Key points
- •
The inner ear has several structural and functional characteristics that make it an appealing target for novel gene therapy, RNA-based therapy, and stem cell therapy for treatment of sensorineural hearing loss (SNHL).
- •
The most rapid advancements have been in gene therapy; for example, a recent phase 1/2 clinical trial uses adeno-associated virus (AAV)-mediated delivery of atonal homolog 1 ( Atoh1 ) to promote hair cell regeneration.
- •
Further research will be aimed at optimizing gene therapy expression and timing of delivery, promoting appropriate connectivity between engineered and endogenous cells, and exploring alternative techniques, such as genomic editing.
AAV | Adeno-associated virus |
ABR | Auditory brainstem response |
ASO | Antisense oligonucleotide |
Atoh1 | Atonal homolog 1 |
BDNF | Brain-derived neurotrophic factor |
DFNA | Nonsyndromic deafness, autosomal dominant |
DFNB | Nonsyndromic deafness, autosomal recessive |
FGF | Fibroblast growth factor |
GDNF | Glial cell line–derived neurotrophic factor |
GJB2 | Gap junction beta-2 |
HSV1 | Herpes simplex virus 1 |
MYO7A | Myosin VIIA |
NT-3 | Neurotrophin 3 |
ONP | Oticlike neural progenitors |
RNAi | RNA interference |
SGN | Spiral ganglion neuron |
SNHL | Sensorineural hearing loss |
USH1C | Usher syndrome 1C |
Introduction
Hearing loss is the most common sensory deficit in developed societies. According to the Centers for Disease Control, 2 to 3 children out of every 1000 births have hearing loss. Hearing impairment in children, particularly of prelingual onset, has been shown to negatively affect educational achievement, future employment and earnings, and even life expectancy.
SNHL, which refers to defects within the cochlea or auditory nerve itself, far outweighs conductive causes for permanent hearing loss in both children and adults. The causes of SNHL in children are heterogeneous, including both congenital and acquired causes. In neonates in particular, a genetic cause accounts for half of all cases of hearing loss. Of those with genetic causes, 30% can be considered syndromic (eg, Pendred, Usher, Waardenburg) and the other 70% nonsyndromic. The inheritance pattern of nonsyndromic SNHL follows this general distribution: 75% autosomal recessive, 20% autosomal dominant, 2%–5% X-linked, and less than 1% mitochondrial. The mode of inheritance is important, as it guides the strategy of intervention, as explained in greater detail later.
The process of auditory transduction, in which mechanical sound waves are converted to electrical inputs, occurs at the level of the hair cell within the cochlea. The hair cell, located in the organ of Corti in mammals, contains specialized structures that project from the apical surface into the scala media and sense waves within the endolymph. The movement of these projections converts sound waves into electrical signals, which are subsequently carried via spiral ganglion neurons (SGNs) to the eighth cranial nerve. At any point along this pathway, a disruption in the conversion of mechanical signals to electrical signals propagated to the auditory nucleus results in SNHL. This article identifies potential mechanisms of intervention both at the level of the hair cell and the SGNs.
Approximately 16,000 hair cells are produced during early development in each cochlea, along with supporting cells and 30,000 to 40,000 afferent SGNs. However, the fragile nature of hair cells makes them susceptible to damage and death because of genetic factors, exposure to noise, ototoxic drugs, and even early infection. Once damaged, these cells cannot be restored. However, since the discovery was made that avian species can spontaneously regenerate new hair cells after damage, translational research has been aimed at understanding this process so as to initiate a similar one in the human ear.
Current treatment of SNHL cannot improve any cellular deficits; sound is either amplified with a hearing aid or the auditory nerve is stimulated with a cochlear implant. Both strategies use the remaining hair cells or auditory neurons. Yet, in recent years, new evidence suggests that future treatments may be able to improve SNHL at the cellular level through modification of hair cells or auditory neurons. These possible treatments follow 3 main approaches: (1) gene therapy to augment production of proteins that may protect or even regenerate hair cells, (2) RNA-based therapy to inhibit expression of detrimental proteins that promote hair cell damage, and (3) stem cell therapy to replace damaged or dead hair cells or auditory neurons. Although it is unlikely that any of these strategies will be a panacea for SNHL, they will add to the armamentarium of treatment options that can serve an individual patient.
