Purpose
To dynamically evaluate contact lens movement and ocular surface shape using ultrahigh-resolution and ultralong-scan-depth optical coherence tomography (OCT).
Design
Clinical research study of a laboratory technique.
Methods
Four different types of soft contact lenses were tested on the left eye of 10 subjects (6 male and 4 female). Lens edges at primary gaze and temporal and nasal gazes were imaged by ultrahigh-resolution OCT. Excursion lag was obtained as the distance between the lens edge at primary gaze and immediately after the eye was quickly turned either nasally or temporally. The inferior lens edges were imaged continuously to track vertical movements during blinking. Ultralong-scan-depth OCT provided quantifiable images of the ocular surface, and the contour was acquired using custom software.
Results
Excursion lag at the horizontal meridian was 366 ± 134 μm at temporal gaze and 320 ± 137 μm at nasal gaze ( P > .05). The lens uplift at the vertical meridian was 342 ± 155 μm after blinking. There were significant differences in horizontal lags and vertical movements among different lenses ( P < .05). Horizontal lags were correlated with radii of curvatures and sagittal heights at 6-mm and 14-mm horizontal meridian ( P < .05). The blink-induced lens uplift first lowered by 104 ± 8 μm, and then lifted 342 ± 155 μm after the blink.
Conclusions
Ultrahigh-resolution and ultralong-scan-depth OCT can assess micrometer-scale lens movements and ocular surface contours. Both lens design and ocular surface shape affected lens movements.
Dynamic evaluation of contact lenses on the eye reveals lens movements that are critical in maintaining ocular health and comfort. Lens movements are regarded as indicators of the tightness of lens fitting, and if too tight, the lens does not move very well. Proper lens movement facilitates tear exchange underneath the lens and the removal of debris and dead cells. In the modern contact lens practice, lens movement is routinely evaluated by slit-lamp biomicroscopy or quantified by a digital camera with video capture. There may be a subjective appraisal during the measurement of the lens movement, and the assessment may not be adequate because of the lack of cross-sectional landmarks and quantification of movement at the micrometer level. Without a static landmark, estimates of the movement by these methods may be noisy. Using a cross-sectional landmark to track micrometer-range movements of the lens may further enhance modeling of the interaction between lens and ocular surface.
Lens fitting characteristics are impacted by many factors, such as lens material and design, blink rate, corneal shape, and post-lens tear film. The diameter of soft contact lenses is about 14 mm, and the lenses cover the whole cornea along with the transition from the cornea to the sclera and some parts of the sclera. In the traditional lens fitting procedure, the central corneal curvature, approximately 3 mm from the apex, is used to select the appropriate base curve of the soft contact lens. Young found the central shape may not play an important role in determining overall lens fitting. This indicates that information regarding the central corneal shape may not be adequate for evaluating the lens fit. Our recent studies show that interaction between the lens edge and the ocular surface at the peripheral cornea plays an equally important or more important role for lens fitting.
Current videokeratoscopes are able to characterize the majority of the entire cornea. However, the limited usefulness of these instruments to predict soft lens fitting can be attributed to their inability to provide information about the peripheral ocular surface, especially the cornea-scleral junction. The impact of interaction around the periphery of the ocular surface on the lens movement remains unclear, mainly because of the lack of a suitable instrument for evaluating the overall ocular surface shape. The goal of this pilot study was to demonstrate the feasibility of using optical coherence tomography (OCT) to quantify the lens movement and overall shape of the ocular surface.
Subjects and Methods
Subjects had no evidence or history of binocular vision anomalies or any pathology that would normally contraindicate contact lens wear. During screening, slit-lamp biomicroscopy evaluation was performed to confirm the lens fitting with a centration of less than 1 mm. After a screening test, 10 subjects (6 male and 4 female; mean ± SD age, 31.2 ± 6.5 years) were recruited.
We imaged the overall ocular surface using a custom-built spectral-domain ultralong-scan-depth OCT instrument. A custom spectrometer with special design was developed to achieve a scan depth of 7.3 mm in air. An internal fixation target displayed on a miniature LCD monitor was provided. To ensure proper alignment during OCT scanning, X-Y cross aiming was applied. The scan width was set to 18 mm. In our recent study, the overall ocular surface of human eyes was imaged and processed with custom software to yield sagittal height, corneal curvature, and peripheral shape around the corneal-scleral junction. The accuracy and repeatability of measurement for ocular surface shape was tested and validated.
