Purpose
To determine the ability to detect normal vitreous structure, evolving posterior vitreous detachment (PVD), and related vitreoretinal changes with combined spectral-domain optical coherence tomography (SD-OCT) and scanning laser ophthalmoscopy (SLO).
Design
Observational cross-sectional study.
Methods
Simultaneous SD-OCT and SLO imaging instruments (SD-OCT/SLO) were used to image both eyes of patients with symptoms of PVD. The vitreous cortex, preretinal lacunae, hyaloid, and its relations to the retinal surface were analyzed. In addition, ultrasound was performed in a subset of patients to determine the stage of PVD.
Results
Two-hundred two eyes of 113 subjects were scanned. There was a high correlation between diagnosis of complete PVD by clinical examination and OCT (95 vs 93 eyes, respectively; κ, 0.82). A partial PVD was detected more frequently by SD-OCT/SLO than by biomicroscopy examination (45 vs 7 eyes; P < .0001). Ultrasound was performed in a subset of 30 eyes. A high agreement was found between ultrasound and SD-OCT/SLO results for both complete PVD (κ, 0.933) and incomplete PVD (κ, 0.91). Vitreous cortex was detected in 181 eyes, and posterior precortical vitreous pocket was detected in 85 eyes. The effects of PVD, including vitreoretinal traction, paravascular lamellar holes, and fine changes at the fovea, could be visualized reliably in detail only with SD-OCT/SLO. In all these eyes, SD-OCT/SLO allowed improved visualization of the vitreoretinal relationship.
Conclusions
SD-OCT/SLO provides unprecedented in vivo information about the physiologic and pathologic vitreous structure; it allows an extremely detailed analysis of the vitreoretinal interface, and it is particularly useful for defining focal changes and PVD.
Standard time-domain optical coherence tomography (TD-OCT) has been used widely to analyze the relationship between the vitreous and the retina in healthy aging eyes as well as in eyes affected by retinal diseases. During aging, biochemical and structural changes occur in the corpus vitreous, leading to posterior vitreous detachment (PVD). Although generally benign, PVD contributes to significant retinal morbidities, such as vitreomacular traction syndrome, macular holes, epiretinal membranes, and retinal tears. Furthermore, vitreous status plays a crucial role in the natural course of preexisting local and systemic conditions (i.e., uveitis and diabetes), significantly affecting their prognosis and management.
Although ultrasound has been considered the standard imaging technique to detect and document vitreous detachment, the higher resolution of OCT offers advantages when studying any intermediate step of this process. TD-OCT studies have illustrated the posterior hyaloid and its adhesions to the retinal surface in vitreomacular traction syndrome (VMT) and related diseases.
The recent introduction of spectral-domain (SD) OCT technology allows better visualization of the hyaloid and a more detailed image of its structure than time-domain OCT. The coupling of this technology with scanning laser ophthalmoscopy (SLO) images allows true simultaneous visualization of the retinal surface and OCT images, leading to a higher level of confidence while localizing clinically relevant details acquired with the OCT. Also, combined SD-OCT and SLO (SD-OCT/SLO) allows rapid, precise focusing from the deep retinal planes to the mid vitreous cavity. Using these advantages, we explored the use of SD-OCT/SLO in imaging the vitreous architecture. Our goal was to visualize for the first time in vivo details of the vitreous structure in different stages of its physiologic aging process, highlighting its relationship with the retina not only at the fovea, but beyond the posterior pole.
Methods
A consecutive series of patients referred to the Jacobs Retina Center at the University of California, San Diego, in La Jolla, California, were scanned with SD-OCT/SLO. This was an observational cross-sectional study. All patients had symptoms suggestive of either recent or prior PVD, such as large floaters or a history of recent or acute and resolved photopsia or recent increase in floaters in at least 1 eye. The fellow eyes of these patients also were included in the study regardless of symptoms. Before the imaging session, all the patients underwent a complete ophthalmologic examination, visual acuity (VA) testing with standardized best-corrected refraction, and slit-lamp biomicroscopy performed in mydriasis by a retina specialist using either a 78- or a 90-diopter indirect lens. Clinical PVD was diagnosed when either a Weiss ring or the clearly defined posterior hyaloid membrane were detected floating in the vitreous cavity. Eyes with media opacity severe enough to prevent a good visualization of the fundus and vitrectomized eyes were excluded from the study.