Introduction
Hearing loss is the most common sensory deficit in developed societies. According to the Centers for Disease Control, 2 to 3 children out of every 1000 births have hearing loss. Hearing impairment in children, particularly of prelingual onset, has been shown to negatively affect educational achievement, future employment and earnings, and even life expectancy.
SNHL, which refers to defects within the cochlea or auditory nerve itself, far outweighs conductive causes for permanent hearing loss in both children and adults. The causes of SNHL in children are heterogeneous, including both congenital and acquired causes. In neonates in particular, a genetic cause accounts for half of all cases of hearing loss. Of those with genetic causes, 30% can be considered syndromic (eg, Pendred, Usher, Waardenburg) and the other 70% nonsyndromic. The inheritance pattern of nonsyndromic SNHL follows this general distribution: 75% autosomal recessive, 20% autosomal dominant, 2%–5% X-linked, and less than 1% mitochondrial. The mode of inheritance is important, as it guides the strategy of intervention, as explained in greater detail later.
The process of auditory transduction, in which mechanical sound waves are converted to electrical inputs, occurs at the level of the hair cell within the cochlea. The hair cell, located in the organ of Corti in mammals, contains specialized structures that project from the apical surface into the scala media and sense waves within the endolymph. The movement of these projections converts sound waves into electrical signals, which are subsequently carried via spiral ganglion neurons (SGNs) to the eighth cranial nerve. At any point along this pathway, a disruption in the conversion of mechanical signals to electrical signals propagated to the auditory nucleus results in SNHL. This article identifies potential mechanisms of intervention both at the level of the hair cell and the SGNs.
Approximately 16,000 hair cells are produced during early development in each cochlea, along with supporting cells and 30,000 to 40,000 afferent SGNs. However, the fragile nature of hair cells makes them susceptible to damage and death because of genetic factors, exposure to noise, ototoxic drugs, and even early infection. Once damaged, these cells cannot be restored. However, since the discovery was made that avian species can spontaneously regenerate new hair cells after damage, translational research has been aimed at understanding this process so as to initiate a similar one in the human ear.
Current treatment of SNHL cannot improve any cellular deficits; sound is either amplified with a hearing aid or the auditory nerve is stimulated with a cochlear implant. Both strategies use the remaining hair cells or auditory neurons. Yet, in recent years, new evidence suggests that future treatments may be able to improve SNHL at the cellular level through modification of hair cells or auditory neurons. These possible treatments follow 3 main approaches: (1) gene therapy to augment production of proteins that may protect or even regenerate hair cells, (2) RNA-based therapy to inhibit expression of detrimental proteins that promote hair cell damage, and (3) stem cell therapy to replace damaged or dead hair cells or auditory neurons. Although it is unlikely that any of these strategies will be a panacea for SNHL, they will add to the armamentarium of treatment options that can serve an individual patient.
Gene therapy for sensorineural hearing loss
Conceptually speaking, gene therapy involves the transfer of a gene of interest to a target cell. This process can be accomplished through 2 main mechanisms: recombinant viruses and nonviral methods, which include liposomes and naked DNA. In the case of virally mediated gene therapy, the desired gene is packaged into a viral vector and the virus subsequently transfects the target cell and incorporates itself, including the desired gene, into the target cell’s DNA. At this point, the target cell produces the protein of interest using its native translation machinery. Thus, long-term expression of a transgenic protein can be induced. Given the right promoter sequences, the target cell can be programmed to express the protein indefinitely or it can be situationally regulated. The alternative to gene therapy is direct administration of proteins, which are limited in their efficacy by their short half-lives and often require the use of continuous pumps.
Several engineered replication-deficient viruses have been used for transfection of a variety of cell types in the nonprimate mammalian inner ear, including adenovirus, lentivirus, herpes simplex virus 1 (HSV1), vaccinia virus, and AAV. Each of these vectors demonstrates a unique profile of strengths and weaknesses with regard to cell types transfected, efficiency of transfection, genetic carrying capacity, and potential side effects ( Table 1 ; for review, see Ref. ). Adenovirus, a 36-kbp double-stranded DNA virus, has been found to be particularly suited for use in SNHL because of its large carrying capacity of up to 10 kbp, ease of production, and ability to efficiently transfect both hair cells and supporting cells as well as neural and glial cells. For these reasons, the first gene therapy clinical trial for SNHL used an adenoviral vector.