A custom-built spectral-domain ultrahigh-resolution OCT with ∼3-μm resolution was used to image each contact lens on the eyes. The ultrahigh-resolution OCT and its use in imaging the tear film and contact lenses in vivo were described in detail previously. In brief, a 3-module superluminescent diode light source (Broadlighter, T840-HP; Superlumdiodes Ltd, Moscow, Russia) was used with a center wavelength of 840 nm and a bandwidth of 100 nm.
Each subject was tested in a consulting room after 10 AM to avoid the edematous cornea and the alterations of the tear film induced by sleep. Using ultralong-scan-depth OCT, the overall ocular surface of the subjects’ left eyes were imaged at the horizontal and vertical meridians ( Figure 1 ) before lens fitting. Then 4 types of commercially available soft contact lenses ( Table 1 ) were randomly chosen and inserted into the left eye. Before ultrahigh-resolution OCT imaging, a 5-minute period for lens adaptation was allowed after every lens insertion. Subjects were asked to sit in front of the ultrahigh-resolution OCT instrument and look straight at the fixation target, which was placed in front of the eye for primary gaze. OCT scanning at the horizontal meridian was conducted to image the lens excursion lag, both nasally and temporally. Excursion lag was defined as the distance between the location of the lens edge while gazing forward and its location immediately after the eye is quickly turned either nasally or temporally. The lag occurs because inertia of the lens causes it to move more slowly than the eye. The lens edge was imaged ( Figure 1 ) at both nasal and temporal sides. When the subject looked at the fixation target placed at 45 degrees temporally (monitored with the slit-lamp angular scale), the lens edge at the nasal side was imaged. Similarly, when the subject looked at the nasally positioned target, the edge at the temporal side was imaged ( Figure 1 ).
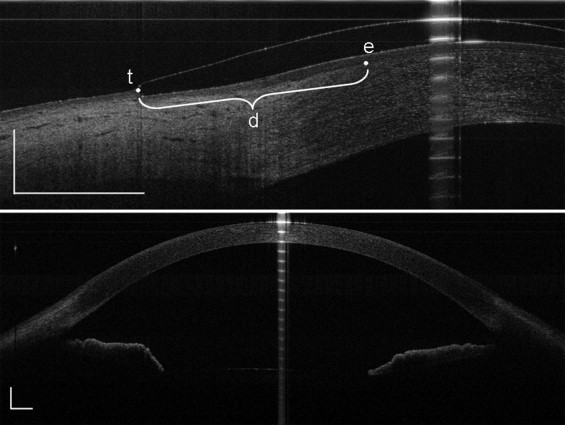
Acuvue Advance | Acuvue 2 | PureVision | O 2 OPTIX | |
---|---|---|---|---|
Manufacturer | Vistakon, Johnson & Johnson | Vistakon, Johnson & Johnson | Bausch & Lomb | Ciba Vision |
Diameter (mm) | 14.0 | 14.0 | 14.0 | 14.2 |
Base curvature (mm) | 8.7 | 8.7 | 8.6 | 8.6 |
Power (diopters) | −3.00 | −3.00 | −3.00 | −3.00 |
Material | Galyfilcon A (SiHi) | Etafilcon A | Balafilcon A (SiHi) | Lotrafilcon B (SiHi) |
Modulus (MPa) | 0.43 | 0.3 | 1.1 | 1.0 |
Edge shape | Angled | Angled | Rounded | Chisel |
Center thickness (mm) | 0.07 | 0.08 | 0.09 | 0.07 |
Water content (%) | 47.0 | 58.0 | 36.0 | 33.0 |
To visualize lens movement induced by blinking, the inferior lens edge was imaged by ultrahigh-resolution OCT at the vertical meridian for 2.7 seconds continuously while blinking, during which 128 frames were acquired. Subjects were asked to have several normal blinks before image acquisition, and then the images were acquired for 2 or 3 blinks. During OCT imaging, subjects were asked to fixate on the primary-gaze position during the open-eye period and immediately after every blink. The lower eyelid was pulled down a little so that the lower edge of the lens could be imaged.
