Diseases that primarily affect the optic nerve may occasionally involve secondarily the macular area or may be mistaken for retinal diseases. Some of the most frequent of these diseases are discussed in this chapter.
Optic Disc Anomalies Associated With Serous Detachment of the Macula
Serous detachment of the sensory retina may occur in association with one or a combination of developmental anomalies of the optic nerve head. This spectrum of anomalies includes pit, coloboma, morning glory deformity, and juxtapapillary staphyloma.
Congenital Pit of the Optic Disc and Serous Detachment of the Macula
Usually between the ages of 20 and 40 years, patients with congenital pit of the optic nerve head may develop serous detachment of the macula ( Figures 15.01–15.03 ). The detachment usually extends in a teardrop fashion ( Figure 15.01 A, I–K) from the disc margin in the vicinity of the optic pit, which in most cases is located along the temporal margin of the optic disc. Pits and detachment are uncommon at the nasal margin of the optic disc ( Figure 15.02 A–C). The optic disc diameter in the affected eye is usually larger than in the unaffected eye. An optic pit may occur bilaterally in 10–15% of cases and may be inherited as an autosomal-dominant abnormality. Pits may be associated with a coloboma of the optic nerve head, and details of the pit may be difficult or impossible to identify ( Figure 15.02 H and I). Some patients with severe colobomatous malformation of the optic disc may develop extensive retinal detachment (see discussion below). Optic pits located in the center of the optic disc are not associated with macular detachment. The optic pit is often covered with a gray membrane that frequently has one or more holes within it, particularly in patients with macular detachments. Most authors agree that there typically is no posterior vitreous detachment in eyes with a pit and serous detachment of the macula. Occasionally, condensed vitreous strands may extend from the surface of the optic pit into the anterior vitreous ( Figure 15.01 M). In some patients a cloudy precipitate may occur on the posterior surface of the detached retina ( Figure 15.01 A, I, and J). When this occurs, the area of detachment may be misdiagnosed as a solid tumor ( Figure 15.03 C and D). Some patients develop a central area of sharply defined retinal detachment with a surrounding larger, less well-defined area of elevation of the inner retinal surface, suggesting the presence of retinoschisis ( Figure 15.01 I–L). Unlike retinoschisis, however, there is not complete loss of retinal function in the area of pseudoschisis in patients with an optic pit. There has been no satisfactory anatomic explanation for this peculiar configuration of retinal elevation seen biomicroscopically in these patients. With prolonged retinal detachment, depigmentation of the retinal pigment epithelium (RPE) occurs in the area of the detachment ( Figures 15.01 K and 15.03 A and B). Cystic retinal degeneration, schisis-like appearance, marked thinning of the foveolar portion of the retina, and rarely full-thickness macular hole and rhegmatogenous retinal detachment may occur ( Figure 15.02 D). Subretinal neovascularization may arise near the optic pit.



In patients with a recent onset of macular detachment, angiography shows no abnormalities in the macular area. In early-phase angiograms the pit appears hypofluorescent ( Figure 15.01 K). In later phases, in most patients, there is evidence of staining in the area of the pit with no evidence of perfusion of dye into the subretinal fluid. Absence of staining of the pit has been associated with absence of retinal detachment, recent macular detachment, and no cilioretinal arteries emanating from the pit. Staining of the subretinal fluid occasionally occurs ( Figure 15.01 K). Patients with loss of pigment in the RPE caused by prolonged retinal detachment show hyperfluorescence corresponding with the areas of depigmentation during the early phases of angiography ( Figure 15.01 K). This is often seen in the papillomacular bundle region adjacent to the optic disc in patients with no previous history of macular detachment. Angiography shows no evidence of either choroidal or retinal capillary permeability alterations. The failure to demonstrate angiographically either retinal or choroidal permeability alterations in the presence of a serous macular detachment should always alert the clinician to the possibility of an optic pit or a more peripheral lesion to account for the detachment. Optical coherence tomography (OCT) through the pit shows the defect, and any communication with the vitreous and/or the subarachnoid space ( Figure 15.01 M). OCT through the macula can show either a serous retinal detachment ( Figure 15.03 H and J) or a schisis or both ( Figures 15.01 L and 15.03 I).
Presently there is limited information concerning the natural course of eyes with an optic pit before or after development of serous macular detachment. It is probable that only a small percentage of eyes with a pit ever develop serous retinal detachment. Spontaneous re-attachment of the macula occurs in 25% or more of patients with optic pits. With a prolonged delay in reattachment, cystic degeneration and partial- or full-thickness hole formation may occur. Long-term follow-up of untreated eyes suggests that in approximately 50–75% of eyes the visual acuity will be reduced to 20/100 or worse within 5–9 years.
Histopathologically, an optic pit consists of herniation of dysplastic retina into a collagen-lined pocket extending posteriorly through a defect in the lamina cribrosa into the subarachnoid space ( Figure 15.03 C and D).
The pathogenesis of the detachment appears to involve the passage of fluid from the area of the pit into the subretinal space. The failure of intravascular fluorescein to diffuse into the subretinal fluid in all but a few cases suggests that it is derived from either the cerebrospinal fluid or the vitreous ( Figure 15.03 E). Chang and associates reported metrizamide cisternographic evidence of communication of the subretinal and subarachnoid spaces in a child with a colobomatous malformation of the optic nerve and extensive retinal detachment. Other studies involving radioisotope cisternography and intrathecal fluorescein, as well as attempts to displace subretinal fluid into the subarachnoid space by elevating the intraocular pressure, have failed to demonstrate evidence of direct communication between the subarachnoid space and subretinal space. One patient with an optic pit experienced two episodes of increased intracranial pressure caused by pseudotumor cerebri without developing a retinal detachment. Direct communication between the vitreous cavity and subretinal space via the optic pit has been demonstrated in collie dogs ( Figure 15.03 E). This communication could not be demonstrated in a human eye with an optic pit.
In general, attempts to close the neck of the detachment at the margin of the optic disc with photocoagulation, as well as photocoagulation of the optic pit itself, have been unsuccessful in causing prompt resolution of the macular detachment ( Figures 15.01 A–C, G, and H and 15.03G–I). Several authors have reported resolution that may require several months or longer after photocoagulation. Redetachment may occur weeks or months later. The absence of posterior vitreous separation in patients with macular detachment suggests that traction of the formed vitreous in these patients on the anterior surface of the retina throughout the macular area may be important in causing the passive movement of either fluid vitreous or of cerebrospinal fluid through a defect within or at the margin of the optic pit into the subretinal space. In older patients whose posterior vitreous is extensively liquefied full-thickness holes in the macula and elsewhere in the posterior fundus do not cause retinal detachment in the absence of biomicroscopic evidence of focal vitreous traction. Some authors have reported successful reattachment of the macula using a combination of one or more of the following: pars plana vitrectomy, intravitreal gas tamponade, and photocoagulation. No technique of treatment has proved uniformly successful in permanently reattaching the retina. Ocassionally gas and/or emulsified silicone oil can migrate through the pit intracranially. This is especially likely to occur when the gas bubble is smaller than the size of the pit or when the intraocular pressure is too high. A reasonable recommendation is observation for at least a month after the onset of detachment; if no improvement in the detachment occurs, photocoagulation across the neck of the detachment; if no response occurs within 6–8 weeks, repeat the laser treatment; if still no improvement in the detachment, consider intravitreal gas tamponade with or without pars plana vitrectomy. Use of intravitreal tissel tissue glue to cover the pit has been reported. The author has used tissel successfully in a patient with two previous vitrectomy failures. Use of prophylactic treatment either adjacent to the pit or as a coarse scatter pattern of laser in the macula to prevent detachment might be considered in the rare patient with a strong family history of detachment associated with optic nerve pit, and particularly in the second eye if the first eye has permanent loss of acuity from this complication.