Mechanism of Delivery | Advantages | Disadvantages | Cellular Tropism |
---|---|---|---|
Viral | |||
Adenovirus | Easy to produce Large insert (7.5–10 kb) Cell division not required | Immunogenic Transient expression | Mesenchymal cells lining perilymphatic spaces Stria vascularis Fibrocytes of spiral ligament Supporting cells of OC Hair cells of OC and vestibule SGNs |
Adeno-associated virus | No human disease Stable expression Cell division not required | Difficult to produce Small insert (4–5 kb) Variable transfection efficiency | Fibrocytes of SL Rm Supporting cells of OC SGNs Vestibular sensory hair cells and supporting cells |
Herpes virus | Large insert (10–100 kb) Stable expression | Human disease Cytopathic | Mesenchymal cells lining perilymphatic spaces Fibrocytes of SL Rm Supporting cells of OC SGNs VGNs |
Lentivirus (retrovirus) | Stable expression | Insertional mutagenesis Low transfection efficiency Cell division required | SGNs Glia |
Nonviral | |||
Liposome | Easy to make Unlimited insert size Cell division not required Nonpathogenic Nonimmunogenic | Transient expression Low transfection | Fibrocytes of SL OHCs and SCs of OC Rm SGNs |
The anatomy of the inner ear is unique and offers several advantages and disadvantages for the advancement of gene therapy treatment. The sensory organ is housed in bone and bathed in fluid, thereby protecting it and facilitating access. The presence of the blood-labyrinthine barrier is thought to prevent the dissemination of viral vector into the systemic circulation, thereby limiting potential side effects of the virus itself as well as reducing the risk of inappropriate gene insertion at distant sites. This protection also works the other way: the barrier prohibits interference by the immune system on exogenous cells or viral vectors. Two injection sites are available for access depending on the strategy of interest: the round window provides access to the fluid surrounding the hair cell bodies, whereas a basal turn cochleostomy can be used for the hair cell bundles ( Fig. 1 ). Although both approaches showed expression of a green fluorescent protein reporter gene using an advanced-generation adenoviral vector in a guinea pig model, the basal turn cochleostomy group demonstrated high-frequency hearing loss, whereas the round window group preserved hearing.
The small volume and small number of target cells that comprise the inner ear form the ideal scenario for gene therapy. The circumstances promote high concentrations of viral vector with few cells requiring transfection combined with a continuous flow of fluid throughout all segments of the inner ear. However, despite the continuity between chambers, studies have shown a transfection gradient after round window injection into the scala tympani, which occurs with multiple vector types, including AAV, cationic liposome, HSV1, and vaccinia virus vectors. Because the volume is constrained, the injection of additional fluid into the cochlea may disrupt the tight junctions separating the endolymph and perilymph, resulting in loss of the endolymphatic potential through mixing of fluids, ultimately causing hair cell death. Furthermore, future therapies targeting endolymphatic delivery of vectors will have to adapt to the high potassium content of the fluid, making the preparation substantially different from those intended for other delivery sites.
There are 3 key ways in which gene therapy has been hypothesized to improve the function of the inner ear. In acquired hearing loss, gene therapy can be used to provide protective factors that promote SGN survival in the absence of healthy hair cells. Similarly, it can be used to provide protective factors to prevent hair cell damage at the outset from chemical or noise-induced insult. In these 2 examples, the results of gene therapy are supportive in nature, with the goal of preventing hearing loss. Gene therapy has also been used as a means of growth and replacement after hearing loss. Some research groups are seeking to use gene therapy to promote hair cell rescue or regeneration to increase the population of remaining cells. The same strategies have also been used in congenital hearing loss and include attempts to transform surrounding cells into expressing a hair cell–like phenotype.