Custom software was used to obtain the location of the contact lens edge on the eye. Two landmarks were chosen to determine the edge location. One was the end of the Bowman’s layer at the limbus and the other was the tip of the contact lens edge ( Figure 1 ). The lens excursion lag at the horizontal meridian was assessed as the linear distance between the ending of the Bowman’s layer and the tip of the lens edge at the nasal and temporal gazes compared to that at primary gaze. To display the movement, an OCT M-mode view was created to show the footprint of the lens edge during blinking ( Figure 2 ) . The M-mode view reconstruction was formed by summing the information of the lens edge in every frame of all 128 acquired images during 1 OCT data acquisition. In every single frame, the vertical cross-sectional image was taken at the same horizontal position. To process the OCT images obtained by ultralong-scan-depth OCT, we used custom software to define the radii of curvature and sagittal heights of 3-, 6-, 10-, and 14-mm zones on the ocular surface at the horizontal meridian and 3-, 6-, and 10-mm zones at the vertical meridian.
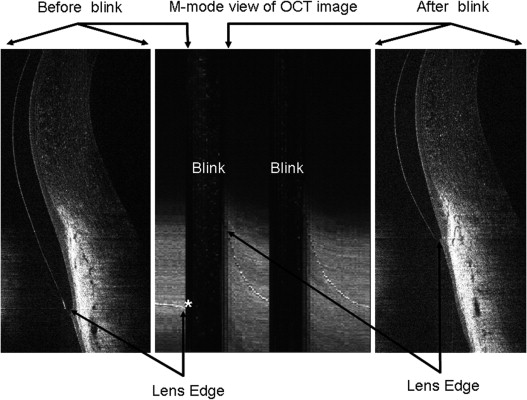
Data analysis was conducted using the Statistical Package for Social Sciences (SPSS version 17.0 for Windows XP; SPSS Inc, Chicago, Illinois, USA). All of the data were presented as means ± standard deviations. Independent t tests were applied to the comparison of lens excursion lags between temporal and nasal gazes. Analysis of variance (ANOVA) was used to determine if there were any differences between the different lenses in movements. Post hoc tests were used to compare the movements between any 2 lenses. Pearson correlation was used to test correlations between lens movements at different meridians and the relationship between lens movements and ocular surface parameters. P < .05 was considered significant.
Results
At the horizontal meridian the lenses were located in different positions at primary gaze compared to temporal or nasal gaze. The lens excursion lag was 366 ± 134 μm at temporal gaze and 320 ± 137 μm at nasal gaze ( Table 2 ). There were no differences between lens temporal and nasal gaze lags at the horizontal meridian (independent t test, P > .05; Table 2 ). There were significant differences in lens movements, including horizontal lags and vertical movements, among all of the contact lenses. The PureVision (Bausch & Lomb, Rochester, New York, USA) lens showed significantly larger excursion lag at the horizontal meridian compared to Acuvue 2 (Vistakon; Johnson & Johnson Vision Care, Inc, Jacksonville, Florida, USA) and O 2 OPTIX (Ciba Vision Corp., Duluth, Georgia, USA) lenses (post hoc, P < .05; Figure 3 ) . Blink-induced uplift of the PureVision lens was larger than for both the Acuvue Advance (Vistakon, Johnson & Johnson Vision Care) and Acuvue 2 lenses (post hoc, P < .05; Figure 3 ). Lens temporal gaze lag was correlated with the nasal gaze lag ( P < .05; Figure 4 ) , but there was no correlation between horizontal lags and blink-induced uplift at the vertical meridian ( P > .05; Figure 5 ) .
Lens | Horizontal Lag a | Vertical Movement a | ||
---|---|---|---|---|
Temporal Gaze Lag b (μm) | Nasal Gaze Lag b (μm) | Blink-Induced Uplift (μm) | Blink-Induced Lowering (μm) | |
Acuvue Advance | 372 ± 103 | 367 ± 194 | 239 ± 122 | 107 ± 17 |
Acuvue 2 | 292 ± 83 | 266 ± 88 | 253 ± 122 | 107 ± 35 |
PureVision | 478 ± 189 | 376 ± 136 | 394 ± 158 | 103 ± 17 |
O 2 OPTIX | 322 ± 53 | 271 ± 79 | 322 ± 112 | 101 ± 25 |
Mean ± SD (μm) | 366 ± 134 | 320 ± 137 | 342 ± 155 | 104 ± 8 |
a Mean ± SD values, n = 10 eyes.
b There were no differences between lens temporal and nasal gaze lag at the horizontal meridian (independent t test, P > .05).