Acquired Pits of the Optic Nerve
Pit-like changes in the optic nerve head may be acquired and may be the cause of unexplained loss of paracentral and occasionally central visual field loss. These pits typically develop in the inferotemporal quadrant in older patients with normal intraocular pressure or glaucoma. There is an increased incidence of acquired pits in patients with low-tension glaucoma (74%) versus patients with typical glaucoma (15%). Development of the pit may be preceded by the appearance on one or more occasions of a flame-shaped hemorrhage on the optic disc. The typical field defect in normotensive eyes extends from the blind spot to near fixation in a pistol-shaped configuration and has a steep central margin.
Because patients with acquired pits and with low-tension glaucoma have significantly greater amounts of field loss than those with elevated intraocular pressure without pits, it is probable that optic nerves predisposed to developing pits are more susceptible to damage from the damaging effects of intraocular pressure. Whereas there are structural differences in the lamina cribrosa in patients with and without low-tension glaucoma, the pathogenesis of the acquired pit of the optic disc is still not clear. It is postulated that these patients have cavitatory defects behind the lamina cribrosa, the walls of which collapse with elevated pressure and become confluent to look like a pit or a large cup. Ischemic necrosis in eyes with poor optic nerve head circulation may be responsible in some eyes.
Some of these patients develop macular thickening leading to schisis-like change that can sometimes progress to macular detachment. This has been noted in acute angle closure glaucoma, juvenile glaucoma, traumatic and primary open-angle glaucoma. The acute rise or frequent spikes in intraocular pressure may force fluid into the inner retina through small defects at the edge of the disc or cup. The increasing fluid may eventually dissect into the subretinal space in some eyes. Lowering the intraocular pressure with surgery or medications results in resolution of the fluid in some eyes; others require a vitrectomy and gas displacement. The schisis may be subtle and may be mistaken for cystoid macular edema secondary to prostaglandin inhibitors or be treated without success with topical nonsteroidal agents.
Coloboma, Juxtapapillary Staphyloma, and Morning Glory Deformity
A coloboma of the optic nerve head involves a defect in its structure occurring as a result of malclosure of the ocular fissure. It may be mild, in which case there is a defect in the optic nerve substance, usually inferiorly. This defect may be more extensive and involve the juxtapapillary choroid and retina. It may be associated with a pit deformity and with a juxtapapillary staphyloma. This latter term refers to an outpouching of the ocular wall around the optic nerve head. This outpouching may occur with little or no abnormality in the structure and function of the eye, or with varying degree of dysplasia of the nerve. If the coloboma or staphyloma is filled with glial tissue, the retinal blood vessels may exit from this tissue in a pattern that has suggested to some a morning glory ( Figure 15.02 K and L). These more severe anomalies of the optic disc may be associated with serous macular detachment ( Figure 15.04 D–H); with other ocular abnormalities, including microphthalmos, lens coloboma, persistent primary hyperplastic vitreous, orbital cyst, and occasionally intracranial abnormalities such as a basal encephalocele ( Figure 15.04 B and C), and midline defects including pituitary stalk duplication, and moya moya disease.


(D and F; Also, Yannuzzi L J., The Retinal Atlas, Saunders 2010, 978-0-7020-3320-9, p. 885.)
(B and C, courtesy of Dr. M. Tariq Bhatti; D–H, courtesy of Dr. Edwin H. Ryan Jr, D and F, in Yannuzzi, Lawrence A. The Retinal Atlas. Philadelphia: Saunders/Elsevier, 2010. p.885.; I and J from Seybold and Rosen ; K, courtesy of Dr. A.R. Frederick Jr; L and M, courtesy of Dr. William H. Spencer, O and P, courtesy of Dr. Louise Mawn; T to Y, courtesy of Dr. Cameron Parsa, reprinted with permission from Ophthalmology. Elsevier 109 .)
A pit deformity may or may not be present and is often obscured clinically by other anomalies ( Figure 15.02 K and L). In some cases these anomalies may be combined to form a mass lesion simulating a capillary angioma, astrocytoma, combined retinal–RPE hamartoma, or melanoma (Figure 15.04B) in the region of the optic disc. Some patients may manifest clinical evidence of communication between the vitreous and retrobulbar ocular cysts via the optic disc anomalies. In patients with these severe disc anomalies the retinal detachment begins in the juxtapapillary area, often on the temporal side. The detachment, unlike that occurring with a pit alone, may extend to involve most of the fundus ( Figure 15.02 K and L). These detachments, like those associated with a pit, may resolve spontaneously. The pathogenesis of the detachment occurring with all of these anomalies is probably similar in most cases, although this is controversial. In the morning glory deformity and juxtapapillary staphyloma, the detachment has been attributed to retinal breaks in the vicinity of the anomaly, to communication between the subarachnoid and subretinal space, to communication between the vitreous and subretinal space, to communication with both the vitreous and subarachnoid space, to vitreoretinal traction, and to exudation from blood vessels within the anomaly, the orbital tissue, and juxtapapillary choriocapillaris. Vitreoretinal traction is probably important in all cases, and successful repair of the detachment has been accomplished by vitrectomy, intravitreal gas, and thermal treatment at the edge of the anomaly. Choroidal neovascularization may occur at the edge of any of these anomalies.
Familial cases have been associated with mutations in the PAX6 gene on chromosome 11p13 ; however, given the association of morning glory discs with dissimilar anomalies, a more complex genetic influence is likely.
Transient Obscuration of Vision Secondary to Peripapillary Staphyloma
Peripapillary staphyloma is a rare congenital anomaly in which the normal or nearly normal optic nerve head lies in the depth of an excavation in the fundus ( Figure 15.04 I, K, and N). It is usually present in only one eye. If the staphyloma does not involve the macula, the visual acuity may be normal ( Figure 15.04 I and N). In adulthood these patients may complain of transient obscurations of vision that in some cases may be associated with intermittent dilation of the retinal veins. Figure 15.04 I illustrates a mild degree of peripapillary staphylomatous formation that was initially misinterpreted as a choroidal hemangioma in a young woman with transient obscurations of vision.
Peripapillary staphylomata may occasionally be associated with contractile movements of the walls of the staphyloma ( Figure 15.04 K). These contractions are not associated with the patient’s respiration or pulse rate. There is some histopathologic evidence to suggest that the presence of atavistic smooth muscle in and around peripapillary staphylomata may be responsible for this contraction and, further, may be responsible for the transient obscuration of vision ( Figure 15.04 L and M). Contractile movements have also been described in patients with choroidal coloboma and morning glory syndrome. In 1962 Longfellow and coworkers reported a young man with unilateral intermittent blindness associated with marked dilation of the retinal veins of undetermined cause. He had minimal abnormality of the optic nerve head, but the clinical findings otherwise suggest the possibility of an anomalous smooth-muscle sphincter around the retrobulbar optic nerve.
Papillorenal (Renal Coloboma) Syndrome
First described in 1977 by Rieger, it was considered to be a rare autosomal dominant disorder of the optic disc and kidneys. Work by Parsa and colleagues suggests that the highly variable phenotype may be responsible for its underdiagnosis. Initial description was of anomalous optic discs associated with hypoplastic kidneys resulting in hypertension and renal failure. Serous retinal detachment extending to the macula accompanies the disc anomalies in some cases.