All the eyes were imaged by trained operators (F.M., M.B., R.M.Y.) using a SD-OCT coupled with a simultaneous SLO device. One of 2 different machines was used for vitreous imaging according to instrument availability at the time the patients were studied. The first was a prototype SD-OCT/SLO (OCT SLO, software version 1.66; Opko Instrumentation/OTI, a division of OPKO Health, Miami, Florida, USA): this instrument uses a modified superluminescent diode as light source, with a bandwidth of 40 nm centered at 830-nm wavelengths. The scan rate is equal to 28 000 A-scans/second, and the axial resolution is up to 5 μm. The coupled SLO image is acquired at the same wavelength by diverting a small amount of reflected light. The second SD-OCT/SLO was the Spectralis HRA+OCT (Heidelberg Engineering, Heidelberg, Germany): a superluminescent diode of 75-nm bandwidth centered at 870 nm is the light source for the OCT. The retina is scanned at 40 000 A-scans/second, and the axial resolution is 7 μm. The OCT image is coupled with the following SLO modes: infrared (IR), red free, autofluorescence, fluorescein angiography, and indocyanine green angiography. The instrument uses 3 additional laser light sources to achieve these imaging methods. The wavelengths of these lasers are 488 nm (red free, autofluorescence, fluorescein angiography), 790 nm (indocyanine green angiography), and 820 nm (IR). The instrument is provided with retinal tracking technology, which enables real-time, simultaneous imaging while tracking and correcting eye movement. In addition, the instrument uses image-averaging software to obtain multiple images from the same exact location. These images are combined, thus obtaining a significant reduction of speckle noise.
The same acquisition protocol was used with both instruments. Initially the linear OCT cursor was swept manually throughout the entire posterior pole in both vertical and horizontal orientation. The SLO image and the OCT were focused on the fovea and the retina surface. Scans also were acquired systematically in the optic nerve area and along the arcades. The focus of the instrument then was shifted slightly toward plus values to highlight the inner retina, the vitreoretinal interface, and the posterior vitreous architecture within 1 mm from the retina itself. If the vitreous cortex was not detected, the operator proceeded with a careful analysis of the vitreous cavity as follows: using the IR SLO image on the optic nerve as a landmark, the operator progressively focused anterior to it. The IR SLO image was used for the initial detection of the Weiss ring ( Figure 1 ) that, when seen, was centered and sharply focused. The cursor of the OCT scanner was swept in the same area. Fine adjustments of the distance of the camera to the head of the patient often were needed to detect the hyperreflective structure of Weiss ring, hyaloid, and cortical vitreous. Final scans were acquired beyond the arcades, covering 360 degrees of the mid periphery of the retina.
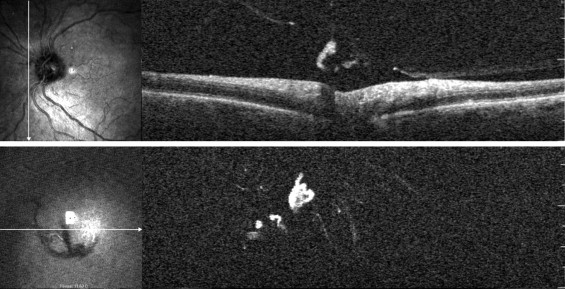
The following anatomic features, as detected by the OCT, were evaluated for each eye: a) adhesion of the hyaloid to the retina (specifying if completely attached or detached and, if incompletely detached, the location of the adhesion and the appearance of the vitreoretinal interface); b) in the macular area, the foveal profile was analyzed and its eventual relationship with the hyaloid (adhesion vs traction) and the visualization of precortical vitreous pocket were noted; c) at the level of the optic nerve, the detection of the cortical lacunae, and the eventual presence of the Bergmeister papilla were noted; d) along the vascular arcades, the presence of focal vitreoretinal adhesions and any focal defect in the retinal nerve fibers layers parallel to the vessels were recorded; e) in the periphery, sites of vitreoretinal adhesions or traction were studied carefully.
A complete PVD was diagnosed using the SD-OCT when the Weiss ring or hyaloid condensation was visible clearly on the scans, or when this was not possible, the diagnosis was made based on the total absence of cortex reflectivity throughout the entire posterior pole.
