the retinal height along the contour line and of the thickness of the nerve fiber layer. A green line represents the retinal height and a red line represents the reference plane. The graph depicts, from left to right: the thicknesses of the temporal (T); temporal-superior (TS); nasal-superior (NS); nasal (N); nasal-inferior (NI); temporal-inferior (TI); and temporal (T) sectors. Because the thickness of the normal retina is irregular, the contour line will appear as what is known as the ‘double-hump.’ The hills or ‘humps’ correspond to the superior and inferior nerve fiber layer, which are normally thicker than the rest of the areas.
- 5.
Vertical and horizontal interactive analysis: these are the optic nerve retinal surface height horizontal and vertical cross-sections. A smooth trace as opposed to a ‘jagged’ trace represents a better quality scan. Observation of the trace can provide information of the disc steepness, presence of sloping, etc.
- 6.
Stereometric analysis: HRT II provides a list of 14 nerve parameters. They are: disc, cup and rim area, cup and rim volume, cup/disc area ratio, linear cup/disc ratio, mean cup depth, maximum cup depth, cup shape measure, height variation contour, mean retinal nerve fiber layer (RNFL) thickness, RNFL cross-sectional area and reference height. To the right of the stereometric parameters, a column specifies ± one standard deviation from the mean of the normative database for each of the parameters. HRT 3 only provides values for 6 stereometric parameters in the ‘OU printout.’ These are: cup/disc area ratio, cup shape measure, rim area and volume, height variation contour and mean RNFL thickness. Each value is designated as within normal limits, borderline or outside normal limits after comparing to the normative database.
- 7.
Moorfields regression analysis (MRA): the MRA is based on a normative database of 112 Caucasian subjects with refractive error <6D and disc size within the range of 1.2–2.8 mm. A predicted rim area/disc area line was obtained after plotting the ratio values among these subjects. Such a predicted line represented the value obtained in 50% of the normal subjects studied. Further classification limits were obtained. In this way, if the rim area of any segment (global assessment or any of the 6 sectors) is below the 99.9% prediction interval, the nerve is classified as outside normal limits (red x). In other words, 99.9 % of ‘normals’ have a higher rim area/disc area value than that of the nerve being classified as outside normal limits (ONL). If the rim area falls between the 95% and 99.9% prediction lines, it is classified as borderline (yellow checkmark). Rim areas that fall above the 95% prediction level are classified as within normal limits (green checkmark). The Moorfields analysis graph is shown in the printout, indicating the predicted intervals on which the nerve classification is based. Seven different columns representing all sectors are shown and classified as within normal limits (WNL), borderline (BL) or ONL. Green color represents the rim and red represents the cup. Moorfields classification is also shown over the reflectance image as a green checkmark, yellow exclamation point, or a red ‘x.’ The Moorfields classification of the nerve is written at the bottom of the graph. Moorfields regression analysis classifies discs based on the worst classified sector.
- 8.
Glaucoma probability score (GPS): new software included in the HRT 3 generation allows calculation of the GPS. It is based on the construction of a three-dimensional model of the ONL and peripapillary RNFL by using five parameters: cup size, cup depth, rim steepness, and horizontal and vertical RNFL. The complete three-dimensional model is then subjected to analysis by an artificial intelligence classifier, the relevance vector machine (RVM), that compares it to a predetermined normal and glaucoma model and then derives the probability of glaucoma for the scanned eye:
- •
Probability ≤ 28% – within normal limits (WNL)
- •
Probability > 28% – borderline (BL)
- •
Probability ≥ 64% – outside normal limits (ONL).
- •
Evaluating scan quality
Only data extracted from a scan of good quality is valuable. Therefore, it is of the utmost importance to know how to identify a poor quality scan. Good quality indicators are even luminance and sharp borders of the topography and reflectance images, as well as good centration of the disc. The standard deviation (SD) is a measurement of variability of the same pixel values among three different scans. The average light intensity for each point is what is used for the RNFL height measurement. The manufacturer has suggested not analyzing scans with a SD value greater than 40. It is important to point out that SD should not be the only parameter to use when assessing quality. A poor quality scan can still have a low SD value if there is small or no variability among the three scans.
The manufacturer’s classification of scans by SD values is:
- •
<10: excellent
- •
11–20: very good
- •
21–30: good
- •
31–40: acceptable
- •
41–50: poor
- •
>50: very poor.
Looking at cross-sections can also help determine the degree of noise in the obtained images. The contour cross-sectional trace should be soft and not ‘jagged.’