The extended ophthalmic features of papillorenal syndrome include large optic discs with several cilioretinal arteries with hairpin-like loops emerging at the edge of the disc ( Figure 15.04 O–S). A large portion of the central part of the disc is devoid of vessels or has rudimentary cilioretinal vessels. Along with multiple cilioretinal vessels, the retinal vasculature is not fully developed, and the choroid is affected to various degrees in certain families (Figure 15.04T-V). It appears that there may be a primary deficiency in the vascular development that compromises growth of substantial portion of the retina and the choroid. Cats and lemurs have a poorly defined central retinal artery and vein and most of the retinal circulation is derived from ciliary vessels in these animals. The eyes with papillorenal syndrome seem to have converted to this feline pattern of circulation. Papillorenal syndrome is likely a hereditary vascular dysgenesis that most severely affects the ocular and renal circulation, the most vascular organs. Visual field defects that do not correspond to glaucomatous changes are seen; this is explained by the absence of retinal ganglion cells and decreased retinal thickness as a result of defect in the vasculogenesis of the retina and choroid. Magnetic resonance imaging (MRI) shows hypoplasia of the optic chiasm due to defect in the decussating fibers of the optic nerve. A significant number of these discs may be misdiagnosed as morning glory or normal-tension glaucoma. The associated kidney diseases include renal hypoplasia, renal hypertension, and renal failure. There is extreme resistance to renal blood flow within the renal parenchyma resulting in renal hypertension. The recurrent pyelonephritis and kidney stones is a variant of expression of the renal disease.
There may be several defects in the PAX2 gene to account for the variable phenotype. In 1995, Sanyanusin et al. discovered mutations in the developmental gene PAX2 in two families with papillorenal syndrome. Schimmenti et al. identified three additional families with ocular and renal abnormalities, including vesicoureteral reflux. Since then five other families with mutations of the PAX2 gene have been reported. However, not all cases can be explained by the PAX2 mutations.
The features that should alert a diagnosis of papillo-renal syndrome include the “vacant discs,” presence of multiple ciliary retinal vessels with their abnormal insertion posterior to the globe, peripheral dysgenesis of the retinal vessels, hypoplasia of the optic chiasm on MRI, and visual field defects that do not correspond to glaucoma.
Optic Disc Hypoplasia and Tilted-Disc Syndrome
Mild dysplasia of the optic nerve must be considered in patients with unexplained visual loss. There is considerable variation in the size of the normal optic disc. The disc diameter is often directly related to eye size and refractive error. Some anomalies of the disc may be either overlooked or misinterpreted as papilledema. Failure to recognize a disc anomaly in a patient with a visual defect may cause initiation of an unnecessarily extensive evaluation for a retinal, retrobulbar, or intracranial lesion.
Moderate or severe hypoplasia of the optic disc is often associated with a visual defect ( Figure 15.05 ). Ophthalmoscopic clues to its presence include reduction in the disc diameter, a low disc/artery ratio, and the peripapillary double-ring sign ( Figure 15.05 A and B). This sign consists of a yellow-gray peripapillary halo delineated by an outer ring corresponding to the junction between the sclera and lamina cribrosa and an inner ring caused by the termination of the RPE. The diagnosis of mild degrees of hypoplasia may be difficult or impossible. Several techniques for measuring and defining optic disc hypoplasia have been described. Romano, using photogrametric methods, found no overlap between the horizontal diameters of normal and hypoplastic discs. He directly measured the horizontal diameter of the discs taken on a standard 30 degree field fundus camera with a x2.5 magnification. The range in diameters for hypoplastic discs was 1.8–3.27 mm, with a mean of 2.64 mm, as compared to a range of 3.44–4.7 mm and a mean of 3.88 mm for normal discs. Zeki and others used the ratio of the distance from the edge of the disc to the center of the fovea to disc diameter to define optic disc hypoplasia; a ratio of 3 to 1 or greater characterizes a hypoplastic optic disc. Disc hypoplasia may be unilateral or bilateral ( Figure 15.05 C and D). In bilateral cases the eye with the smaller disc often has a better Snellen acuity, indicating that factors other than size determine the visual function, e.g., macular hypoplasia, high refractive error, amblyopia, central scotoma, and optic atrophy. A hypoplastic disc with a large central cup may have a disc diameter of normal size. Hypoplastic optic discs may occasionally be supplied largely by cilioretinal arteries. Disc hypoplasia may be associated with other extraocular or intraocular anomalies (e.g., aniridia) ; it may be segmental ; and it may be associated with normal visual acuity ( Figure 15.05 C and D). Superior segmental disc hypoplasia may occur as a sign of maternal diabetes and can be diagnosed by finding characteristic inferior visual field defects.

Optic nerve hypoplasia can result from an insult to the embryo at any level of the optic pathway. Evidence for a neuroendocrine disorder should be sought in any child presenting with bilateral optic disc hypoplasia, because of the frequent association of hypothalamic and pituitary dysfunction, partial or complete absence of the septum pellucidum, midbrain abnormalities, hypotonia, hydrocephalus, porencephaly, and orthopedic deformities (de Morsier’s syndrome) ( Figure 15.05 D and F–I). Other known associations include aniridia, monocular temporal hemianopsia, contralateral megalopapilla, microphthalmia, achiasmia, polymicrogyria, periodic alternating-gaze nystagmus, intracranial arachnoid cyst, oval cornea and lens duplication, tortuous retinal veins, and mitochondrial cytopathies.
In a 100-case series, optic nerve hypoplasia was associated with premature birth (21%), maternal diabetes (6%), fetal alcohol syndrome (9%), and endocrine abnormalities (6%). Thirty-two percent had associated developmental delay, 13% cerebral palsy, and 12% seizures. Sixty percent had abnormal neuroimaging, including ventricular and white- or gray-matter abnormalities, septo-optic dysplasia ( Figure 15.05 J), corpus callosum abnormalities, and hydrocephalus. Associated clinical neurological deficits were seen in 57% of bilateral and 32% of unilateral cases.
No specific gene defect has been found, though isolated cases have been associated with PAX6 defect, pericentric inversion of chromosome 9, trisomy 18, 5p deletion (cri du chat syndrome), SOX2 , SOX, and HESX1 gene mutations.
The tilted-disc syndrome has the following features: the long axis of the oval optic disc is obliquely directed; the upper and temporal portion of the disc lies anterior to the inferonasal portion; the retinal vessels emerge from the disc tissue in the upper and temporal aspect rather than nasally (situs inversus); there is an RPE conus in the direction of the tilt, as well as a large area of hypopigmentation and staphylomatous ectasia inferonasal to the optic disc; myopic astigmatism is present; and visual field depression occurs bitemporally (not truly hemianopic) ( Figure 15.05 E). Central vision may or may not be affected. The asymmetry of the disc elevation with the ill-defined margins superiorly may be mistaken for papilledema. Visual loss may be caused occasionally by choroidal neovascularization. The disc anomaly may occur in association with other, nonocular anomalies.
Drusen (Hyaline Bodies) of the Optic Nerve Head
Drusen are discrete, multiple, amorphous, partly calcified extracellular deposits in the prelaminar portion of the optic nerve reported in 2% of population. In small numbers they may be present deep within a normal-appearing optic nerve head. In larger numbers in children and young adults, they cause a swollen nerve head that may simulate papilledema ( Figure 15.06 A and B). As they become larger, more calcified, and associated with atrophy of surrounding nerve fibers, they become visible as discrete crystalline structures later in life ( Figure 15.06 C and E and 15.07J and K). When buried, they are most easily detected by retroillumination biomicroscopically. Although a “lumpy, bumpy” appearance to a swollen disc suggests the presence of drusen ( Figure 15.06 E–L), a similar picture occasionally occurs in papilledema. They frequently occur in small discs and may be associated with anomalous branching and tortuosity of the retinal vessels ( Figures 15.06 A and B and 15.07A and B).