Spiral Ganglion Neuron Survival
In the healthy ear, inner ear cells, including hair cells, secrete multiple neurotrophic factors. Both neurotrophin 3 (NT-3) and brain-derived neurotrophic factor (BDNF) have been associated with the maintenance of functional SGNs, which can be damaged through lack of adequate trophic support or through direct trauma to the afferent fibers or cell bodies. Thus, hair cell loss negatively affects the survival of SGNs via the absence of these key trophic factors. Although direct infusion of NT-3 and BDNF into the cochlea has been shown to improve SGN survival significantly, the requirement of an osmotic mini pump is impractical for clinical use. However, given that the hearing benefits of cochlear implants depend on functional SGNs and the auditory nerve, the question of how to provide sustained delivery of these trophic factors is of high importance. Gene therapy may prove to be a means to accomplish this goal.
Gene therapy has been successfully used to increase BDNF expression after hair cell loss using multiple viral vectors, including HSV and AAV in an aminoglycoside-deafening regimen and adenovirus after a deafening regimen in guinea pigs, thereby increasing the versatility of this potential strategy. As several viral vectors have been compatible with this strategy, its versatility is a further potential benefit.
In addition, studies have demonstrated that glial cell line–derived neurotrophic factor (GDNF) can have protective effects for SGNs. Both direct application of GDNF to the cochlea in vitro as well as GDNF intratympanic infusion to the middle ear in vivo improved SGN survival in an ototoxic deafening model in guinea pigs. Again, enhanced survival was seen after adenoviral-GDNF treatment in a similar guinea pig ototoxic model, and interestingly, adenoviral-GDNF therapy combined with electrical stimulation was more effective at promoting SGN survival than either treatment alone. These findings support the hypothesis that gene therapy may be best used as an adjunct to, instead of as a replacement for, other types of existing SNHL treatment.
Although most of these studies have tested trophic replacement in acquired SNHL models, BDNF supplementation has also been found to improve SGN survival in nonsyndromic SNHL. Mutations in the gene POU4F3 in humans are responsible for DFNA15, a progressive nonsyndromic SNHL. Although the mutations are autosomal dominant, the phenotypic presentation varies greatly in age of onset and degree of hearing loss. Unlike the human mutation, the Pou4f3 mutation in mice is recessive and more uniform in phenotype, resulting in SGN loss at 2 to 6 weeks of age. Fukui and colleagues treated Pou4f3 mutant mice with an adenoviral-BDNF vector. Treated mice were found to have significantly more viable SGNs than controls, and the surviving SGNs demonstrated substantial sprouting to the auditory epithelium.
Hair Cell Survival
The neurotrophic factors that have been shown to improve SGN survival, namely, NT-3, BDNF, and GDNF, have also been shown to promote hair cell survival. In a gene therapy study with pharmacologic ototoxicity, an adenoviral-GDNF construct protected both vestibular and cochlear hair cells, which ultimately rescued hearing function. In addition, damage caused by reactive oxygen species has been implicated as a mechanism of hair cell damage in acquired SNHL, including aminoglycoside- and noise-induced hearing loss. Adenoviral vectors with catalase and Mn superoxide dismutase inserts curbed hearing loss when administered prophylactically in a model of aminoglycoside toxicity.
Genetic Hearing Loss
Of particular interest in the pediatric population are gene therapy treatments for genetic hearing loss. As mentioned earlier, gene therapy can replace a defective allele with its wild-type counterpart and is a compelling strategy for treatment of patients with these types of diseases. Genetic causes for deafness are divided into 2 categories: (1) syndromic, in which deafness is associated with other deficits, and (2) nonsyndromic, in which deafness is the only deficit.
One of the well-studied syndromic causes of hearing loss is Usher syndrome, a disease characterized by congenital deafness, progressive vision loss, and vestibular dysfunction. The most common gene associated with profound congenital SNHL is Usher syndrome type 1B, in which mutations in myosin VIIA ( MYO7A ) cause the production of an atypical myosin in the sensory cells of the ears and eyes, resulting in retinitis pigmentosa and hearing loss. A phase 1/2 clinical trial initiated in 2013 is underway to test the effect of delivery of a corrected version of MYO7A on vision loss, based on the successful results of this approach in a mouse model. Thus, in the future, it may be plausible to use a similar approach to treating the hearing loss caused by the same defective protein. In a related mouse model of Usher syndrome 1C (USH1C), Lentz and colleagues were able to restore hearing and vestibular function using injections of antisense oligonucleotides (ASOs). The therapy reduced production of mutated harmonin in favor of translation of the wild-type protein. Further discussion of the strategy and mechanism are described in the RNA-based therapy section.