In a subset of patients (Patients 1–15), ophthalmic ultrasound also was performed. These patients were selected consecutively to evaluate PVD with ultrasound versus SD-OCT. Ultrasound was performed by a masked operator (I.K., certified for the Study to Evaluate Plasmin for the Creation of a Posterior Vitreous Detachment, Bausch & Lomb, Inc; ClinicalTrials.gov identifier NCT00348439 ). None of the patients of our study were enrolled in the above-mentioned clinical trial; however, we followed the standardized ultrasound method to scan our patients using the Innovative Imaging Inc (I 3 ) ABD diagnostic ophthalmic ultrasound machine (Sacramento, California, USA). This instrument uses 25 frames per second true real-time imaging with a 10-MHz sealed probe. With a sector scan angle of 52 degrees and an image depth of 45 mm, it optimally reaches 0.15-mm axial and 0.2-mm lateral resolution. At least 11 scans were performed in every eye. Longitudinal, reverse longitudinal scans through the macula and optic disc and transverse scans through the macula were acquired. In the reverse longitudinal scan, the probe is placed at the temporal limbus with the subject fixating nasally. The sound beam sweeps perpendicular to the limbus, along a horizontal meridian. The macula is displayed below the optic nerve void; the nasal posterior fundus is displayed above the optic nerve void. Transverse and longitudinal scans near the equator also were acquired at 12, 3, 6, and 9 o’clock. Additional scans were carried out in case of suspicious PVD in areas of interest. Apart from static images, dynamic examination with video recording also was performed. The primary goal in recording video loops was to capture kinetic properties (movement) of the vitreous body. Up to 10 seconds of imaging were recorded, and video acquisition was repeated as necessary.
The operator analyzed images for the presence of a PVD as well as the presence of vitreous opacities, strands, and membranes. A PVD was diagnosed by appearance, location, extent, and characteristic undulating after-movement (motion of the membrane observed on the screen after cessation of eye movement). The presence of a Weiss ring also helped to identify a complete PVD. The Weiss ring produces 1 or 2 distinct, point-like echoes typically near the optic disc. All patients had topical anesthetic drops administered before the examination.
The clinical, OCT, and ultrasound findings were compared. Vitreous status was categorized independently by each operator into 1 of 3 categories: complete detachment, partial detachment, or physiologic vitreoretinal adhesion. In case of discrepancy among the operators, 2 additional masked operators analyzed either the OCT (S.F.O.) or the ultrasound (W.R.F.) scans, and a consensus was obtained. The vitreous status was considered for statistical analysis. The proportion of complete, partial PVDs or absence of PVD diagnosed by clinical examination was compared with the corresponding proportion diagnosed by OCT using a κ statistic and generalized estimating equations (GEEs), which properly compare the correlated proportions and correct for intereye correlation in the same patient. In addition, we separately compared the clinical diagnosis of PVD with the OCT detection of Weiss ring using the GEE. In the 30 patients who underwent ultrasound, the proportion of PVD diagnosed by ultrasound findings was compared with the proportion of PVD diagnosed by OCT using the κ statistic and GEE. All analysis were conducted using SAS software version 9.2 (SAS, Inc, Cary, North Carolina, USA).
Results
Scans were acquired over a period of 9 months, from January through September 2008. A total of 202 eyes from 113 patients were included in the study. Fifty-eight patients were female, and 55 patients were male. The mean age was 59 years (standard deviation ± 17 years; range, 21 to 86 years).
Details of clinical and OCT examinations regarding vitreous status are reported in Table 1 . There was no significant difference in the clinical diagnosis and OCT detection of complete PVD ( P = .683), and high agreement was found with the κ statistic (0.82). No statistically significant difference was also found between clinical diagnosis of complete PVD and Weiss ring detection by OCT ( P = .5127). In 88 of the 93 eyes that were diagnosed as complete PVD at the OCT, the Weiss ring was visible clearly on the scans ( Figure 1 ). In the remaining 5 cases, no cortex was detected as being attached to the posterior pole in any of the scans, and in 3 cases, the additional presence of hyaloid remnants on the foveal clivus was detected ( Figure 2 ). None of these patients underwent ultrasound scanning, but the complete PVD was seen at the biomicroscopy examination. In 10 cases of clinically diagnosed complete PVD, the hyaloid and vitreous cortex were detected clearly as still attached to part of the retinal surface, and floaters were seen in the vitreous body. Overall, a partial PVD was detected more frequently by the OCT than by biomicroscopy examination (45 of 202 eyes vs 7 of 202 eyes, respectively; P < .0001; κ, 0.22), and a significant difference also was noted in the absence of PVD (100 with clinical evaluation vs 64 eyes with OCT of a total of 202 eyes examined, respectively; P < .0001, GEEs; κ, 0.58).