In some patients drusen may cause slow progressive loss of visual fields (characteristically inferonasal) in a nerve fiber distribution. Drusen may be associated with abnormal visual evoked potentials. In a few patients drusen of the optic disc may lead to severe loss of central vision. Drusen may also cause acute visual loss, presumably resulting from acute swelling of the optic nerve head induced by the drusen interfering with the blood supply of the nerve ( Figure 15.07 G). This swelling may be evident ophthalmoscopically and may be accompanied by a few flame-shaped hemorrhages and cotton-wool patches, a picture suggesting anterior ischemic optic neuropathy (AION). In others the swelling of the optic nerve may be less apparent and cause obstruction of the central retinal vessels. In those eyes that develop an AION, the prevalence of vascular risk factors, pattern of visual field loss, and incidence of second eye involvement are similar to eyes without disc drusen; however they are younger, enjoy a better prognosis, and more often report transient visual obscurations. Optic disc drusen may also cause loss of vision associated with subretinal exudation and hemorrhage derived from peripapillary choroidal neovascularization ( Figure 15.07 A–F). Bleeding into the subretinal space may occur adjacent to the optic disc in the absence of angiographic evidence of subretinal neovascularization ( Figure 15.07 A). Very rarely Pulfrich phenomenon is reported, likely from delayed conduction in one eye compared to the other, and this can be minimized by using a tinted lens on the affected side.

Other fundus findings that have occurred in association with drusen of the optic nerve head include retinitis pigmentosa, retinal hemorrhages, angioid streaks in patients with pseudoxanthoma elasticum (see Figure 3.38I and 3.40H to L) subfoveal choroidal neovascularization, chronic papilledema associated with pseudotumor cerebri, and chorioretinal folds (see Chapter 4). Disc drusen have been reported in children with primary megacephaly. Drusen bodies of the optic nerve head occur commonly, and therefore many associated findings may be coincidental. The association of drusen and chorioretinal folds probably is not coincidental. Most chorioretinal folds are probably acquired and are caused by some subclinical inflammatory process causing shrinking and flattening of the posterior sclera. This process also causes narrowing of the optic canal and in turn may predispose the optic nerve head to drusen accumulation and other complications such as ischemic optic neuropathy and obstruction of the central retinal vessels. In some patients hyaline bodies are inherited as an autosomal-dominant trait.
Fluorescein angiography is helpful in identifying alterations in the normal optic nerve vascular pattern as well as in identifying subretinal neovascularization associated with drusen. By virtue of their autofluorescence, drusen near the surface of the optic disc can be detected with fundus photography using appropriate filters ( Figure 15.06 F, G, J, and K). The author has found this technique helpful in detecting drusen that were not visible biomicroscopically. OCT is helpful in monitoring the change in contour of the disc, with changes in the size or anterior movement of the drusen over time ( Figure 15.06 H and L).
Ultrasonography is also helpful in detecting optic disc drusen, particularly when they are buried in an optic disc that appears normal clinically, or in cases of unexplained optic disc swelling. Failure to demonstrate calcified bodies in the optic nerve head of infants and children with a swollen optic nerve head may not exclude the presence of drusen. It is possible, although not proven histopathologically, that drusen may be relatively noncalcified hyaline structures in young patients. The calcification caused by drusen anterior to the level of the lamina cribrosa should not be confused with that located several millimeters posterior to the lamina cribrosa. The cause of this latter focal calcification is uncertain. In some patients the calcification may be located in the walls or lumen of the central retinal artery, where it may develop as a degenerative change in association with atheromatous disease, or it may be a calcific embolus derived from the aortic valve. These focal retrolaminar calcifications may be found in some eyes with central retinal artery occlusion. Computed tomography (CT) is also capable of detecting buried drusen.
Histopathologically, drusen are calcified extracellular bodies located anterior to the lamina cribrosa ( Figure 15.06 D). They are associated with small scleral canal, crowding of nerve fibers, partial optic atrophy, elevated disc margins, cytoid bodies, dilated capillaries, juxtapapillary subretinal hemorrhage, subretinal neovascularization ( Figure 15.07 B–F), retinal scarring, and calcification. Histochemically they are composed of a mucoprotein matrix containing acid mucopolysaccharides, ribonucleic acid, and occasionally iron. Their pathogenesis has been ascribed to RPE migration, hyaline degeneration of the neuroglia, accumulation of degenerative products of axons, and coalescing intracellular deposits of glial cells, transudative vasculopathy, and axoplasmic transport alterations. It is probable that a congenital, and less commonly an acquired, small optic nerve scleral canal that is crowded with nerve fibers is responsible for a local disturbance of axoplasmic transport and drusen formation. Tso found ultrastructural evidence to suggest that optic disc drusen are the result of abnormal intracellular metabolism and calcification of mitochondria. The mitochondria are extruded into the extracellular space, where they act as nidi for continued build-up of calcific deposits. Acquired causes of chronic crowding of the optic nerve fibers such as idiopathic chorioretinal folds (see Chapter 4) and pseudotumor cerebri may also be a factor in drusen formation.
The long-term visual prognosis for most patients with drusen of the optic disc is probably good. Those with buried drusen have fewer visual field defects than those with visible drusen. Visual field defects may show progression in some patients. The natural course of those patients who develop juxtapapillary subretinal neovascularization is variable, and some patients may retain central vision in spite of peripapillary subretinal hemorrhage.
The biomicroscopic diagnosis of drusen of the optic disc is relatively secure if the calcified bodies are visible within the elevated optic nerve head. Occasionally noncalcified drusen-like changes are caused by papilledema. The differential diagnosis of juxtapapillary choroidal neovascularization associated with a swollen optic disc includes the presumed ocular histoplasmosis syndrome, angioid streaks, idiopathic choroidal neovascularization, sarcoidosis, papilledema with pseudotumor cerebri, and congenital pit of the optic disc.
Hereditary Optic Neuropathies
The heredodegenerative optic neuropathies must be considered in patients with insidious as well as rapid loss of central vision.
Dominant Optic Atrophy (Kjer Type)
Patients with dominant optic atrophy note the insidious onset of mildly progressive loss of visual acuity, usually beginning before 10 years of age. Visual loss is bilateral but may be asymmetric. Many patients are unable to recall the time of onset of the disease, and some may be asymptomatic. Although the optic atrophy is dominantly inherited, a positive family history may not be obtained ( Figure 15.08 ). Interfamilial and intrafamilial variations in acuity loss may occur (range: 20/30–20/400). The characteristic field defect is a cecocentral scotoma. Depression of the temporal isopters may simulate a bitemporal hemianopia. Constriction of peripheral isopters is rare. Tritan (blue) dyschromatopsia is the characteristic color defect in dominant optic atrophy. A generalized dyschromatopsia, however, may be present in some cases. Temporal optic disc pallor, often with a triangular area of temporal excavation, is characteristic ( Figure 15.08 A and B). Although uncertainties remain concerning the pathogenesis of the frequently encountered West Indian optic atrophy, it is probable that many cases are examples of dominant optic atrophy ( Figure 15.08 B and C; see discussion of nutritional amblyopia below).

Weleber and Miyake described familial optic atrophy associated with “negative” electroretinograms in two families with loss of central vision occurring in the second and third decades of life, optic atrophy, defective color vision, mild to moderate myopia, and pericentral or central scotomas.
The OPA1 (optic atrophy type 1) gene is localized to a 1.4-cM interval on chromosome 3q28-q29. Missense, nonsense, deletion frameshift and splice site alterations accounting for more than 100 mutations have been seen in various pedigrees of patients with dominant optic atrophy. This explains the heterogeneity of the pathophysiology of the disease and the variable phenotype ( Figure 15.08 G–L). The penetrance of the gene varies from 43 to 89%. It is likely that environmental factors also play a role in disease severity in susceptible individuals. The OPA1 protein is anchored to the mitochondrial cristate inner membrane and is believed to prevent cytochrome c release from mitochondria and block organelle fragmentation, thus protecting the cells from apoptosis. It is likely there are other hitherto unknown gene defects that account for some cases of dominant optic atrophy.
