Nasal Pathology in Snoring and Obstructive Sleep Apnea
Summary
Both snoring and obstructive sleep apnea (OSA) are conditions associated with multilevel partial or complete temporary upper airway obstruction. Pathophysiologic mechanisms that explain the role of nasal pathology in sleep-disordered breathing (SDB) include the unstable oral airway, the Starling resistor model, the role of nitric oxide (NO), and the nasal ventilatory reflex. Although some clinical case control studies have shown that nasal obstruction is associated with snoring and mild SDB, there is no evidence of a direct correlation between the degree of nasal obstruction and the severity of SDB. However, randomized, controlled studies have shown that in patients with allergic rhinitis or nonallergic rhinitis and sleep disturbance, nasal steroids improve the subjective quality of sleep and may be useful for patients with mild OSA. However, they are not by themselves an adequate treatment for most patients with OSA. Similarly, although nasal surgery may improve quality of life and snoring in a subgroup of patients with mild SDB and septal deviation, it is not considered effective OSA treatment. In the subgroup of patients who cannot tolerate continuous positive airway pressure (CPAP) or require very high pressures, surgical or medical treatment of obstructive nasal passages can potentially improve CPAP compliance.
Introduction
From the association of nasal polyps and restless sleep in Hippocrates’ “De Morbis Popularibis” to the commonly observed sleeping difficulty during episodes of virally induced nasal congestion, a link between nasal breathing and sleep seems intuitive. However, and despite the interest generated in this area, as demonstrated by the number of articles published on the subject, the literature is far from conclusive. Nevertheless, our understanding of nasal and sleep physiology, particularly of the importance of nasal and oral breathing as related to sleep apnea and total airway resistance, has significantly progressed over the past decade, while recent double-blind, randomized, controlled trials evaluating treatment outcomes objectively and the use of quality of life outcome instruments have added further to our knowledge.
Sleep apnea is usually defined as cessation of airflow into the lungs that lasts for more than 10 seconds. The severity of sleep apnea is primarily defined by the apneahypopnea index (AHI): the number of episodes of apnea (cessation of airflow for > 10 s) and hypopnea (variable definitions, usually a significant decrease in airflow) per hour. Sleep-related breathing disorder (SRBD), or sleep-disordered breathing, is essentially a disease continuum ranging from simple or primary snoring to OSA.1 Upper airway resistance syndrome (UARS) occupies an intermediate position in this disease continuum. The prevalence of primary/simple snoring in middle-aged men ranges from 25 to 50%,2 whereas significant SDB affects up to 8% of men and 3% of women3 (as shown in the recent prospective Sleep Heart population study in 6441 subjects). Dynamic magnetic resonance imaging (MRI), acoustic analysis, sedation endoscopy, and pharyngeal luminal pressure recordings have established the fact that both snoring and OSA are multilevel phenomena.4 However, the first port of entry of inspired air under normal conditions is the nose, with nasal pathology having a significant impact on airflow.5
Pathophysiology of Nasal Obstruction and Breathing During Sleep
The nose accounts for > 50% of the total resistance of the upper airway, and nasal breathing serves important physiologic functions, including humidification, heating, and filtration.6 Physiologic mechanisms that explain the relationship between nasal airflow and breathing during sleep include the Starling resistor model, the unstable oral airway proposition, the nasal ventilatory reflex, and the role of NO ( Table 34.1 ).
The Starling resistor model 7,8 regards the upper airway as a hollow tube, with a partial obstruction at the inlet, corresponding to the nose, and a collapsible segment downstream, corresponding to the oropharynx ( Fig. 34.1 ). This model predicts that a further obstruction upstream (nose) will generate a suction force (negative intraluminal pressure) downstream (oropharynx) that could result, in predisposed individuals, in oropharyngeal collapse. This effect is exacerbated at the supine position, when nasal resistance tends to increase both actively as the result of postural reflex mechanisms and passively due to the effects of hydrostatic pressure on nasal venous circulation.9
However, this model fails to take into account a major feature of normal breathing: oral breathing bypass, that is, the option of mouth breathing when nasal resistance exceeds a certain level. This switch (from nasal breathing to oral breathing) is physiologically disadvantageous and results in unstable oral breathing. During sleep, upper airway resistance is lower in subjects breathing through the nose than through the mouth; during the awake state, the resistance is equal.10 Hence, the fraction of oral breathing normally decreases even further during sleep from 7.6 to 4.3%.11 When the nasal airway is almost completely obstructed, the switch from nose to mouth breathing occurs, but at a high physiologic cost: mouth breathing is associated with up to 2.5 times higher total resistance10 and with narrowing of the pharyngeal lumen, a decrease in the retroglossal diameter as a result of further posterior retraction of the tongue, and an increase in the oscillation and vibration of the soft palate and redundant pharyngeal tissue,12 all factors of unstable oral breathing potentially leading to SRBD. These pathophysiologic changes can be visualized in Video 47 (Sedated, Snoring, Supine Patient, Pure Palatal ), a sleep nasendoscopy of a patient with nasal obstruction and associated oral breathing. It is clear how unstable oral breathing leads to posterior tongue retraction and oropharyngeal collapse. In Videos 48 (Tongue Base Snorer ) and 49 (Palatal Snoring and Effect of Jaw Lift ) of a patient with OSA with nasal obstruction, posterior tongue retraction is associated with palatal vibration and snoring.