Strengths and limitations
Heidelberg retina tomography allows for rapid and simple operation and for three-dimensional representation of the optic nerve without the need for pupil dilation. Heidelberg retina tomography was used in one of the largest glaucoma clinical trials, the Ocular Hypertensive Treatment Study (OHTS), and therefore a large amount of data is available.
Limitations of the HRT include the use of a reference plane that depends on the contour line drawn by the operator. Measurements might be affected by the blood vessels. The nasal border of the nerve can be difficult to identify given that blood vessels can appear crowded in this area and obscure the disc’s edge. Confocal scanning laser ophthalmoscopy is appropriate for scanning the ONH but not the macula or RNFL .
Substantial inter-observer variability exists among HRT parameters, depending on the placement of the contour line. The most dependent parameters were rim volume and disk area and the least dependent were mean height contour and cup shape in one study.
Higher rim measurements obtained from HRT optic nerve analysis compared to planimetric evaluation of disc photos are thought to be in part a result of blood vessel inclusion as part of the disc. These limitations are secondary to potential errors in the delineation of the nerve done by the operator. The GPS analysis bypasses this problem. Early studies regarding the reliability of GPS analysis and its comparison with conventional HRT-MRA analysis are available.
Heidelberg retina tomography’s capability of discrimination between normal and glaucomatous patients has been tested in the past, and parameters were compared to search for the best discriminating parameters. Among the best parameters were cup shape measure, rim area and cup volume. Nevertheless, combining parameters can render the strongest discrimination between groups. Different methods have been designed to analyze the data. Mikelberg’s discriminating analysis and MRA are part of HRT software. Moorfields regression analysis can discriminate glaucomatous nerves from normals with 84.3% sensitivity and 96.3% specificity. Still, the HRT will occasionally call a severely damaged optic nerve normal or a normal optic nerve abnormal.
Heidelberg retina tomography tends to overestimate rim area in small optic nerves and to underestimate rim area in large nerves. So on either extreme of disc size range, care should be taken when analyzing these scans.
New developments
The latest generation is HRT 3. HRT 3 includes the same MRA classification as HRT II, but it is based on a larger, more diverse normative database. It also assigns a GPS to the optic nerve disc. The GPS is based on three optic disc parameters and two RNFL parameters. A three-dimensional optic nerve is constructed and compared to preconstructed models of normal and glaucomatous nerves and a GPS is assigned to the nerve being tested. This analysis is independent of optic nerve contour delineation.
Testing from the patient’s perspective
The patient’s experience is similar to that of having a slit-lamp exam and definitely more comfortable than having a fundus photo taken. The luminance of the diode laser is 100 times lower than the luminance of a digital fundus flash camera. The diode laser used in HRT is safe to the eye. A typical imaging session can be completed in less than 7 seconds.
OPTICAL COHERENCE TOMOGRAPHY (OCT)
Optical coherence tomography (Carl Zeiss Meditec, Inc., Jena, Germany), developed in 1991, is an imaging technology that performs high-resolution, cross-sectional imaging of the ONH, RNFL and macula. It measures the intensity and echo time delay of back-scattered and back-reflected light from the scanned tissues ( Fig. 14-8 ). Optical coherence tomography is analogous to ultrasound B-mode imaging, the difference being that the former uses light and the latter uses sound. It is based on the principle of low-coherence interferometry and the ability to differentiate retina layers depending on the different time delay of their reflections.

A super luminescent 820 or 850 nm diode laser beam is the light source directed to a partially reflecting mirror that splits the light into two beams: one is directed towards a mirror placed at a known distance (reference mirror) and the other is directed towards the eye, from where it will reflect back. This back-reflected light will consist of multiple echoes, with information about the distance and thickness of the different intraocular tissues. The back-reflected light from the eye is combined with the back-reflected light from the reference mirror and coherent light is compared. Interference is produced when two light pulses coincide. The reference mirror is then moved so that the time delay of the reference light pulse can change accordingly and therefore other intraocular structures can be measured ( Figs 14-9 and 14-10 ). The laser beam is panned throughout the tissue and a series of scans are obtained in the manner explained above, until a two-dimensional map is created based on the interference signals detected. The map is color coded in a way that white and red represent areas with high reflectivity and blue and black represent areas with low reflectivity. High reflectivity layers include the nerve fiber layer, retinal pigment epithelium (RPE) and choriocapillaris. Low reflectivity layers or tissues include the photoreceptor layer, choroids and pockets of fluid.