Leber’s Hereditary Optic Neuropathy
Leber’s hereditary optic neuropathy (LHON) is a maternally inherited disease that is characterized by acute, severe, bilateral visual loss in healthy young persons (15–35 years), usually males (80–90%). The visual loss can occur in younger and older individuals and the age range of onset is 2–80 years. This variability of age at onset can occur in members of the same family. The central visual loss is acute or subacute at onset, painless, and accompanied by large cecocentral scotomata and dyschromatopsia. Central vision deteriorates progressively, first in one eye then in the other, typically with an interval of days to weeks. Intervals of as long as 12 years have been reported. Transient worsening with exercise or warming, as occurs in other optic neuropathies (Uhthoff’s symptom), may occur. All levels of visual acuity loss have been reported ranging from no light perception to 20/20, but it commonly declines to levels worse than 20/200 bilaterally, usually over several weeks to several months. Although these patients are typically between the ages of 18 and 30 years at onset, visual loss may not occur until the sixth decade or beyond, when the clinical picture may be mistaken for AION. Color vision is affected early, and visual field examination reveals a central or cecocentral scotoma. Biomicroscopy reveals circumpapillary telangiectatic microangiopathy, swelling of the nerve fiber layer around the disc (pseudo-edema), and absence of fluorescein leakage ( Figure 15.09 ). Most patients show no improvement but partial or even complete recovery may occur 5–10 years after onset of visual loss. Environmental triggers such as smoking, trauma, human immunodeficiency virus (HIV) infection can precipitate onset of visual loss in susceptible individuals carrying the genetic defect. Dilation of the optic nerve sheaths with cerebrospinal fluid has been demonstrated by ultrasonography (30° test) and histopathologically. Pathology of the retina and optic nerve is available only for late-stage disease. Ganglion cell atrophy, excavation of the optic disc cup filled with glial and connective tissue, and symmetric destruction of the myelin sheaths in the optic nerve transmitting papillomacular bundle fibers are the findings within the eye. The optic chiasm and tracts also showed loss of myelin sheaths and axis cylinders centrally with relative preservation of peripheral fibers. Shriveled lateral geniculate bodies and demyelination of the optic radiations suggest transsynaptic degeneration.

Visual dysfunction is the only manifestation of LHON in most patients. Although other neurologic disorders are occasionally reported (LHON “plus”), the best-established link is between LHON and cardiac conduction abnormalities. Wolff–Parkinson–White and Lown–Ganong–Levine are the most common associations (9%). Prolonged QT interval, syncope, palpitations, and sudden death are seen occasionally. Minor neurological associations include exaggerated reflexes, myoclonus, seizures, muscle wasting, sensory and auditory neuropathy, and migraine. More severe associations include movement disorders, spasticity, psychiatric disturbances, skeletal abnormalities, acute infantile encephalopathic episodes, dystonia, Leigh-like encephalopathy, periaqueductal syndrome, and demyelinating disease resembling multiple sclerosis. A similar optic neuropathy has been reported with skeletal abnormalities, and in association with Charcot–Marie–Tooth (CMT) disease, a hereditary disease of peripheral nerves in at least two families. Uemura and coworkers have identified mild but distinct biochemical and electron microscopic changes in muscle biopsy in patients with LHON. Wallace and others, in 1988, identified a mitochondrial replacement mutation in nine of 11 families with members diagnosed with LHON.
When LHON appears for the first time in a family, the diagnosis is often delayed or missed. In the acute stage, circumpapillary telangiectatic microangiopathy, swelling of the nerve fiber layer around the optic disc (pseudoedema), and absence of staining on fluorescein angiography are characteristic ( Figures 15.09 and 15.10 B). Progressive enlargement of the blind spot until it reaches fixation characterizes the early progression of field loss. Many asymptomatic family members with Leber’s disease have peripapillary microangiopathy. These vascular changes may be evident angiographically and occur years before the acute phase of the disease. They include progressive arteriovenous shunting that starts in the inferior arcuate nerve fibers and that may be associated occasionally with preretinal hemorrhages. Acute visual loss is accompanied by dilation of the branches of the central retinal artery and peripapillary telangiectatic capillaries. These changes disappear as atrophy of the optic nerve develops ( Figure 15.09 D). Unlike dominant optic atrophy, the atrophy often progresses to involve the entire optic disc. Retinal arterial narrowing and increased circulation time occur. Angiography is probably useful in excluding Leber’s disease in asymptomatic individuals. Acquired red–green deficiency characterized by a deutan-like discrimination defect is characteristic and may be detected in some asymptomatic carriers. Electroretinography and dark adaptation are usually normal in these patients. Visual loss typically stabilizes soon after onset, but some patients may show either improvement or worsening. There is no effective treatment for the disease. Since the primary locus of the disease appears to be in the intraocular rather than the retrobulbar area, Leber’s hereditary vascular neuroretinopathy has been suggested as a more appropriate name. LHON is strictly maternally inherited and passes to future generations only through women. An affected male never has affected offspring, whereas a woman may have affected children although she herself is visually normal.


LHON is associated with four different point mutations of mitochondrial DNA that appear to be pathogenetic for the disease. These mutations affect nucleotide positions 11778, 14484, 3460, and 15257 and are located within the genes that code for proteins in the complex I of the respiratory chain. The clinical findings are similar, except that patients with 14484 are more likely to experience visual recovery than patients with the other three mutations. Patients with 15257, who also have an associated mutation at 15812, are less likely to recover vision than those without this association. Patients with the 15257 mutation also have a higher incidence of spinal cord and peripheral neurologic symptoms than patients with the other mutations. Molecular genetic testing is of practical value in confirming the diagnosis of atypical cases or in patients presenting with optic atrophy, in the absence of a family history, and after the characteristic telangiectasis is no longer evident. The proportion of mutant mitochondrial DNA molecules was found to shift markedly across generations and within tissues of an individual (heteroplasmy). This explains the variability of expression of the disease in family members. Approximately 20% of men and 4% of women experienced visual loss in an Australian study where a dramatic decline in the penetrance of the disease has been noted. Therefore, successful determination of the mitochondrial DNA genotype of a family or patient with LHON requires testing of more than one family member and more than one tissue from each individual. Molecular genetic tests are 100% specific but only 50% sensitive for the diagnosis of LHON. It may be prudent to advise carriers to avoid exposure to toxins such as tobacco smoke and excessive alcohol intake since they may further compromise mitochondrial energy metabolism.
Leber’s Idiopathic Stellate Neuroretinitis and Multifocal Retinitis (See Chapter 10)
In 1916 Theodor Leber described the clinical syndrome characterized by unilateral loss of vision, optic disc swelling, macular star, and spontaneous resolution of unknown cause in otherwise healthy patients ( Figures 15.10 D–L and 15.11). In two-thirds of the patients seen at the Bascom Palmer Eye Institute, a viral-like illness has preceded the onset of symptoms. In most patients the visual acuity ranges from 20/50 to 20/200 and an afferent pupillary defect is present. During the first week after the onset of symptoms, the macular star is usually preceded by mild swelling of the optic disc and peripapillary exudative detachment of the retina ( Figures 15.10 D, J, K and 15.11A and J). In a few patients the swelling of the disc may be more marked and may be associated with splinter hemorrhages. One or more focal white retinal lesions may occur ( Figures 15.10 H–J and 15.11F and I–L). These focal areas of retinitis may cause occlusion of either a branch retinal artery or a vein. In some patients the multifocal retinitis is not associated with optic disc involvement. Vitreous cells are present in 90% of cases. Anterior uveitis is present in a few patients. Within several days or weeks, the peripapillary exudate begins to subside and a macular star appears and becomes more prominent as the disc and peripapillary swelling disappears ( Figure 15.10 F and J). Within the first several weeks the visual acuity begins to improve, and with a few exceptions it eventually returns to normal. The macular star usually disappears within 6–12 months. A few patients may be left with mild pallor of the optic disc and mild pigmentary changes in the center of the macula. The focal areas of retinitis resolve spontaneously, usually within several weeks.