Nonsyndromic hearing loss is heterogeneous in inheritance with 32 autosomal dominant genes associated with 29 loci (DFNA1–DFNA67) and 60 autosomal recessive genes associated with 57 loci (DFNB1–DFNB103). Mutations in the gap junction beta-2 ( GJB2 ) gene, located at the DFNB1A locus, account for 20% to 50% of congenital recessive nonsyndromic SNHL, depending on the population. The GJB2 gene encodes connexin-26, a protein found in the supporting cells of the inner ear whose aggregates form gap junctions. These gap junctions are thought to facilitate potassium circulation between the hair cells and the stria vascularis during mechanosensory transduction. Without proper potassium recirculation, the endolymph potential decays, and hearing loss ensues. Encouraging evidence from in vitro and in vivo studies shows that gene transfer of GJB2 can restore expression of connexin-26 and even functional gap junctions. However, improvement in hearing has yet to be demonstrated in an animal model.
Hair Cell Regeneration and Supporting Cell Transformation
Although the aforementioned strategies seem more conducive to the treatment of congenital hearing loss, gene therapy also shows potential for the improvement of acquired hearing loss. Even in the pediatric population, SNHL can be attributed to infection (both viral and bacterial), acoustic trauma, or pharmacologic toxicity from aminoglycoside antibiotics, oncologic chemotherapy, or chelation therapy. These insults have the capacity to damage delicate hair cells, which, unlike those of avian species, do not spontaneously regenerate. However, recent research of these lower vertebrates capable of hair cell regeneration has led to strategies that may have the potential to promote the regeneration of hair cells themselves or to trigger differentiation of supporting cells to assume a hair cell–like phenotype.
For example, various groups have been able to generate hair cells by transfecting endogenous supporting cells with regulatory proteins that are normally found earlier in development. Atoh1 , also referred to as Math1/Hath1 (mouse atonal homologue 1/human atonal homologue 1), is a basic helix-loop-helix transcription factor required for normal hair cell differentiation in the developing ear. Initial in vitro studies by Zheng and Gao induced overexpression of murine Atoh1 , which resulted in ectopic hair cell production. Subsequently, the team used an adenoviral vector to overexpress human Atoh1 , which generated increased numbers of hair cells both in a rat utricular macula model as well as after aminoglycoside injury.
In in vivo studies, transfection of Atoh1 into supporting cells has been shown to generate hair cells in a deafened guinea pig model, leading to lower thresholds on auditory brainstem responses (ABRs) than in deafened controls. In an embryonic mouse model, a similar protocol showed generation of not only new hair cells from supporting cells but also new hair cells that could support mechanosensory function. An adenoviral-packaged Atoh1 delivery was found to increase hair cell numbers in the macular organs of an aminoglycoside-treated mouse model with resulting improvement in balance. In more recent studies, increased expression of Atoh1 has been shown to be protective of hair cells by means of induction of repair processes after noise-induced hearing loss. The therapeutic window in which recovery can be maximized has yet to be determined and may likely require initiating therapy within a short time of injury.
The first clinical trial of gene therapy for hearing loss incorporates adenoviral delivery of Atoh1 to the inner ear in adult patients to treat auditory and vestibular dysfunction. The clinical trial of the experimental drug CGF166, which is a collaboration between Novartis (Basel, Switzerland) and GenVec (Gaithersburg, Maryland), received approval of its Investigational New Drug application to the US Food and Drug Administration in 2014 and is currently in phase 1/2. Although this clinical trial is approved only for adults older than 20 years who have noncongenital, severe to profound hearing loss, there is potential for the same treatment to be used in pediatric patients with acquired SNHL in the future.