A third factor is the nasal ventilatory reflex. Experimental application of local anesthetics to the nasal mucosa of healthy volunteers leads to a significant increase in both central and obstructive apneic episodes of the same magnitude as those reported with complete nasal obstruction.13 Similar results from other experiments14 confirmed that the activation of nasal receptors during nasal breathing has a direct positive effect on spontaneous ventilation, resulting in higher resting breathing frequency and minute ventilation. Bypassing the nasal airway can lead to reduced nasal receptor activation, deactivation of the nasal ventilatory reflex, and reduced spontaneous ventilation, which, in a subset of susceptible individuals, can be associated with exacerbating or unmasking previously subclinical sleep apnea,15 as well as increasing the duration of apneic episodes.16
Nitric oxide (NO) is thought to play a role in maintaining upper airway patency by acting as an aerotransmitter between the nose, pharyngeal musculature, and lungs.17 NO is produced in significant amounts in the nose and sinuses and has been shown to be (and used clinically as) a potent lung vasodilator, reducing perfusion-ventilation mismatch and improving overall blood oxygenation.18 As the total amount of inspired NO varies according to nasal flow,19 it seems logical that a decrease in nasal ventilation would result in reduced NO delivery to the lungs and a reduced oxygen exchange capability. However, NO also has a role in the maintenance of muscle tone and the regulation of neuromuscular pathways in the pharyngeal musculature, as well as spontaneous breathing and sleep regulation, so, overall, its role in the nasal regulation of OSA, although probably quite significant, is still not completely understood.20
Clinical Correlation Between Nasal Pathology and Sleep-Disordered Breathing
Several investigators have assessed the effect of experimental or externally induced reduction of nasal patency and flow on breathing and blood oxygen saturation during sleep. In one study, intranasal application of petroleum jelly gauzes in healthy volunteers induced obstructive apneic episodes and resulted in significant increases in AHI, producing frank OSA in one subject.21 In another study, artificially induced complete or partial nasal obstruction resulted in significant alteration of sleep pattern, with increased micro-arousals and total number of episodes of apnea.22 In a clinical setting, the application of nasal packs during the management of epistaxis results in significantly worse quality of sleep with multiple episodes of apnea and desaturation episodes.23 Hence, it is probably safe to conclude that externally induced partial or complete nasal obstruction results in increased episodes of sleep apnea.