Fluorescein angiography shows evidence of abnormal capillary permeability, particularly from the capillaries deep within the optic disc, not only during the early phase of the disease ( Figure 15.10 B) but even after the disc has returned to its normal appearance ( Figure 15.10 F and G). Some 10–15% of patients may show mild leakage of dye from the optic disc in the opposite eye ( Figure 15.11 D). Focal white retinal lesions, if present, usually show evidence of staining ( Figure 15.11 H and I). Angiography shows no abnormality of capillary permeability in the area of the macular star. It may demonstrate a mild window defect in the RPE, particularly in those patients following resolution of a prominent macular star. Loss of visual function primarily results from changes in the optic nerve head and not the macula. Laboratory investigations done at the time of visual loss are usually normal. Patients may show mild spinal fluid pleocytosis ( Figure 15.11 E–I).



There is enough evidence that cat-scratch disease is one of the causes of this syndrome (see Chapter 10). In one patient, Leptospira organisms were cultured from the spinal fluid.
Histopathologically, the macular star is caused by the microglial ingestion of the lipid-rich exudate lying in the outer plexiform layer of Henle. Figure 15.11 M depicts diagrammatically the probable pathogenesis of a macular star. A protein- and lipid-rich exudate leaks from the capillaries in the depth of the optic disc and extends beneath the retina in the peripapillary region as well as along the plane of the outer plexiform layer into the macular region. With reabsorption of the serous component of the exudate in the macular region the lipid and protein precipitate in the outer plexiform layer and are engulfed by macrophages. This creates the fine stellate pattern of yellow exudate that is characteristic of a macular star. Stellate maculopathy is caused by a variety of diseases affecting the permeability of the capillaries in the depth of the optic nerve head. Retinal vascular diseases usually cause a more irregular and coarser deposition of yellowish exudate in the inner nuclear as well as the outer plexiform layers in the macular region.
The differential diagnosis of patients with an optic disc swelling and a macular star includes hypertensive retinopathy (see Figures 6.25A and 6.26D), diabetic optic neuropathy and diseases associated with optic neuritis, such as sarcoidosis, bacterial septic optic neuritis, Lyme disease, and luetic optic neuritis. A macular star infrequently accompanies diffuse unilateral subacute neuroretinitis and is rarely, if ever, seen in patients with optic neuritis secondary to demyelinating diseases. The possibility of septic retinitis caused by pyogenic bacteria, cat-scratch disease, toxoplasmosis, or fungi is greater in those patients who manifest multifocal retinitis. The differential diagnosis in those presenting with a branch retinal artery or vein inclusion expands to include idiopathic recurrent branch retinal artery disease and Eales’ disease (see Chapter 6). Management of these patients depends upon the ocular findings and the presence or absence of signs and symptoms of systemic disease. Many of these patients are afebrile and the antecedent illness has resolved by the time of their eye examination. A general physical examination, routine blood counts, and serology to exclude syphilis may be all that is necessary. Those with multifocal retinitis, and particularly those who are febrile, should have appropriate evaluation including blood cultures to exclude systemic septic disease. Serologic testing for exposure to Bartonella , skin test for cat-scratch disease, and biopsy of enlarged lymph nodes may be appropriate in those patients exposed to cats. The Bartonella bacillus can be identified with the Warthin–Starry stain. The visual prognosis for patients with idiopathic stellate neuroretinitis and multifocal retinitis is good, and no treatment is required. An occasional patient will have a recurrence in the opposite eye months or years later (see Chapter 10).
Purvin and Chioran reported on seven young adults (mean age of 27 years) who developed multiple episodes of monocular neuroretinitis, macular star formation, dense arcuate visual field defects, and in some cases severe permanent visual loss. Both eyes were eventually affected in five patients. Laboratory studies were not revealing and the disorder appeared unresponsive to systemic corticosteroids. Except for the presence of a macular star, their cases seem to share more in common with AION in young patients (see discussion of idiopathic AION in the young, below).
Recurrent Optic Neuropathy Associated With Family History of Charcot–Marie–Tooth Disease
Progressive muscular weakness and atrophy that begin in the first two decades of life characterize CMT disease spectrum. CMT is a genetically heterogeneous group of diseases that accounts for almost 90% of hereditary polyneuropathies. The commonest form, CMT type 1, is an autosomal-dominantly inherited demyelinating neuropathy mapped most commonly to the short arm of chromosome 17(17p11.2) type 1A, and a few to long arm of chromosome 1(type 1B). Type 2 CMT has a similar clinical phenotype but nerve conduction is normal, suggesting the disease is neuronal rather than demyelinating. It is inherited in a dominant fashion (short arm of chromosome 1) or recessively (long arm of chromosome 8). Type 3 is the most severe form – AD linked to chromosome 1, the same locus as type 1B, and AR to chromosome 17, same as type 1A. X-linked dominant and recessive forms are also seen.
Systemic features begin with foot deformities, pes cavus, and scoliosis, followed by progressive weakening of the muscles of the leg, feet, and hands. Sensory abnormalities are few. Progressive optic atrophy that usually begins in the sixth decade or later has been reported in several pedigrees. Subclinical optic neuropathy can be detected by electrophysiologic testing in asymptomatic individuals. CMT type 6 is especially associated with optic atrophy. This 40-year-old woman had a family history of clinically diagnosed CMT disease in her brother, niece, paternal aunt, and father. She had no neurological disease, but presented with recurrent optic neuritis associated with lipid exudation that improved with systemic steroid therapy each time ( Figure 15.12 ).

Familial Dysautonomia
Familial dysautonomia (Riley–Day syndrome) is an autosomal-recessive disorder causing congenital sensory and autonomic dysfunction that affects Ashkenazi Jews exclusively.
The disease is present from birth, manifested as hypotonia and feeding difficulties resulting in frequent aspirations and pneumonias. Autonomic disturbances with lack of emotional tears, defective body temperature control, cold hands and feet, excessive sweating over head and trunk when emotionally excited, skin blotching, blood pressure lability, and orthostatic hypotension are hallmarks of the disease. There is also decreased sensitivity to pain and temperature, hypo- or areflexia, poor corneal sensation, and pupillary abnormalities. Progressive optic atrophy is seen in those patients that survive beyond early childhood.
Neuroretinopathy and Progressive Facial Hemiatrophy
Progressive facial hemiatrophy (Parry–Romberg syndrome) is a disorder of unknown cause that is characterized by progressive unilateral atrophy of the skin, muscles, and bony structures of the face, usually in preadolescent patients. It may extend down into the neck, shoulders, trunk, and extremities. All of the facial structures on one side may be involved ( Figure 15.13 D and H), or there may be only a linear depression in the scalp and forehead (coup de sabre) ( Figure 15.13 A). Vitiligo, poliosis, nevus flammeus, and moles are often present on the affected side. Ptosis, trichiasis, lagophthalmos, ectropion, neuroparalytic keratitis, canalicular obstruction, dacryocystitis, extraocular muscle palsies, enophthalmos, Horner’s syndrome, heterochromia of the iris, uveitis, optic atrophy, and pigmentary disturbances of the fundi may occur. The brain may show evidence of hemiatrophy, homolateral migraine, contralateral epilepsy, evidence of encephalitis, intracranial vascular malformations; and occasionally movement disorders may be present. This syndrome is not usually associated with visual loss. Visual loss, however, may be caused by either ipsilateral neuroretinopathy characterized by acute visual loss, optic disc swelling, peripapillary exudation, and macular star, or retinal telangiectasis and exudative retinal detachment ( Figure 15.13 A–I and see Figure 6.40I–L). Ultrasonography may demonstrate some enlargement of the affected optic nerve. The optic foramina are normal radiographically. Optic atrophy may occur as the peripapillary and macular exudation clears, but no patient has demonstrated progressive loss of visual field. Retinal vascular abnormalities have been reported previously in this disorder (see Chapter 6, Coats’ syndrome).