Vitreous Status | SD-OCT Findings | Clinical Findings | P Value a | κ Statistic |
---|---|---|---|---|
Complete PVD | 93/202 | 95/202 | .683 | 0.82 |
Partial PVD | 45/202 | 7/202 | < .0001 | 0.22 |
Normal vitreous | 64/202 | 100/202 | < .0001 | 0.58 |
Ultrasound examination was performed in a consecutive series of patients. A total of 30 eyes of 15 patients were scanned, and the PVD detection rate was compared with the OCT results ( Table 2 ). There was no significant difference between the ultrasound and the OCT findings for both complete PVD and incomplete PVD, and there was an almost perfect agreement when the κ statistic was performed in all the PVD stages (0.93 for complete PVD, 0.91 for partial PVD, 0.81 for normal vitreoretinal adhesion, respectively; P = .317; Figure 3 ).
Vitreous Status | SD-OCT Findings | Ultrasound Findings | P Value a | κ Statistic |
---|---|---|---|---|
Complete PVD | 16/30 | 15/30 | .3173 | 0.9333 |
Partial PVD | 7/30 | 8/30 | .3173 | 0.9112 |
Normal vitreous | 7/30 | 7/30 | 1.00 | 0.8137 |
Table 3 reports detailed anatomic findings detected by SD-OCT/SLO. OCT was able to detect in most of the eyes fine details of hyaloid, such as focal disruptions, intrinsic tension status, and tractional forces exerted on the retinal surface, which may occur in the fovea, but also in the optic nerve and arcades ( Figures 4, 5, and 6 ). In eyes with complete PVD, paravascular rarefaction and lamellar retinal tears (retinal pits) were noted in 10 cases (11%; Figure 5 ). In 2 of these eyes, a small cystic space localized in the outer retinal layers, on the temporal edge of the optic nerve, also was detected. A localized inner retinal schisis nasally to the optic nerve was noted in 2 cases of incomplete PVD with persisting attachment to the disc only ( Figure 6 ).
SD-OCT Findings | Complete PVD (n = 93) | Partial PVD (n = 45) | No PVD (n = 64) | Total (n = 202) |
---|---|---|---|---|
Posterior precortical vitreous pocket | 0 (0%) | 27 (60%) | 58 (91%) | 85 (42%) |
Vitreal cortex | 74 (79%) | 45 (100%) | 62 (97%) | 181 (89%) |
Floaters | 20 (21%) | 1 (2%) | 5 (8%) | 26 (13%) |
Bergmeister papillae | 10 (11%) | 5 (11%) | 6 (9%) | 31 (15%) |
Paravascular lamellar holes | 10 (11%) | 0 (0%) | 0 (0%) | 10 (5%) |
Macular abnormalities a | ||||
VMT | 0 (0%) | 6 (13%) | 0 (0%) | 6 (3%) |
ERM | 7 (7%) | 6 (13%) | 0 (0%) | 13 (6%) |
Dehiscence | 0 (0%) | 3 (7%) | 0 (0%) | 3 (1%) |
Hyaloid remnant | 13 (14%) | 2 (5%) | 0 (0%) | 15 (7%) |
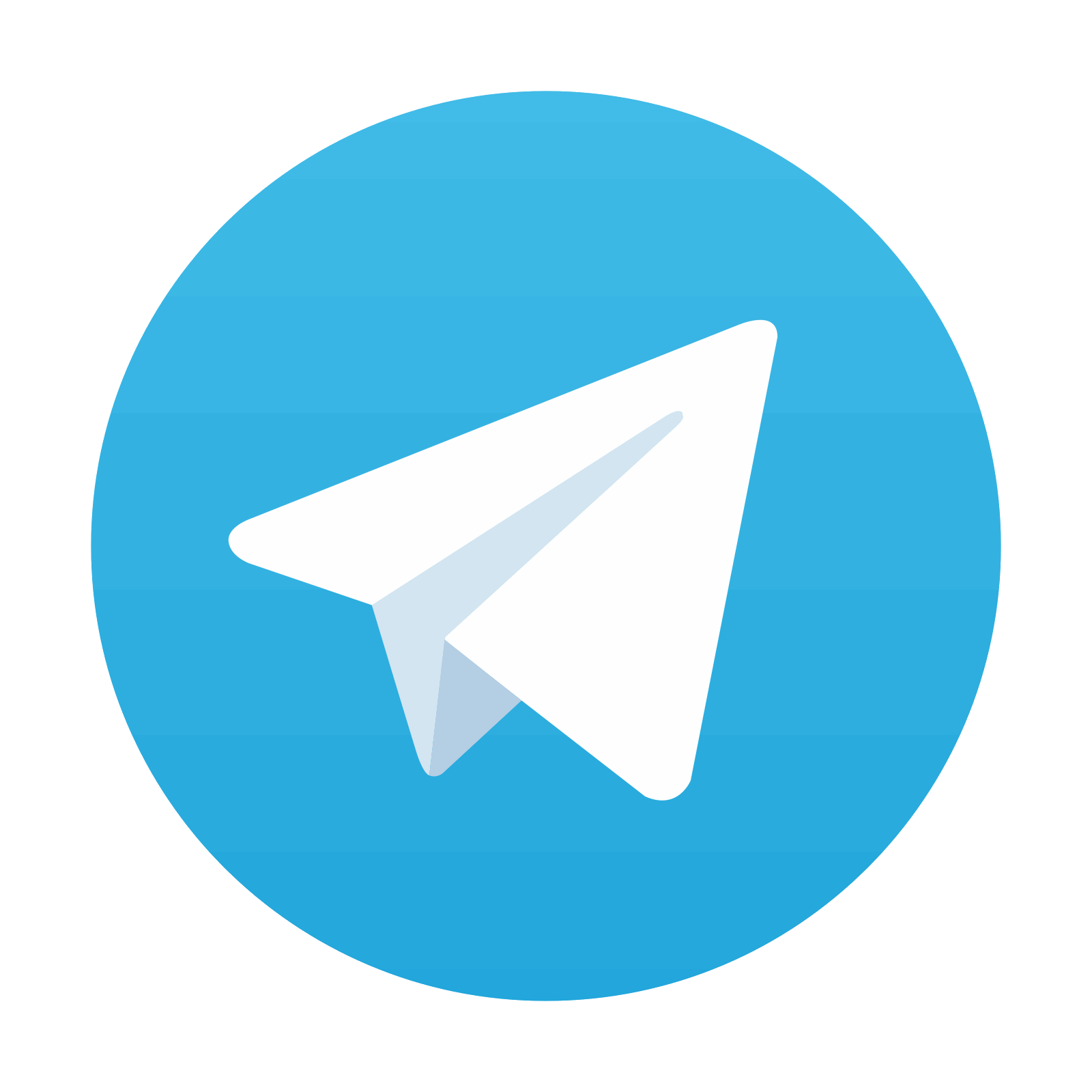
Stay updated, free articles. Join our Telegram channel

Full access? Get Clinical Tree
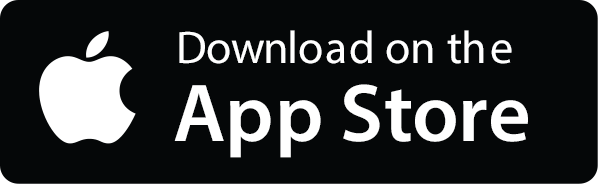
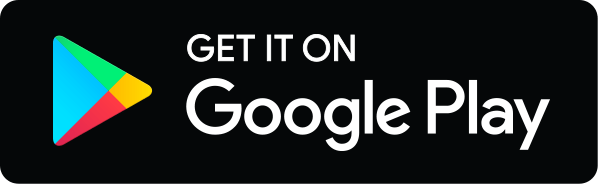