Non-externally induced nasal obstruction experienced by patients could be the result of structural abnormalities (e.g., deviated nasal septum [ Fig. 34.2 ], enlarged turbinates, and nasal valve collapse), inflammatory mucosal disease (rhinitis and chronic rhinosinusitis with or without nasal polyps [ Fig. 34.3 ]), tumors, or, rarely, neuromuscular problems. Numerous observational studies have demonstrated that nasal congestion is associated with snoring and daytime sleepiness. The Wisconsin Sleep Study, a prospective population study of 1032 healthy volunteers, showed a three-fold increase in the incidence of snoring and daytime sleepiness in volunteers with self-reported nocturnal nasal congestion,24 and a study of 37 patients assessed with polysomnography and rhinomanometry showed a correlation between nasal resistance in the supine position and habitual snoring.25 On the other hand, Lofaso et al26 in a prospective study showed that nasal obstruction as documented by posterior rhinomanometry in the awake state is an independent risk factor for OSA, albeit accounting for only 2.3% of the total AHI variance. These results have been contradicted by some other studies, including that by Miljeteig et al27 in 1992. The researchers divided 683 patients referred for sleep polysomnography into three groups on the basis of their nasal resistance. They subsequently failed to find any differences in apnea and snoring indices among the three groups. However, the study assessed awake nasal resistance in the erect position, whereas, as the authors noted themselves, it seems more likely that sleep disturbances correlate with supine nasal obstruction during sleep. The same authors performed a few years later a similar study measuring supine nasal resistance and again failed to find any correlation with sleep disturbance indices. However, their study did not include patients with significant nasal obstruction, whereas all the measurements were done in nonphysiologic conditions, with the subjects having their mouths taped throughout the night to prevent oral breathing.28


Allergic rhinitis affects 9 to 42% of the population.29 The mechanism by which allergic rhinitis causes poor sleep quality and daytime fatigue is not entirely clear, but it is thought that multiple factors are involved. In addition to nasal obstruction, various inflammatory mediators, such as interferon (IFN)-γ, tumor necrosis factor (TNF)-α, and interleukin (IL)-1b, IL-4, and IL-10,30 postural changes, and some therapeutic agents, such as antihistamines, may also have a direct effect on sleep regulation. There seems to be a direct association between nasal resistance in patients with allergic rhinitis and SRBD severity31 or at least between subjective quality of sleep and daytime sleepiness and allergic rhinitis.32 A recent study found that both allergic rhinitis and nonallergic rhinitis are associated with impaired sleep quality, with up to 83% of patients with the latter complaining of poor sleep quality.33
In an overview, McNicholas34 tried to synthesize these apparently conflicting results, pointing out that reversible nasal obstruction may be more closely associated with SRBD than permanent nasal obstruction, and noting that studies assessing patients with temporal obstruction (including iatrogenic and allergic rhinitis) tended to show a more consistent association with SDB than studies assessing patients with structural abnormalities such as deviated nasal septum.
Note that nasal obstruction, especially reversible, either artificial or disease-induced, is associated with snoring and mild SDB. However, there is no linear correlation between the degree of nasal obstruction and the severity of SDB.
Note
Nasal obstruction does not appear to be the main contributing factor in the majority of patients with moderate to severe OSA.
Implications for Management
Problems with Research
The link between nasal pathology and SDB has led to attempts to improve sleep quality by focusing on the treatment of nasal obstruction either medically (nasal steroids, antihistamines, decongestants, leukotriene antagonists, and nasal dilators) or surgically. Although several clinical trials have assessed the effectiveness of the above, they lacked uniformity, and only a handful assessed objective outcome measures. Many of the studies were nonrandomized, lacked a control group, and had small numbers of patients and short follow-up periods.
Another problem in many of the studies assessing the efficacy of these treatments was the lack of clear inclusion criteria. In many studies, patients with self-reported nasal obstruction were included, whereas in others, only those with physician-assessed nasal obstruction took part. On the other hand, the use of objective criteria for nasal obstruction (e.g., rhinomanometry or acoustic rhinometry) must take into account the unreliable correlation between such objective measures and patient-reported nasal obstruction. Finally, the results of some studies frequently assessed the effect of treating the underlying disease (e.g., allergic rhinitis) rather than the symptom of nasal obstruction.
Medical Treatment
The management of chronic rhinitis includes topical intranasal steroids, sympathomimetic decongestants, and leukotriene antagonists.
Decongestants
Three randomized, controlled trials were performed on the use of nasal decongestants (oxymetazoline) in OSA. In the study by Kerr et al,35 the decongestant was combined with an internal nasal valve dilator; in the one by Mclean et al,36 with an external dilator (Breath Right, CNS Inc.; Bloomington, Minnesota); while the more recent study by Clarenbach et al37 assessed the effect of decongestant alone. The characteristics and results of the studies are presented in Table 34.2. Two of the three studies showed no change in AHI and sleep architecture, whereas the study by McLean et al showed a modest decrease in AHI of limited clinical significance with no improvement in sleep quality or daytime somnolence. The study by Clarenbach et al showed no improvement in either subjective or objective indices of AHI, although it did show that at the time of maximal effect of oxymetazoline, there was a significant reduction in AHI. Finally, the study by Kerr et al demonstrated only a subjective improvement in sleep quality.
Taken together, these results show that nasal decongestants with or without nasal dilators are not effective in the management of OSA (no improvement in sleepiness or AHI). Hence, long-term use of nasal decongestants is contraindicated and is not part of the management of OSA.