The pathogenesis of the acute neuroretinopathy and retinal vasculopathy is unknown. The pathogenesis of the entire disorder is complex and poorly understood. Autonomic dysfunction secondary to sympathetic abnormality as evidenced by Horner’s syndrome ; autoimmune etiology due to positive autoantibodies to double-stranded DNA, and some features common to scleroderma ; facial trauma as the inciting factor; trigeminal neuritis as evidenced by hemifacial changes; genetic etiology due to familial occurrence; developmental abnormality; possible encephalitis due to association of Rasmussen encephalitis in some have all been considered. None of these hypotheses explains all the manifestations seen in the condition. It is best considered a disorder of clinical heterogeneity likely caused by etiological heterogeneity.
Anterior Ischemic Optic Neuropathy
The term “anterior ischemic optic neuropathy” is used to describe swelling, ischemia, and varying degrees of infarction of the anterior part of the optic nerve caused by reduction in blood flow to the nerve ( Figures 15.14 and 15.15 ). The optic nerve head, by virtue of its closely arranged nerve fibers within the nonexpansile intrascleral canal, is ideally situated for ischemia to occur. Primary vascular insufficiency or secondary vascular insufficiency caused by any process that promotes stasis of axoplasmic flow and nerve fiber swelling can cause ischemia. AION typically affects older patients, and the acute loss of vision may be mistakenly attributed to a macular disorder, for example, macular degeneration if pigment epithelial changes are present, or to cystoid macular edema in the aphakic patient ( Figure 15.14 K and L). For pathogenetic as well as therapeutic reasons, these patients can be subdivided into two major subgroups: (1) a nonarteritic group, n-AION (those without evidence of arteritis, 95%) and (2) an arteritic group, a-AION (those with giant-cell arteritis, 5%). The nonarteritic group may be subdivided into an idiopathic group, those with no identifiable cause, and those with a probable cause. Functionally, ophthalmoscopically, and fluorescein angiographically, all subgroups may present similar findings. The visual prognosis, however, is not the same for all groups.


Idiopathic (“Nonarteritic”) Anterior Ischemic Optic Neuropathy
Over 50% of patients with n-AION are generally healthy patients whose age is 45 years or older (mean of 57–65 years) and who experience an acute, usually moderate loss of vision in one eye (20/50 to 20/200) over hours to days. Unlike patients with optic neuritis or arteritic AION (a-AION), pain and headache are not features. About 10% report a minor discomfort. Less than 5% report prodrome such as intermittent transient visual blurs, shadows, or spots that more often accompany a-AION. Optic disc swelling accompanied by one or several flame-shaped hemorrhages is usually evident ( Figure 15.14 ). The swelling may or may not be pallid. It may be more pronounced in the superior half of the optic disc. A lower altitudinal or arcuate field defect that often is maximum at the time of presentation but that may progress during the first few weeks of the disease is present. Occasionally hard exudates and cotton-wool spots are present (7%). Fluorescein angiography usually demonstrates a delay of perfusion of the optic disc blood vessels, minimal alterations of choroidal filling, and staining of the optic disc ( Figure 15.14 B and C). In some cases there is a delay in the retinal artery appearance time, and an increased retinal circulation time, that presumably is caused by compression of the central retinal vessels by the swollen ischemic nerve fibers in the region of the lamina cribrosa. This compression occasionally may be sufficient to cause a fundus picture of central retinal artery or vein occlusion ( Figure 15.14 A–C). Occasionally the compression could occlude a cilioretinal artery or a branch retinal artery coming off the disc ( Figure 15.14 G–I). Focal telangiectatic changes may appear on the surface vessels of the disc within a few days, considered to be luxury perfusion due to autoregulatory response by increased perfusion surrounding an infarct. Sometimes this may be interpreted as a capillary hemangioma or neovascularization when prominent ( Figure 15.14 K). The disc swelling resolves in several weeks. The disc becomes pale and usually shows minimal cupping. Spontaneous improvement of visual acuity occurs in 10–35% of cases. Recurrence of n-AION in the same eye is infrequent. Possible explanations for the infrequent recurrences in n-AION include loss of nerve fibers after n-AION, providing more space for surviving nerve fibers to swell, and shunting of blood from the area of infarction to the surviving part of the nerve. Approximately 40% of patients will develop n-AION in the opposite eye. Although it has been presumed that most of these patients probably have an arteriosclerotic disorder, there is minimal evidence to support this view. These patients have no greater incidence of cardiovascular or cerebrovascular disease than a matched group of patients. Idiopathic or n-AION occurs at a younger age in smokers (mean age 51 years) compared to nonsmokers (mean age 64 years). The frequency of involvement in the upper half of the optic disc, the rarity of second attacks of AION in the same eye, and the small cup/disc ratio suggest that structural factors are important in the pathogenesis of idiopathic AION.
Associated disorders that may precipitate nonarteritic AION include diabetes, malignant hypertension ( Figure 15.15 A and B), uremia, eclampsia, migraine, embolism, hemodynamic shock, anemia, papilledema, orbital inflammation ( Figure 15.14 A–C), cataract extraction ( Figure 15.13 K and L), elevation of intraocular pressure, congenital anomalies of the optic disc, and optic disc drusen. Subretinal neovascularization occasionally occurs. Familial n-AION has been reported. HLA-A29 may be a risk factor for development of n-AION.
Histopathologic evidence suggests that vascular insufficiency causing acute ischemic swelling of a segment of the nerve fibers immediately posterior to the lamina cribrosa is responsible for the clinical picture of n-AION. Vasculopathy of the paraoptic branches of the short posterior ciliary arteries may play a major role. The reason for the susceptibility of the superior segments of the optic nerve to ischemic damage and the role of nocturnal hypotension and sleep apnea are uncertain.
Additional factors that might affect perfusion such as optic disc drusen, hyperopia, elevated intraocular pressure, migraine, and drugs such as sildenafil and interferon-alpha may have a role in some cases. Amiodarone may itself cause toxic optic neuropathy or trigger an ischemic optic neuropathy in susceptible individuals, hence a history of its use should be sought. There is no proven treatment for n-AION; aspirin is recommended for its role in decreasing strokes and myocardial infarctions in this vasculopathic population.
Idiopathic Anterior Ischemic Optic Neuropathy in the Young (AIONY)
Idiopathic AIONY is a rare entity characterized by recurrent episodes of acute visual loss associated with segmental pallid swelling of the optic nerve that frequently causes severe and permanent visual loss in otherwise healthy young adults with a mean age of onset of 25 years. The fundus picture and visual field changes during the acute stage are identical to those in n-AION. This similarity includes the small optic disc size. Medical evaluation is negative. The cause is unknown.
It is important therapeutically to differentiate n-AION and AIONY from a-AION. The blood sedimentation rate, acute-phase reactants such as C-reactive proteins, and platelet counts are usually within normal limits in n-AION and AIONY and are usually greatly elevated in most cases of a-AION.
There is no effective treatment for n-AION or AIONY. Optic nerve sheath decompression was suggested as effective in the treatment of the acute progressive stage of n-AION. Evidence, however, including the results of a controlled clinical trial, indicates that the procedure is ineffective and may be harmful for the treatment of n-AION. The rate of operative complications, which include central retinal artery occlusion associated with optic nerve sheath decompression, may be as high as 40%. Treatment of the associated systemic disorders in patients with nonarteritic AION, for example, corticosteroids or X-ray irradiation in orbital inflammatory disease ( Figure 15.14 A–C), blood transfusion for patients with anemia, or hemodialysis in patients with uremia, may improve the prognosis for return of vision. Patients with evidence of a-AION require prompt treatment with high doses of corticosteroids (see next subsection).