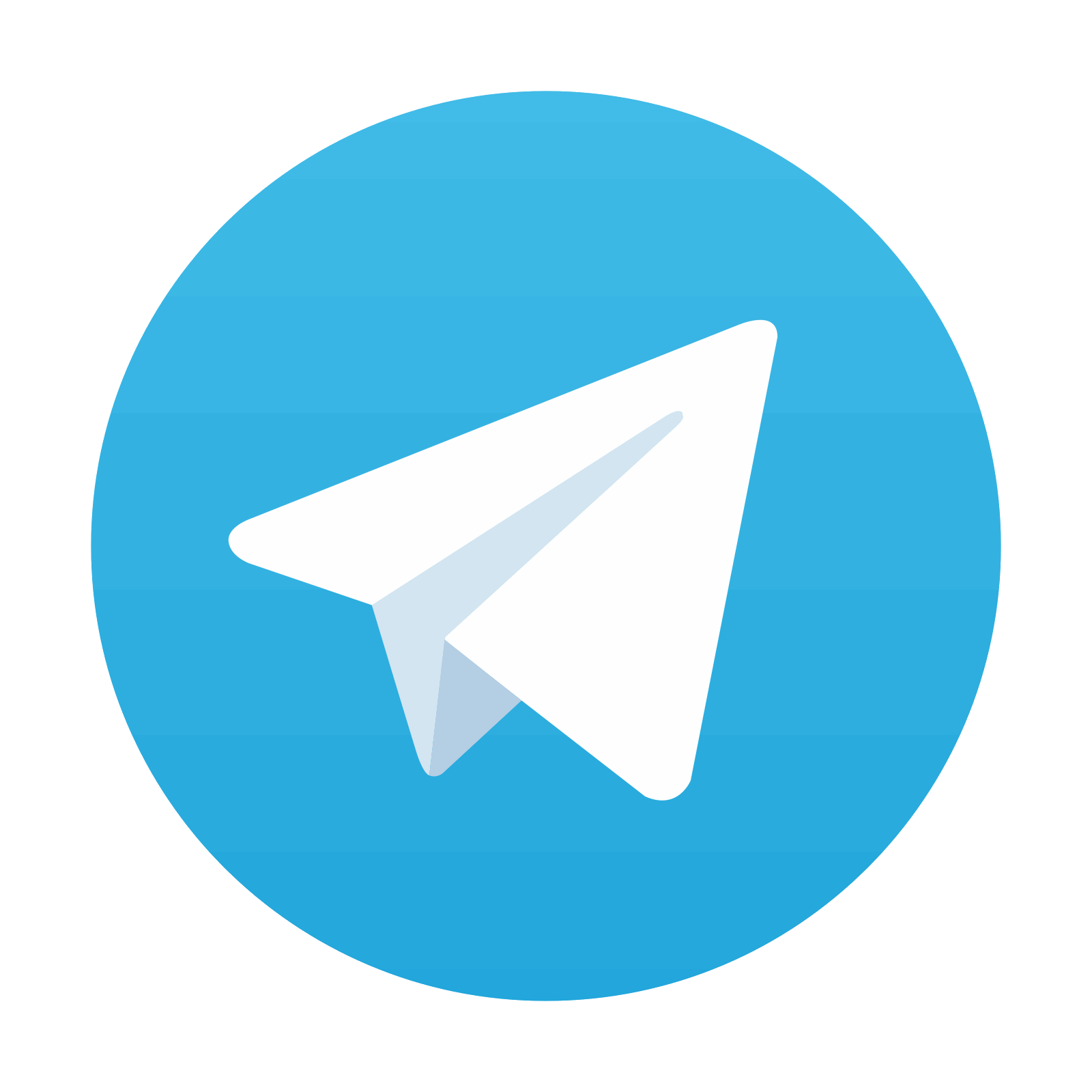
Stay updated, free articles. Join our Telegram channel

Full access? Get Clinical Tree
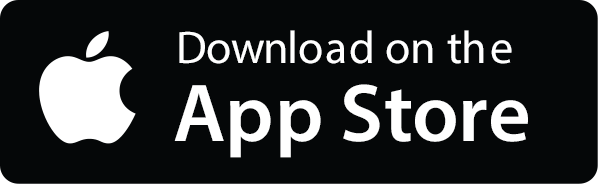
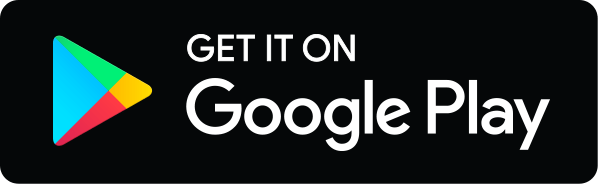