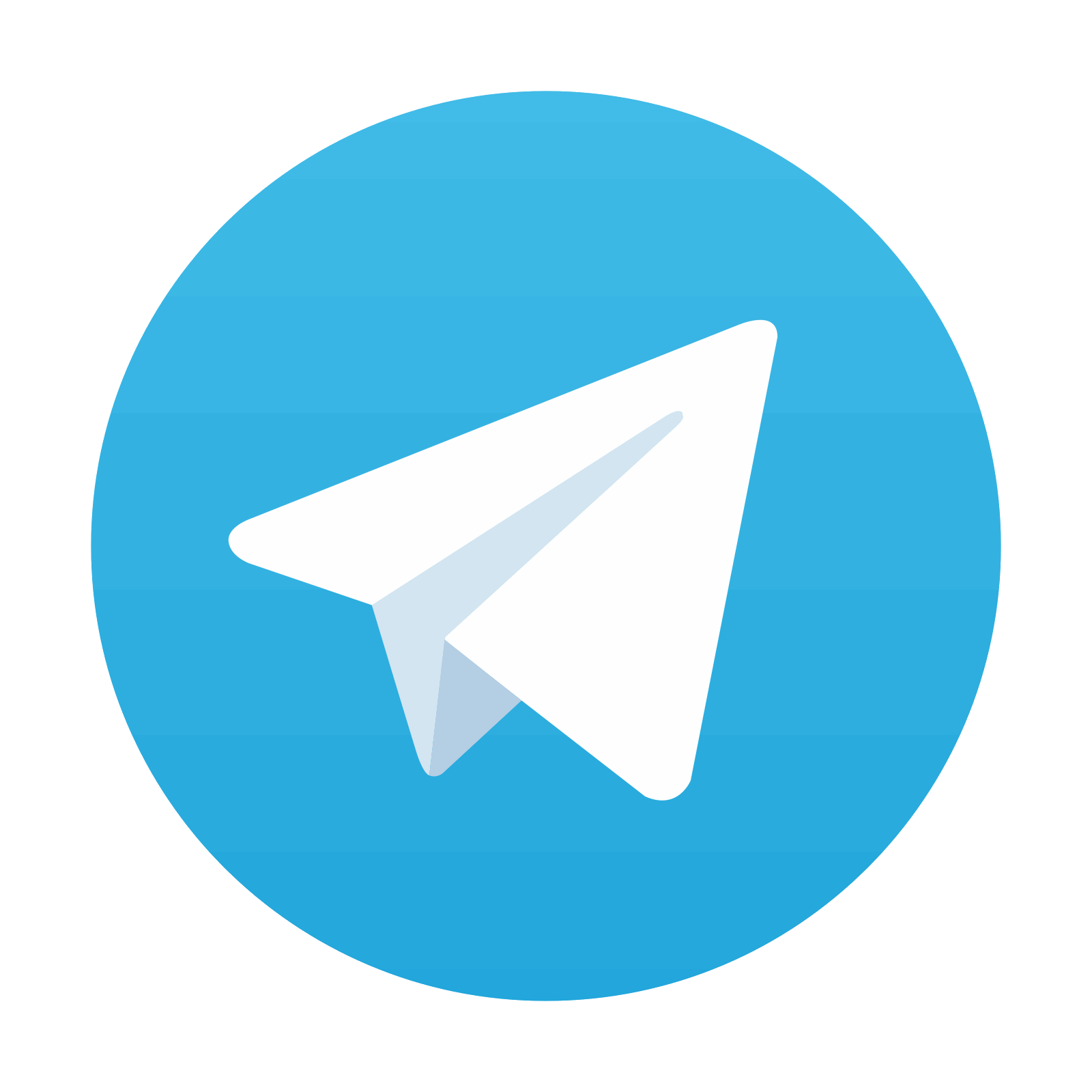
Stay updated, free articles. Join our Telegram channel

Full access? Get Clinical Tree
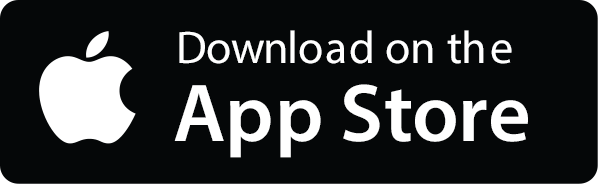
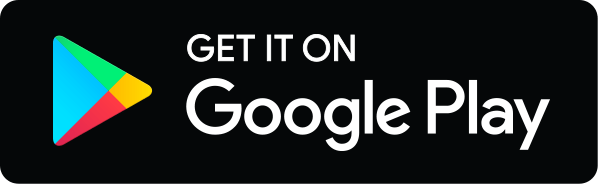