Arteritic Anterior Ischemic Optic Neuropathy (a-AION)
Giant-cell arteritis (temporal arteritis, cranial arteritis) is a systemic disease that is characterized by arthralgias, headaches, fever, weight loss, jaw claudication, myalgia, and, frequently, acute severe visual loss in one eye that is often followed by severe loss in the second eye in elderly patients, usually 60 years of age or older. Ischemic necrosis of the scalp may occur in severe cases; often these patients are also irritable due to the myalgia in the examining chair. The visual loss is generally more profound than in n-AION, and the optic disc pallor is usually more striking ( Figure 15.15 D and F and Figure 6.23L). Cotton-wool patches and flame-shaped hemorrhages may be present. Other findings may include hypotony, extraocular muscle palsies, and central retinal arterial as well as choroidal arterial occlusion (see Figure 6.23). Fluorescein angiography demonstrates delayed perfusion of the choroid and optic disc ( Figure 15.15 G and H). The Westergren sedimentation rate is usually 100 mm or greater; C-reactive proteins and platelets are elevated. Increased plasma viscosity, decreased red cell filterability, and decreased hematocrit are other findings in these patients. The sedimentation rate and temporal artery biopsy findings are important in differentiating a-AION from n-AION. Occasionally there may be a delay in the rise of the sedimentation rate, hence a high index of suspicion by positive review of systems warrants a repeat check 1–2 days later. Biopsy of the temporal artery shows infiltration and thickening of the media and proliferation into the lumen thus obliterating it, resulting in vascular insufficiency ( Figure 15.15 I and J). Systemic corticosteroids should be instituted promptly if giant-cell arteritis is suspected. Monitoring changes in the blood sedimentation rate or C-reactive protein is used to adjust the corticosteroid dosage, which should be continued for 2 years or more. The goal of steroid therapy is to prevent loss of vision in the fellow eye and involvement of other cranial arteries.
Development or progression of visual loss occurs rarely in patients with giant-cell arteritis after the initiation of glucocorticoid therapy. In a retrospective study of 245 patients with giant-cell arteritis seen over a 5-year period at the Mayo Clinic, Aiello and coworkers found that 14% had permanent loss of vision in one or both eyes. In all but two of the patients, visual loss occurred before institution of corticosteroid therapy. Visual loss progressed after corticosteroid therapy in three patients. After 5 years the probability of developing visual loss after initiation of oral glucocorticoid treatment was determined to be 1% (Kaplan–Meier), and the probability of additional loss in patients who had a visual deficit at the time therapy was begun was 13%.
Other rare causes of a-AION are periarteritis nodosa, Churg–Strauss syndrome, Wegener’s granulomatosis, systemic lupus erythematosus, rheumatoid arthritis, and relapsing polychondritis. These conditions should be considered in patients who present earlier than 60 years of age or if review of systems points to any of them.
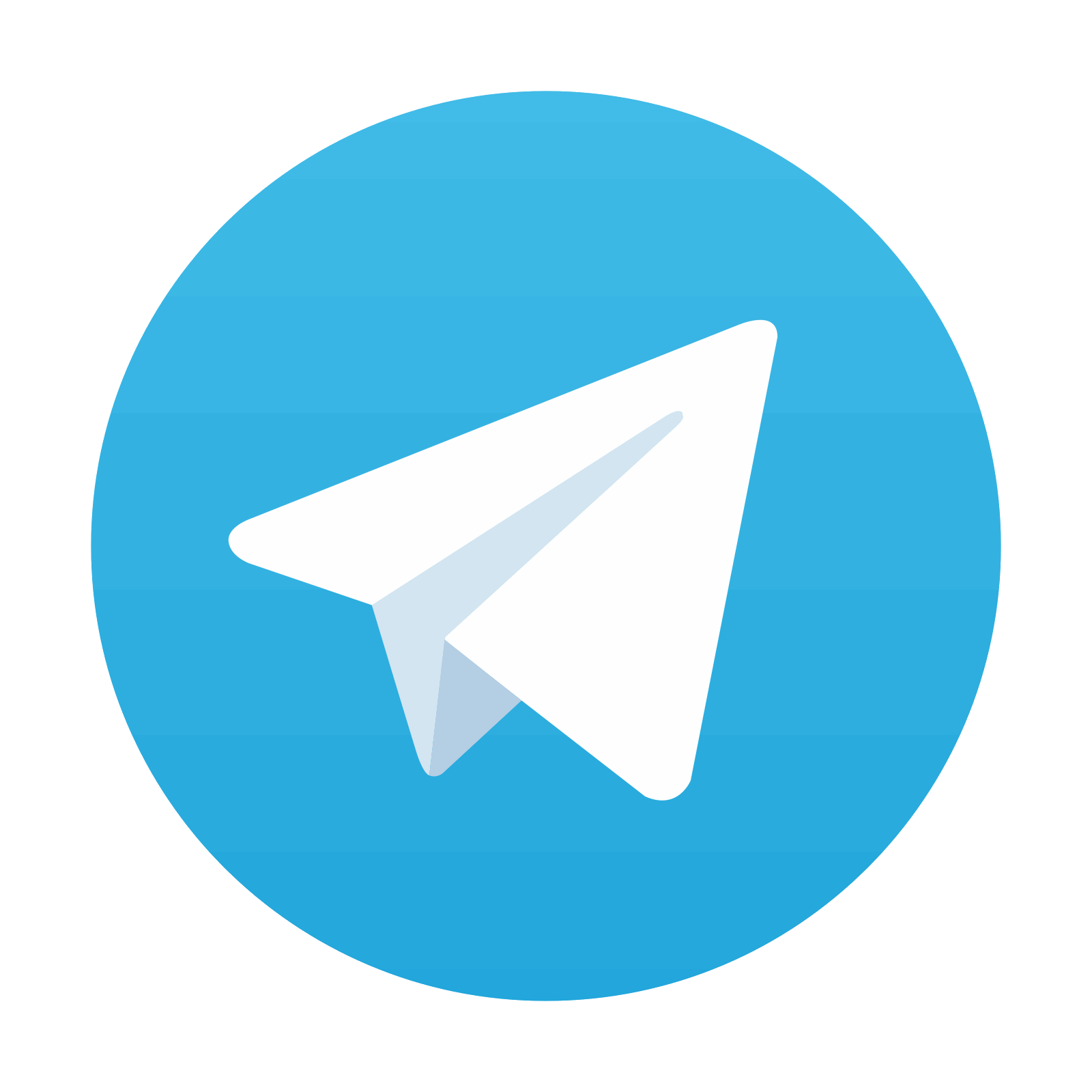
Stay updated, free articles. Join our Telegram channel

Full access? Get Clinical Tree
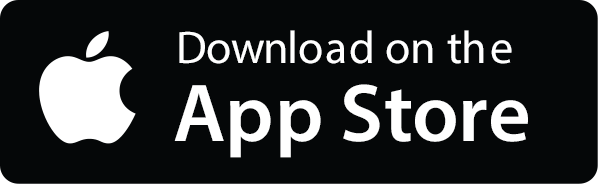
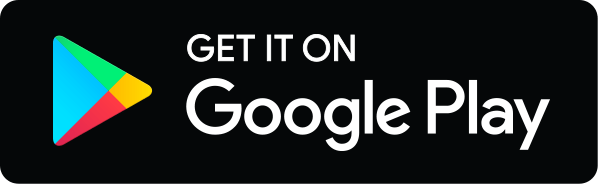