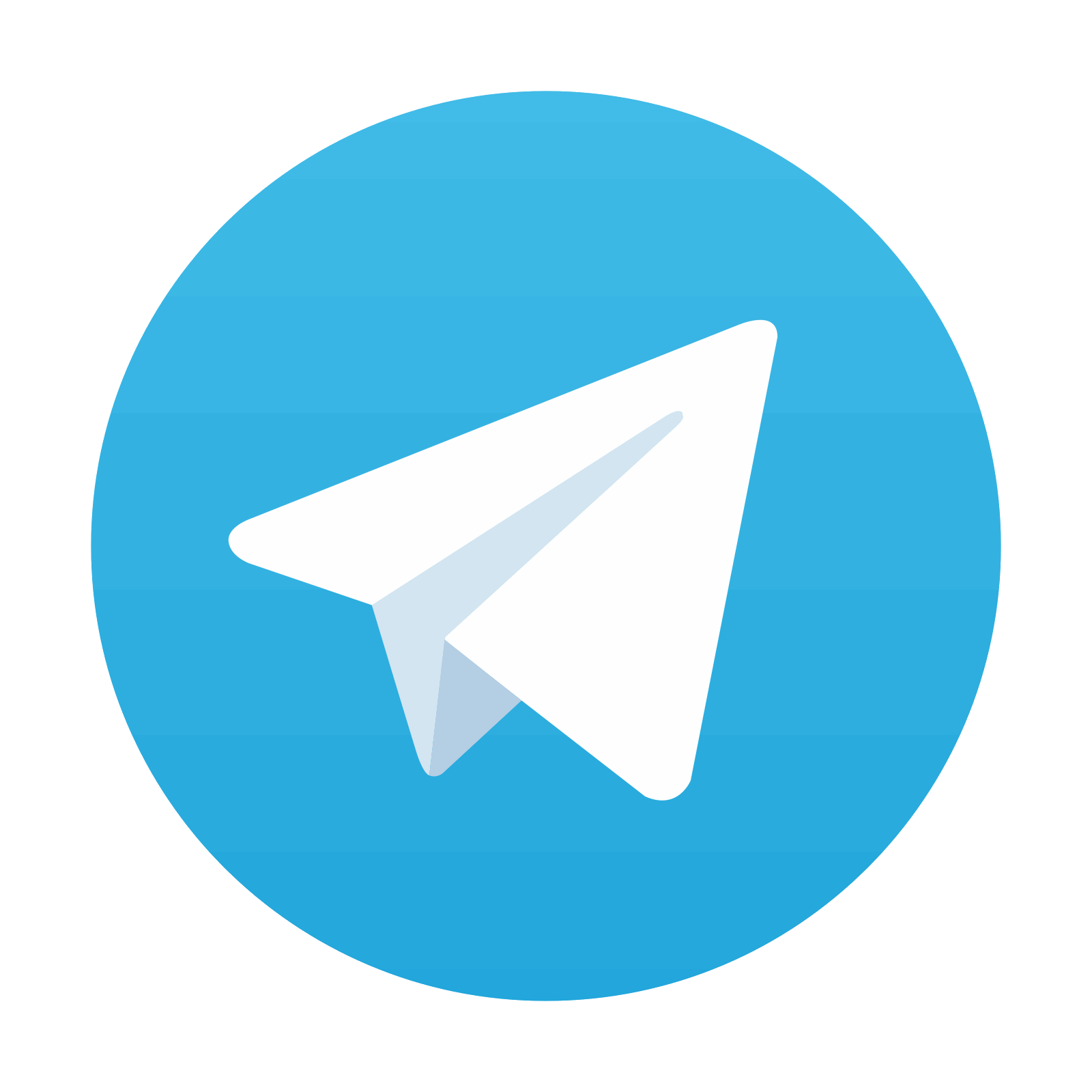
Stay updated, free articles. Join our Telegram channel

Full access? Get Clinical Tree
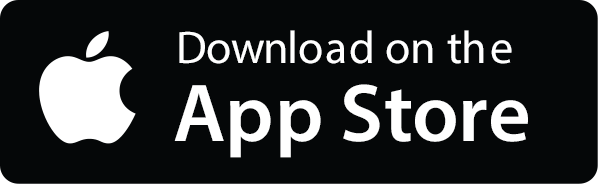
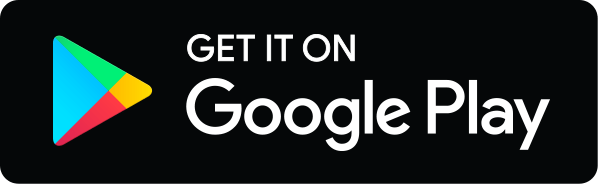