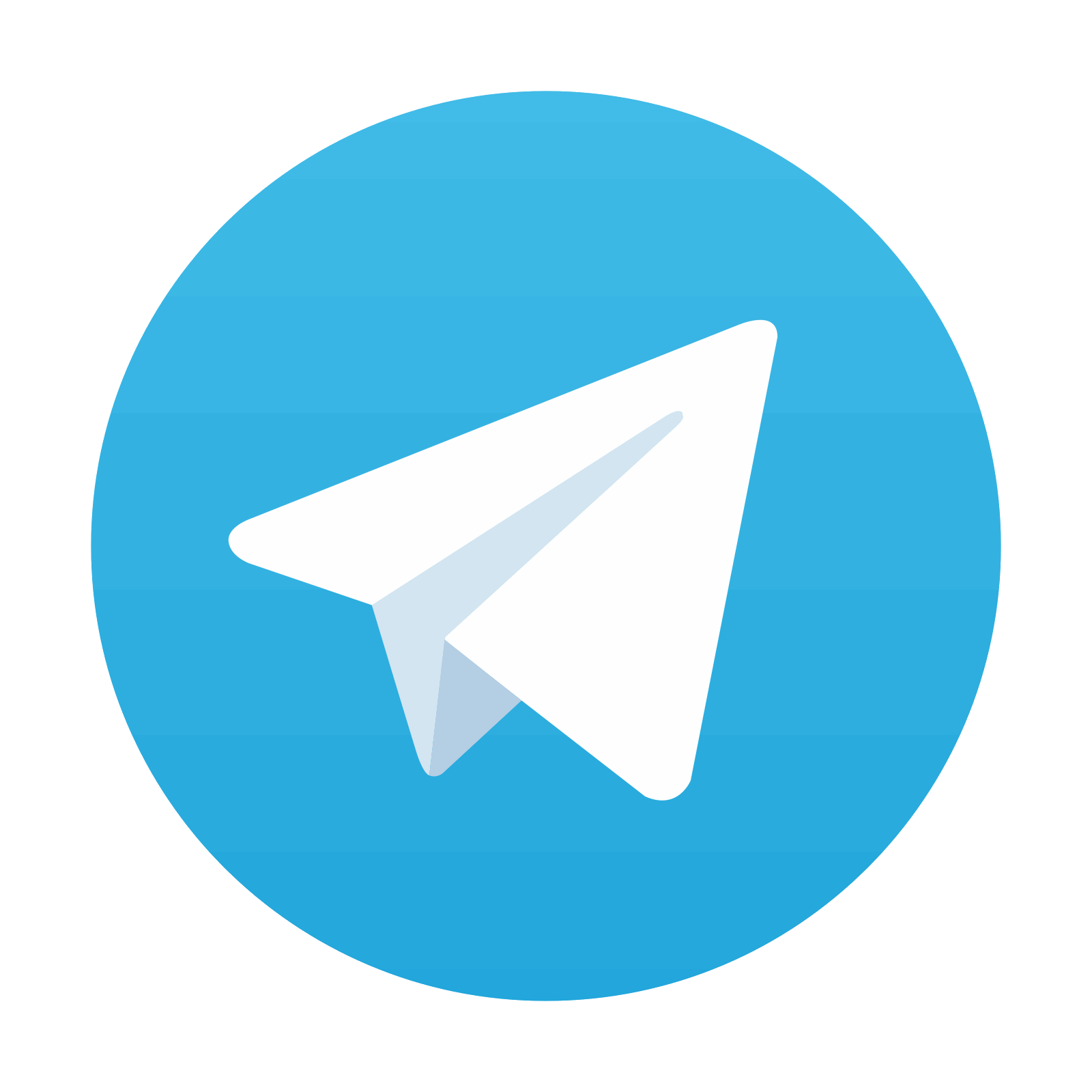
Stay updated, free articles. Join our Telegram channel

Full access? Get Clinical Tree
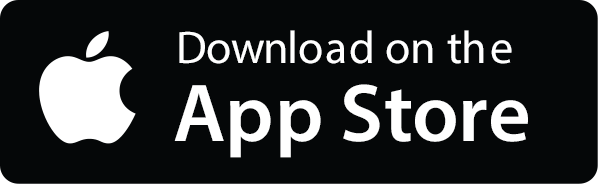
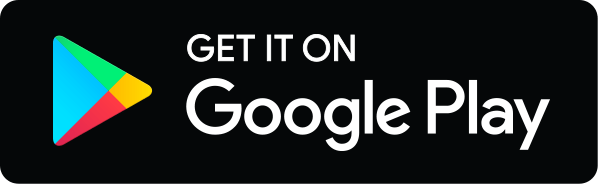
